Last updated: August 27, 2021
Article
National Park Service Air Quality Analysis Methods 2020
December 2020
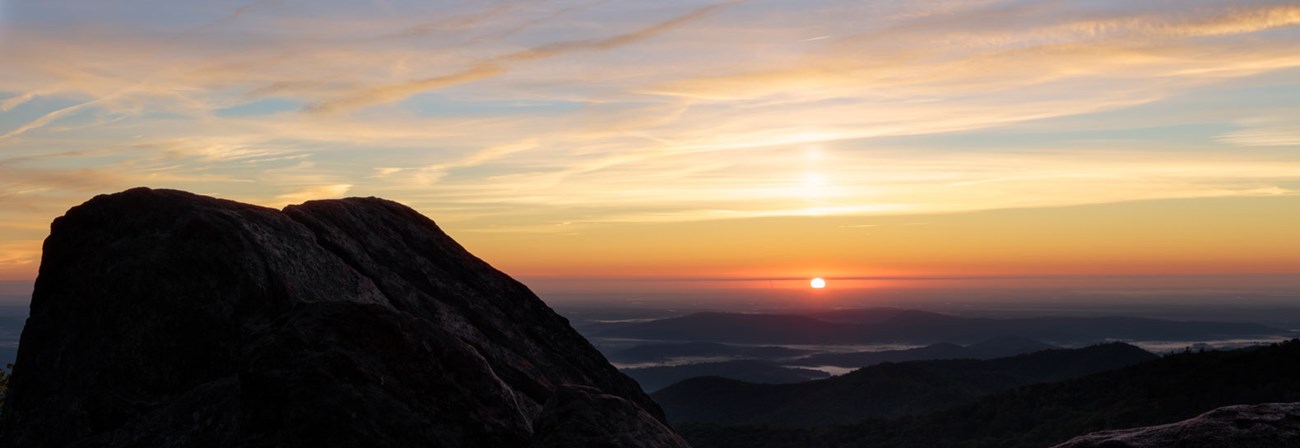
1. Introduction
The National Park Service Air Resources Division (NPS-ARD) provides specific air quality information for over 400 NPS areas, including the status and trends of key air quality indicators. This webpage documents the consistent, service-wide analysis methods we use to evaluate air quality for NPS areas. Air quality indicators considered include visibility, ozone, nitrogen & sulfur deposition, particulate matter, and mercury. This webpage details methods used to:
- Identify current air quality
- Assess air quality conditions
- Calculate 10-year and long-term trends
- Present the
- composition of particulate matter affecting visibility
- distribution of clearest, haziest, and most-impaired visibility days
- number and distribution of exceedance days for ozone and particulate matter
- composition of nitrogen and sulfur deposition
- annual precipitation
Air quality analysis methods described here support the park-specific NPS air quality conditions & trends and national air quality condition & trends maps webpages. Visit these webpages to learn more about air quality in your favorite NPS area. Visit the NPS Air website to learn more about the overall importance of clean air for national parks.
2. Identifying Current Air Quality
The NPS participates in several national, multiagency air quality monitoring networks. These networks focus on ozone, visibility, particulate matter, and atmospheric deposition of nitrogen, sulfur, and mercury. We use data from these networks to evaluate current air quality values for each end-year going back to 2009. These air quality values provide a quick snapshot of the air quality in parks.
We identify current air quality values for NPS areas using either an estimation method or by directly reporting monitoring data from in-park or representative monitoring sites. Because air quality monitoring sites are not evenly distributed across the US, we use spatial interpolation to estimate air quality measures in non-monitored locations or locations with multiple monitors. NPS-ARD uses Inverse Distance Weighting (IDW) to estimate ozone, visibility, and atmospheric deposition for NPS units within the contiguous US.
Uncertainties in air quality estimates vary by location, pollutant, and type of pollution source. More detailed assessments (e.g. emissions testing and more refined modeling) may be needed to understand specific risk values within a park. Further, monitoring inputs into spatial interpolation can change between assessments making comparison across end-years inaccurate. For this reason, air quality estimates should not be used to examine trends from one end-year to another. Trend assessments from in-park or nearby representative monitoring sites are a more accurate representation of air quality changes over time.
Where monitoring data are too sparse for spatial estimation, or local monitors are preferred, monitor-specific data are directly reported from in-park or representative monitoring sites. This is the case for the particulate matter, some visibility data, and all indicators monitored in Alaska, Hawaii, Puerto Rico, and the Virgin Islands.
▸ 2.1. Ozone Values
Ozone is monitored across the US through air quality monitoring networks operated by the NPS, Environmental Protection Agency (EPA), states, and others. Aggregated hourly ozone concentration data are acquired from the EPA Air Quality System (AQS) database. Note that prior to 2012, monitoring data for selected sites were obtained from the EPA Clean Air Status and Trends Network (CASTNET) database. Hourly ozone concentration data are used to calculate annual concentration statistics detailed below.
Ground-level ozone values are derived using two statistics:
- Human health: 4th-highest daily maximum 8-hour average ozone concentrations
- The daily maximum 8-hour average ozone concentration for a given day is the highest of the 17 consecutive 8-hour averages, beginning with the 8-hour period spanning from 7:00 a.m. to 3:00 p.m. and ending with the 8-hour period from 11:00 p.m. to 7:00 a.m. the following day (i.e., the 8-hour averages for 7:00 a.m. to 11:00 p.m.). The 4th-highest daily maximum 8-hour average ozone concentration within in a calendar year is reported in parts per billion (ppb). See Appendix C for more information.
- Vegetation health: 3-month maximum 12-hour W126 index
- The W126 index preferentially weighs the higher ozone concentrations most likely to affect plants and sums all the weighted concentrations during daylight hours (8 a.m. to 8 p.m.). The highest 3-month period that occurs during the growing season (March–September) is reported in parts per million-hours (ppm-hrs). See Appendix C for W126 index calculation methods.
Each of these statistics is averaged over a 5-year period at all monitoring sites with at least 3-years of complete annual data. Annual completeness criteria are different for the two ozone statistics (see Appendix C for details). This 5-year average is directly reported for parks with an in-park or nearby representative monitor in Alaska, Hawaii, Puerto Rico, and the Virgin Islands.
The 5-year averages are then interpolated across all monitoring locations in the contiguous US using an IDW method to estimate 5-year average values for this area. For individual parks, ozone estimates reported are the maximum values within park boundaries from this national-scale analysis.
▸ 2.2. Visibility Values
Visibility is monitored throughout the US by the Interagency Monitoring of Protected Visual Environments (IMPROVE) network. Every third day, 24-hour particulate samples are collected. These samples are analyzed for chemical composition and data are used to calculate annual visibility impairment as expressed by the haze index in deciviews (dv) or standard visual range in miles (mi).
Visibility values are derived using the following statistics:
- Haze index on clearest days
- The 20 percent of sampled days where visibility is most clear.
- Haze index on haziest days
- The 20 percent of sampled days where visibility is most limited.
- Haze index on mid-range days
- The 20 percent of sampled days where visibility is between the 40th and 60th percentiles.
- Haze index on most impaired days
- The 20% of sampled days where visibility is most limited (impaired) by human-caused air pollution.
- Standard visual range on clearest days
- The 20 percent of sampled days where visibility is most clear.
- Standard visual range on haziest days
- The 20 percent of sampled days where visibility is most limited.
Note that annual IMPROVE Regional Haze Rule datasets report annual values rounded to one hundred-millionth place (8 decimals). Annual values are rounded to the to the nearest tenth (2 decimals) for haze index statistics and nearest whole number for standard visual range statistics.
Each of the annual haze index statistics are averaged over a 5-year period for monitoring sites with at least 3-years of complete annual data. This 5-year average is directly reported for Class I parks and additional parks with in-park IMPROVE monitors. Note that there are monitoring sites that are classified as in-park but are slightly outside of park boundaries (i.e. within 1 km boundaries; see Appendix B for list of in-park visibility monitoring sites). Estimated 5-year averages are used for parks in the contiguous US that do not have in-park visibility monitoring. To estimate 5-year average values, monitor-specific 5-year averages are interpolated across all monitoring locations in the contiguous US using an IDW method. For individual parks, haze index estimates reported are the maximum values within park boundaries from this national-scale analysis.
Standard visual range statistics are reported using annual values as opposed to 5-year averages. Monitor-specific annual values are directly reported for Class I parks and additional parks with in-park IMPROVE monitors. Estimated annual values are used for parks in the contiguous US that do not have in-park visibility monitoring. To estimate annual values, monitor-specific annual values are interpolated across all monitoring locations in the contiguous US using an IDW method. For individual parks, standard visual range estimates reported are the maximum values within park boundaries from this national-scale analysis.
▸ 2.3. Wet Nitrogen and Sulfur Deposition Values
Atmospheric wet nitrogen and sulfur deposition are monitored across the United States as part of the National Atmospheric Deposition Program/National Trends Network (NADP/NTN). Every Tuesday, weekly precipitation samples are collected. These samples are analyzed for nitrate, ammonium, and sulfate concentration. Weekly concentration data are used to calculate annual precipitation-weighted mean concentrations measured in milligrams/liter (mg/L).
Wet nitrogen and sulfur deposition values are derived using the following six precipitation-weighted mean concentrations statistics:
- Ammonium (NH4+)
- Nitrate (NO3-)
- Sulfate (SO42-)
- Nitrogen (N)
- Nitrogen concentration reported is the combined nitrogen portion of nitrate (NO3-) and ammonium (NH4+). Molecular weight ratios are used to calculate the nitrogen portions of nitrate (NO3- concentration * 0.22581) and ammonium (NH4+ concentration * 0.77778) then these values are summed.
- Sulfur (S)
- Sulfur concentration reported is the sulfur portion of sulfate. Molecular weight ratio are used to calculate the sulfur portion of sulfate (SO42- concentration * 0.3338).
Annual ammonium, nitrate, sulfate, nitrogen, and sulfur precipitation weighted mean concentrations are averaged over a 5-year period at monitoring sites with at least 3 years of annual data that meet the following completeness criteria (Lehmann et al. 2005):
- Criterion 1: Percentage of time during the meteorological season for which valid samples are available ≥ 50%. Meteorological spring includes March, April, and May; summer includes June, July, and August; fall includes September, October, and November; and winter includes December, January, and February.
- Criterion 2: Percentage of time during the meteorological season for which valid precipitation amounts are available ≥ 75%.
- Criterion 3: Percentage of the total measured precipitation associated with valid samples ≥ 50% for the meteorological season.
For parks in Alaska, Hawaii, Puerto Rico, and the Virgin Islands with in-park or nearby representative monitors, monitor-specific 5-year averages are used to calculate wet deposition by multiplying average sulfate, nitrate, nitrogen, or sulfur concentrations (mg/L) by measured total annual precipitation (centimeters [cm]) and dividing by 10 to get deposition (kilograms per hectare [kg/ha]).
The 5-year averages are also interpolated across all monitoring locations in the contiguous US using an IDW method in order to estimate 5-year average values. For individual parks, minimum and maximum values within park boundaries are reported from this national-scale analysis. Estimated wet sulfate, nitrate, nitrogen, and sulfur depositions are then calculated by multiplying estimated 5-year average concentrations (mg/L) by normalized precipitation (centimeters [cm]) and dividing by 10 to get deposition (kilograms per hectare [kg/ha]). Normalized 30-year (1981–2010) annual precipitation values are used to calculate deposition to minimize interannual variation in deposition caused by fluctuations in precipitation (see Appendix A for more information).
▸ 2.4. Wet Mercury Deposition Values
Atmospheric wet mercury deposition is monitored across the United States as part of the National Atmospheric Deposition Program/Mercury Deposition Network (NADP/MDN). Every Tuesday, weekly precipitation samples are collected. These samples are analyzed for mercury concentration. Concentration data are used to calculate annual precipitation-weighted mean concentrations measured in nanograms per liter (ng/L).
Annual mercury precipitation weighted mean concentrations are averaged over a 3-year period at monitoring sites with 3 years of annual data that meet the following completeness criteria (Lehmann et al. 2005):
- Criterion 1: Percentage of time during the meteorological season for which valid samples are available ≥ 50%. Meteorological spring includes March, April, and May; summer includes June, July, and August; fall includes September, October, and November; and winter includes December, January, and February.
- Criterion 2: Percentage of time during the meteorological season for which valid precipitation amounts are available ≥ 75%.
- Criterion 3: Percentage of the total measured precipitation associated with valid samples ≥ 50% for the meteorological season.
For parks in Alaska, Hawaii, Puerto Rico, and the Virgin Islands with in-park or nearby representative monitors,monitor-specific 3-year averages are used to calculate wet deposition by multiplying average mercury concentrations (ng/L) by measured total annual precipitation (centimeters [cm]) and dividing by 100 to get deposition (micrograms per square meter [µg/m2]).
The 3-year averages are also interpolated across all monitoring locations in the contiguous US using an IDW method to estimate 3-year average values. For individual parks, minimum and maximum values within park boundaries are reported from this national-scale analysis. Estimated wet mercury deposition is calculated by multiplying estimated 3-year average concentrations (nanograms per liter [ng/L]) by normalized precipitation (cm) and dividing by 100 to get deposition (micrograms per square meter [µg/m2]).
Currently, these data are not available online. Please contact the NPS-ARD for mercury concentration and deposition values
▸ 2.5. Particulate Matter Values
Particulate matter (PM) is monitored across the US through air quality monitoring networks operated by the NPS, Environmental Protection Agency (EPA), states, and other agencies. Aggregated hourly PM concentration data are acquired from Federal Reference Method (FRM) or Federal Equivalent Method (FEM) monitors in the EPA Air Quality System (AQS) database. In addition, particulate matter data from the IMPROVE network are sometimes used when there are no FRM or FEM data available. Many FRM/FEM sites take measurements every day, while IMPROVE measures every 3rd day. Either hourly or daily data are used to calculate annual concentration statistics measured in micrograms per cubic meter of air (μg/m3) (see Appendix D for data acquisition and calculation methods).
Particulate matter values are derived using three concentration statistics:
- 98th percentile 24-hour average PM2.5 (particles with diameters < 2.5 micrometers) mass concentration
- The 24-hour average PM2.5 mass concentrations for a given day (midnight to midnight local standard time) is an integrated 24-hour mass measurement or is calculated from hourly concentration measurements. The 98th percentile value corresponds to the highest 2% of 24-hour mass values within a calendar year.
- Annual mean 24-hour average PM2.5 mass concentration
- The 24-hour average PM2.5 mass concentrations for a given day (midnight to midnight local standard time) is an integrated 24-hour mass measurement or is calculated from hourly concentration measurements. Arithmetic mean of quarterly 24-hour means within a calendar year that has four quarters meeting data completeness criteria.
- 2nd maximum 24-hour average PM10 (particles with diameters < 10 micrometers) mass concentration
- 24-hour PM10 mass concentration for a given day (midnight to midnight local standard time) is an integrated 24-hour mass measurement or is calculated from hourly concentration measurements. PM10 is typically measured every sixth day, which results in about 60 values per year. Some sites take measurements every day. The 2nd maximum 24-hour PM10 concentration within a calendar year is the second highest 24-hour values of the year.
Each of these annual statistics is averaged over a 3-year period at monitoring sites with at least 3-years of complete annual data (see Appendix D for completeness criteria details).
Estimated values are not calculated for particulate matter. Monitor-specific 3-year averages are directly reported from in-park or nearby monitors that are considered representative (i.e within 10 km of park boundaries; see Appendix B for a list of in-park and nearby representative monitoring sites). Monitoring sites can measure both PM2.5 and PM10 but there are sites that only measure PM2.5 or PM10. In those cases, parks may either have data for PM2.5 or PM10 or have two representative particulate matter monitors where one monitors PM2.5 and the other monitors PM10. When selecting representative PM monitors, FRM or FEM monitors take precedence over in-park or nearby representative IMPROVE monitors. This is because IMPROVE data are collected only every 3 days resulting in less data for condition assessments. Note that the methodology for calculating annual concentration values from FRM/FEM and IMPROVE monitors differ. See Appendix D for particulate matter data acquisition and calculation methods.
3. Assessing Conditions
Conditions convey the status of air quality. Air quality conditions provide parks, decision makers, and the public the opportunity to explore past and current air quality at individual parks in context of risk to human health or ecosystems. NPS-ARD uses six specific measures to evaluate air quality conditions at NPS units (see Table 1).
Table 1. Indicators and specific measures for assessing air quality conditions.
Indicator of air quality |
Specific Measure(s) |
---|---|
Ozone / human health |
4th-highest daily maximum 8-hour average ozone concentration |
Ozone / vegetation health |
3-month maximum 12-hour W126 |
Visibility |
Visibility minus natural condition on mid-range days |
Sulfur |
Wet deposition |
Nitrogen |
Wet deposition |
Particulate matter |
PM2.5 annual, PM2.5 24-hour, and PM10 24-hour |
Condition categories (Poor, Fair, Good), are assigned by comparing current air quality values discussed in section 2 to the NPS-ARD benchmarks. Benchmarks for specific measures of air quality have been established using regulatory standards and best available scientific knowledge. In some cases, condition categories are adjusted based on risk assessments or other information to better represent park air quality condition. This section describes the specific benchmarks used for each indicator of air quality as well as procedures for assigning condition categories.
Air quality conditions in summary tabs on the NPS air quality conditions and trends webpage and national summary maps are indicated by the color of the circle, where red is Poor, yellow is Fair, and green is Good.
▸ 3.1. Ozone Condition / Human Health
Ozone is harmful to humans when they breathe it in and to plants when they respire. The primary National Ambient Air Quality Standard (NAAQS) for ground-level ozone is set by the EPA and is based on human health effects. The current NAAQS for ozone was established in 2015 and is set at 70 ppb for the 3-year average of the 4th-highest daily maximum 8-hour average ozone concentration. The NPS-ARD benchmarks for the human health risk from ozone condition are based on the EPA Air Quality Index (AQI) breakpoints.
Ozone human health conditions (Poor, Fair, Good) are based on ozone values identified in section 2.1 compared to NPS-ARD benchmarks (see Table 2). Ozone values for each NPS area are the estimated or monitor-specific 5-year average of the 4th-highest daily maximum 8-hour average ozone. Ozone concentrations greater than or equal to 71.0 ppb are assigned a Poor condition. Ozone concentrations from 55.0–70.9 ppb are assigned Fair condition. A Good condition is identified when ozone concentrations are less than or equal to 54.9 ppb.
Table 2. Benchmarks for human health ozone condition (2015 ozone standard).
Condition category |
Ozone concentration (ppb) |
---|---|
Poor |
≥ 71.0 |
Fair |
55.0–70.9 |
Good |
≤ 54.9 |
Historic Condition Methods: The human health ozone condition assessments for end-years prior to 2014 used NPS-ARD benchmarks that aligned with the 2008 ozone standard (Table 3). Ozone conditions assessments for end-years prior to 2014 also had adjustments based on nonattainment area designation. In instances where the NPS unit fell within an area designated by the EPA as nonattainment for the 2008 ground-level ozone standard, the ozone condition was elevated to the Poor category. See Appendix E for NPS units that are in designated non-attainment area(s) for the 2008 standard. Appendix E also has NPS units that are in designated non-attainment area(s) for the 2015 standard. The 2015 non-attainment information does not impact the condition assessments and is for information only.
Table 3. Historic benchmarks for human health ozone condition for 2009 to 2013 end years (2008 standard).
Condition category |
Ozone concentration (ppb) |
---|---|
Poor |
≥ 76.0 |
Fair |
61.0–75.9 |
Good |
≤ 60.9 |
▸ 3.2. Ozone Condition / Vegetation Health
Long-term exposures to ground-level ozone can cause injury to ozone-sensitive plants. Over the course of a growing season ozone can damage plant leaf tissues decreasing photosynthetic capacity, weakening plants, and making them less resistant to disease and insect infestations. The W126 metric is a biologically relevant measure that focuses on plant response to continuous ozone exposure. This measure is a better predictor of vegetation response to ozone exposure than the metric used for the human health standard (4th-highest daily maximum 8-hour average ozone concentration) because damage develops slowly under continuous exposure. The W126 metric equation preferentially weights the higher ozone concentrations that are more likely to cause plant damage. It sums all of the weighted concentrations during daylight hours because this is when the majority of gas exchange occurs between plants and the atmosphere. The highest 3-month period that occurs during the growing season (March–September) is reported in parts per million-hours (ppm-hrs). See Appendix C for calculation methods for the ozone W126 statistic.
NPS-ARD benchmarks for the W126 metric are based on information in EPA’s Policy Assessment for the Review of the ozone National Ambient Air Quality Standards (EPA 2014), which outlines use of the W126 metric for assessing plant response to ground-level ozone. This document also compiled scientific evidence about impacts to vegetation from ground-level ozone. Research has found that for a W126 value of:
- ≤ 7 ppm-hrs, tree seedling biomass loss is ≤ 2 % per year in sensitive species; and
- ≥13 ppm-hrs, tree seedling biomass loss is 4–10 % per year in sensitive species.
The NPS-ARD applies the lower concentration level (i.e. W126 of ≤ 7 ppm-hrs) as the basis for the good condition benchmark to more conservatively evaluate air quality effects on sensitive vegetation in parks.
Ozone vegetation health conditions (Poor, Fair, Good) are based on ozone values identified in section 2.1 compared to NPS-ARD benchmarks (see Table 4). Ozone values for each NPS area are the estimated or monitor-specific 5-year average of the 3-month maximum 12-hour W126 index. A W126 index greater than 13 ppm-hrs is assigned a Poor condition. A W126 index from 7–13 ppm-hrs is assigned Fair condition. A Good condition is identified when W126 index is less than 7 ppm-hrs.
Table 4. Benchmarks for vegetation health ozone condition.
|
W126 (ppm-hrs) |
---|---|
Poor |
> 13.0 |
Fair |
7.0–13.0 |
Good |
< 7.0 |
▸ 3.3. Visibility Condition
Particles in the atmosphere – from both natural (e.g. wildfires) and human-caused sources (e.g., power plants) – scatter and absorb light, creating a haze that limits how far and how well we can see. The clarity of park views is affected by human-caused pollution in virtually all national parks.
NPS-ARD evaluates visibility conditions using the measure of haze index on mid-range days. Mid-range days capture overall trends in human-caused contributions to haze by reducing the influence of episodic natural events such as wildfires and dust that often dominate the haziest days, especially in the Western US.
The Regional Haze Rule established a national goal to return visibility to natural visibility in Class I areas. See Appendix F for estimated natural visibility in NPS units. Based on this national goal, the NPS-ARD set a visibility benchmark for Good of less than 2 dv above natural visibility for all NPS units, regardless of class designation. The NPS-ARD chose visibility benchmarks for Poor and Fair condition categories to reflect the variation in visibility conditions across the monitoring network (see Table 5).
Visibility conditions (Poor, Fair, Good) are based on visibility values identified in section 2.2 compared to NPS-ARD benchmarks (see Table 5). Visibility values for each NPS area are the estimated or monitor-specific 5-year average of the haze index on mid-range days minus the natural visibility on mid-range days. A result greater than 8 dv is assigned a Poor condition. A result from 2–8 dv is assigned Fair condition. A Good condition is identified when the result is less than 2 dv.
Table 5. Benchmarks for visibility condition.
Condition category |
Deciview amount above natural visibility |
---|---|
Poor |
> 8.0 |
Fair |
2.0–8.0 |
Good |
< 2.0 |
Haze index on most impaired days is a statistic that shows park visibility conditions on days that are most affected by human-caused pollution. Visitors experience parks throughout the year and not just on the most impaired days. As a result, the statistic used currently for conditions or haze index on mid-range days, better captures the average visibility impairment experienced by visitors. Haze index on most impaired days is a statistic that shows park visibility conditions on days that are most affected by human-caused pollution. This is interesting and helps the NPS work with regulators to target emission reductions and understand how air pollution is affecting visibility in parks. Haze index on most impaired days is also a useful statistic for assessing human effects on wilderness areas. However, people cannot visually distinguish natural haze (e.g., from wildfires) and haze from human caused pollution. For this reason, the visibility condition is based on the haze index on mid-range days to capture the average visibility impairment experienced by visitors. This statistic is helpful in tracking trends for wilderness character assessments where the goal is to evaluate changes to the natural environment from human-caused changes.
▸ 3.4. Nitrogen and Sulfur Deposition Condition
Excess nitrogen deposited from the air may have harmful effects on nature, including acidification and nutrient imbalances. Airborne nitrogen and sulfur are deposited to the earth’s surface through atmospheric deposition. When nitrogen or sulfur is removed from the atmosphere with rain, snow, or other precipitation it is called wet deposition. Dry deposition occurs when nitrogen or sulfur is removed from the atmosphere in the absence of precipitation.
While ecosystems respond to total (wet and dry) deposition, assessment of nitrogen and sulfur atmospheric deposition condition is based solely on wet deposition. Wet deposition is used as a surrogate for total deposition because wet deposition is the most widely available source of measured deposition data. NPS-ARD selected a wet deposition threshold of 1.0 kg/ha/yr wet nitrogen or sulfur deposition as the level below which natural ecosystems are likely protected from harm. This level is based on studies linking early stages of aquatic health decline correlated with 1.0 kg/ha/yr wet deposition of nitrogen both in the Rocky Mountains (Baron et al. 2011), and in the Pacific Northwest (Sheibley et al. 2014).
Nitrogen or sulfur conditions (Poor, Fair, Good) are based on deposition values identified in section 2.3 compared to NPS-ARD benchmarks (see Table 6). Deposition values for each NPS area are the maximum estimated or monitor-specific 5-year average of wet sulfur or nitrogen deposition. Although both minimum and maximum values are reported for parks, condition assessments rely on maximum estimated values to more conservatively characterize ecosystem sensitivity in parks. Precipitation, and therefore deposition, is often highest at high elevations. In parks with a wide range of precipitation and/or elevation, the maximum and minimum values can be significantly different. The maximum value provides a more conservative context for condition assessment while the minimum value may indicate the degree to which portions of the park have lower levels of deposition and potentially lower risk. In parks with a narrow range of annual precipitation and/or elevation, the maximum and minimum values are usually very similar.
Wet deposition greater than 3 kg/ha/yr is assigned a Poor condition. Wet deposition from 1–3 kg/ha/yr is assigned Fair condition. A Good condition is identified when wet deposition is less than less than 1 kg/ha/yr.
Table 6. Benchmarks for nitrogen and sulfur deposition condition.
Condition category |
Wet deposition (kg/ha/yr) |
---|---|
Poor |
> 3.0 |
Fair |
1.0–3.0 |
Good |
< 1.0 |
Condition adjustments: Nitrogen or sulfur deposition condition is adjusted based on results from national assessment reports that identified ecosystems and resources at risk to acidification and nitrogen enrichment in national parks. These reports provide a relative risk assessment of acidification and nutrient enrichment impacts from atmospheric nitrogen and sulfur deposition for parks in 32 inventory & monitoring networks. If park ecosystems are ranked “very high” in sensitivity to acidification or nutrient enrichment effects from atmospheric deposition relative to all inventory & monitoring parks (Sullivan et al. 2011a; Sullivan et al. 2011b), the condition category is adjusted to the next most conservative condition category (see Appendix G for a list of parks with condition adjustments).
Note that the methodology for nitrogen and sulfur deposition condition assessments will be improved in coming years by using total deposition estimates and established critical loads. This will allow conditions to be set based on exceedance of a threshold of sulfur or nitrogen deposition known to negatively impact an ecosystem component within the park.
▸ 3.5. Mercury Condition
Airborne mercury from air pollution sources, including coal-fired power plants, is deposited to park ecosystems through atmospheric deposition. Behavioral, neurological, and reproductive effects of toxic compounds including mercury can occur in fish, birds, wildlife, and even humans they build up in the food chain. The NPS-ARD assesses mercury condition using a combination of risk to biota, wet mercury deposition measurements, and landscape sensitivity to mercury methylation. Currently, these data are not available online. Please contact the NPS-ARD for mercury conditions and methodology details.
▸ 3.6. Particulate Matter Condition
Particulate matter is composed of extremely small particles and liquid droplets suspended in air. These include acids (such as nitrates and sulfates), organic chemicals, metals, soil or dust particles, and allergens. Inhalable particles of human health concern are classified as PM2.5 or “fine particles”, which are 2.5 micrometers in diameter or less, and PM10, which have diameters of 10 micrometers or less. Breathing small particles can cause human health effects including chest pain, respiratory irritation, and reduced lung function. Particulate matter also scatters and absorbs light creating haze that influences visibility. Visibility conditions are described in section 3.3.
The particulate matter condition is based on the NAAQS for PM2.5 and PM10, which are established by the EPA to protect human health. The PM2.5 primary standard is 12 micrograms per cubic meter (µg/m3) annually (3-year average of weighted annual mean) and 35 µg/m3 for 24-hours (3-year average of the 98th percentile of 24-hour concentrations). The primary and secondary NAAQS for PM10 is 150 µg/m3 for 24-hours (not to be exceeded more than once per year over 3 years). EPA Air Quality Index (AQI) breakpoints were used to develop benchmarks.
Each of the particulate matter measures is assigned a condition ((Poor, Fair, Good). These individual conditions are based on particulate matter values identified in section 2.5 compared to NPS-ARD benchmarks (see Table 10). Particulate matter values for each NPS area are the monitor-specific 3-year averages (98th percentile of 24-hour PM2.5 concentration, weighted annual mean 24-hour PM2.5 concentration, and 2nd maximum 24-hour PM10 concentration). An overall particulate matter condition for a park is based on one or a combination of multiple particulate matter measures. The measurement with the most conservative condition category determines the overall particulate matter condition.
Overall PM condition is Poor if the 98th percentile 24-hour PM2.5 concentration is ≥ 35.5 µg/m3, weighted annual mean 24-hour PM2.5 concentration is ≥ 12.5 µg/m3, or 2nd maximum 24-hour PM10 concentration is ≥ 155 µg/m3. Overall PM condition is Fair if none of the PM measures meet the criteria for Poor AND the 98th percentile 24-hour PM2.5 concentration is 12.1–35.4 µg/m3, weighted annual mean 24-hour PM2.5 concentration is 4.1–12.4 µg/m3, or 2nd maximum 24-hour PM10 concentration is 55–154 µg/m3. Overall PM condition is Good if none of the PM measures meet the criteria for Poor or Fair meaning that 98th percentile 24-hour PM2.5 concentration is ≤ 12.0 µg/m3, weighted annual mean 24-hour PM2.5 concentration is ≤ 4 µg/m3, or 2nd maximum 24-hour PM10 concentration is ≤ 54 µg/m3.
Table 7. Benchmarks for particulate matter condition. Particulate matter concentrations are averaged over 3-years and are expressed in micrograms per cubic meter (µg/m3).
Condition category |
98th percentile 24-hour PM2.5 concentration |
Weighted annual mean 24-hour PM2.5 concentration |
2nd maximum 24-hour PM10 concentration |
---|---|---|---|
Poor |
≥ 35.5 |
≥ 12.5 |
≥ 155 |
Fair |
12.1–35.4 |
4.1–12.4 |
55–154 |
Good |
≤ 12 |
≤ 4 |
≤ 54 |
▸ 3.7. Overall Air Quality Condition
Available air quality condition assessments at each NPS unit are rolled into a single air quality condition. To determine the combined condition, each Poor (red) symbol is assigned zero points, each Fair (yellow) symbol is assigned 50 points, and each Good (green) symbol, 100 points. The average is calculated from all condition assessments and applied the scale below (Table 11) to determine the overall air quality condition. Where averages have decimal points, the overall score is truncated to the nearest whole number.
Table 8. Scale for overall air quality condition.
Condition score |
Overall air quality condition |
Overall air quality condition symbol color |
---|---|---|
0–33 |
Poor |
|
34–66 |
Fair |
|
67–100 |
Good |
Historic Condition Adjustments: For end years 2009–2013, NPS units that were in EPA designated ozone or particulate matter nonattainment areas were automatically placed in the Poor condition category (see Appendix E: Parks in EPA Designated Nonattainment Areas). Starting with end year 2014, the overall condition is no longer adjusted based on nonattainment designation.
4. Assessing Trends
NPS-ARD uses a non-parametric regression technique called the Kendall-Theil method to determine statistically significant trends. Kendal-theil methods for computing the trend line and test for significance are explained in Statistical Methods in Water Resources section “10.1 Kendall-Theil Robust Line”. Short-term trends are computed from data collected over a 10-year period at in-park or nearby representative monitors (see Appendix B for a list of in-park and representative sites). Short-term trends are calculated for sites that have at least 6 years of annual data and an annual value for the end year of the reporting period.
Long-term trends are computed from the entire monitoring record at in-park monitors or nearby representative monitors. Long-term trends are calculated for sites that have at least 60 percent of annual data, no data gaps greater than 4 years, and an annual value for the reporting end year.
On the NPS park conditions & trends web page, a chart on the trend tab may still be displayed without a trend line if data completeness criteria for a trend are not met. Ten-year charts will not be displayed for a monitoring site with 6 years of missing data. Long-term trend charts will not be displayed for a monitoring site with 11 years of missing data.
The summary tabs on the NPS park conditions & trends web page display only the 10-year trends, which are represented by arrows. All improving (up arrows) and deteriorating (down arrows) trends have at least 90% probability of being correct (those with p-values ≤ 0.10). Statistically significant (p-value ≤ 0.10) trends with zero slope are represented by flat arrows. Varied trends (up and down arrow) result from a combination of improving and degrading trends for either particulate matter or overall air quality trends (see section 4.6). Parameters with no statistically significant trend (p-value ≥ 0.10) are also represented by flat arrows.
Table 9. Symbology for trend categories
Trend category |
Trend Symbol |
---|---|
Improving |
|
Deteriorating |
|
Unchanging |
|
Varied |
▸ 4.1. Ozone Trends
Trends are evaluated using the following ozone concentration statistics:
- Human health: 4th-highest daily maximum 8-hour average ozone concentrations in ppb
- The daily maximum 8-hour average ozone concentration for a given day is the highest of the 17 consecutive 8-hour averages, beginning with the 8-hour period spanning from 7:00 a.m. to 3:00 p.m. and ending with the 8-hour period from 11:00 p.m. to 7:00 a.m. the following day (i.e., the 8-hour averages for 7:00 a.m. to 11:00 p.m.). The 4th-highest daily maximum 8-hour average ozone concentration within in a calendar year is reported in parts per billion (ppb). See Appendix C for more information.
- Vegetation health: 3-month maximum 12-hour W126 index in ppm-hrs
- The W126 index preferentially weighs the higher ozone concentrations most likely to affect plants and sums all the weighted concentrations during daylight hours (8 a.m. to 8 p.m.). The highest 3-month period that occurs during the growing season (March–September) is reported in parts per million-hours (ppm-hrs). See Appendix C for W126 index calculation methods.
Concentrations are trended over a 10-year period and the entire data record for each reporting year. For a year to be considered in trend analyses, it must meet annual completeness criteria. Annual completeness criteria are different for the two ozone statistics (see Appendix C for details).
Ozone trends are computed for parks with an in-park or nearby representative ozone monitor that is within 10 km of park boundaries. Monitors operated by NPS take precedence over other nearby monitors. In cases where the park has more than one monitor operated by the NPS, the monitor with the longest monitoring history is selected to represent the park. There are a handful of representative monitors that are no longer the closest monitor within a 10 km radius but are retained as the representative monitor to maintain consistent historic trend records. See Appendix B for a list of in-park and representative ozone monitoring sites.
▸ 4.2. Visibility Trends
Trends are evaluated using the following haze index statistics:
- Haze index on clearest days
- The 20 percent of sampled days where visibility is most clear.
- Haze index on haziest days
- The 20 percent of sampled days where visibility is most limited.
- Haze index on mid-range days
- Mid-range days are sampled days where visibility is between the 40th and 60th percentiles.
- Haze index on most impaired days
- Most impaired days are the 20% of sampled days each year where measured visibility has the highest contribution from anthropogenic pollution relative to natural visibility.
Concentrations are trended over a 10-year period and the entire data record for each reporting year. For a year to be considered in trend analyses, it must meet annual completeness criteria. Annual completeness criteria are based on EPA’s Guidance for Tracking Progress Under the Regional Haze Rule and are predetermined by IMPROVE in the annual Regional Haze Rule data summaries and calculated values. Accordingly, all reported annual values in the Regional Haze Rule data summaries meet completeness criteria.
Trends are computed for parks with in-park or nearby representative monitors. Representative IMPROVE monitoring sites were selected based the following criteria: within +/- 100 feet or 10% of maximum and minimum elevation of the park and at a distance of no more than 150 kilometers from park boundaries. IMPROVE representative monitors are not assigned to parks with a urban land-use category unless the monitor is also within the urban land-use category. See Appendix B for a list of in-park and representative visibility monitoring sites.
On the NPS park conditions & trends, the summary tab displays an overall visibility trend derived by combining trends of the haze index values on the 20% haziest days and the 20% clearest days. If the haze index trend on the 20% clearest days is deteriorating, the overall visibility trend is reported as deteriorating. Otherwise, the haze index trend on the 20% haziest days is reported as the overall visibility trend. The overall trend combination logic is consistent with visibility goals in the Regional Haze Rule, which include improving visibility on the haziest days and allowing no deterioration on the clearest days.
▸ 4.3. Nitrogen and Sulfur Deposition Trends
How wet nitrogen and sulfur deposition change over time is evaluated by looking at the trends of pollutant concentrations in precipitation (micro equivalents/liter) so that yearly variations in precipitation amounts do not influence trend analyses. Measured concentrations from the NADP-NTN monitoring sites are converted from miligrams per liter (mg/L) to microequavalents per liter (ueq/L) using the following multipliers:
-
- Ammonium (NH4+): 55.44
- Nitrate (NO3-): 16.13
- Sulfate (SO42-): 20.83
This conversion is necessary because nitrate ion has one negative charge (NO3-) and ammonium has one positive charge (NH4+). Converting concentrations to microequavalents is a way of normalizing for ionic charge so that ion concentrations can be compared to each other.
Trends are evaluated using the following concentration statistics:
- Ammonium (NH4+)
- annual precipitation-weighted mean concentration
- Nitrate (NO3-)
- annual precipitation-weighted mean concentration
- Sulfate (SO42-)
- annual precipitation-weighted mean concentration
- Nitrogen (N)
- annual precipitation weighted mean concentration. Nitrogen concentration is reported by summing the nitrogen portion of nitrate (NO3-) and ammonium (NH4+) concentrations. Molecular weight ratios are used to calculate the nitrogen portions of nitrate (NO3- concentration * 0.22581) and ammonium (NH4+ concentration * 0.77778).
- Sulfur (S)
- annual sulfur precipitation-weighted mean concentrations. Sulfur concentration is the sulfur portion from sulfate. Molecular weight ratio is used to calculate the sulfur portion of sulfate (SO42- concentration * 0.3338).
Additionally, wet deposition and precipitation trends are displayed for parks with in-park or representative monitors:
-
- total annual precipitation measured in centimeters (cm)
- annual wet nitrogen deposition, measured in kilograms/hectare/year (kg/ha/yr). Nitrogen deposition is the nitrogen portion of nitrate and ammonium as described in the bullet above for nitrogen concentration. Wet nitrogen deposition equals nitrogen concentration (mg/L) multiplied by the total annual precipitation (cm) and divided by 10.
- annual wet sulfur deposition, measured in kg/ha/yr. Sulfur deposition is the sulfur portion of sulfate as described in the bullet above for sulfur concentration. Wet sulfur deposition equals precipitation multiplied by nitrogen concentration. Wet sulfur deposition equals nitrogen concentration (mg/L) multiplied by the total annual precipitation (cm) and divided by 10.
Annual concentration, deposition, or precipitation values are trended over a 10-year period and the entire data record. For a year to be considered in trend analyses, it must meet meteorological season completeness criteria referenced in section 2.3.
Nitrogen and sulfur trends are computed for parks with an in-park or representative NADP/NTN wet deposition monitor that is within 16 km of park boundaries. See Appendix B for a list of in-park and representative NADP/NTN monitoring sites.
On the NPS park conditions & trends, the summary tab only display the annual nitrogen or precipitation weighted mean concentration trends. When viewing deposition trends, it is important to consider the relative influence of precipitation and concentration. For example, locations with low nitrogen concentrations and more rainfall may experience more deposition than places with high nitrogen concentrations and considerably less rainfall. Excess nitrogen deposited from the air may have harmful effects on nature, including acidification, nutrient imbalances, and loss of biodiversity.
▸ 4.4. Mercury Deposition Trends
Atmospheric wet mercury deposition trends are evaluated using pollutant concentrations in precipitation (micro equivalents/liter) so that yearly variations in precipitation amounts do not influence trend analyses. Mercury concentrations in precipitation are trended over a 10-year period and the entire data record for each reporting year. For a year to be considered in the trend analysis, it must meet the same meteorological season completeness criteria referenced in in section 2.4.
Mercury trends are computed for parks with a representative NADP/MDN wet deposition monitor that is within 16 km of park boundaries. Mercury concentrations are trended over a 10-year period and the entire data record for each reporting year. See Appendix B for a list of NADP/MDN in-park and representative monitoring sites.
Currently, mercury deposition trends are not available online. Please contact the NPS-ARD for these trends.
▸ 4.5. Particulate Matter Trends
Trends are evaluated using the following particulate matter concentration statistics relevant to human health:
- 98th percentile 24-hour average PM2.5 (particles with diameters < 2.5 micrometers) mass concentration
- The 24-hour average PM2.5 mass concentrations for a given day (midnight to midnight local standard time) is an integrated 24-hour mass measurement or is calculated from hourly concentration measurements. The 98th percentile value corresponds to the highest 2% of 24-hour mass values within a calendar year.
- Annual mean 24-hour average PM2.5 mass concentration
- The 24-hour average PM2.5 mass concentrations for a given day (midnight to midnight local standard time) is an integrated 24-hour mass measurement or is calculated from hourly concentration measurements. Arithmetic mean of quarterly 24-hour means within a calendar year with data that are at least 75 percent complete in each calendar quarter.
- 2nd maximum 24-hour average PM10 (particles with diameters < 10 micrometers) mass concentration
- 24-hour PM10 mass concentration for a given day (midnight to midnight local standard time) is an integrated 24-hour mass measurement or is calculated from hourly concentration measurements. PM10 is typically measured every sixth day, which results in about 60 values per year. Some sites take measurements every day. The 2nd maximum 24-hour PM10 concentration within a calendar year is the second highest 24-hour values of the year.
Particulate matter concentrations are trended over a 10-year period and the entire data record for each reporting year. For a year to be considered in trend analyses, it must meet annual completeness criteria (see Appendix D).
Particulate matter trends are computed for parks with in-park or nearby monitors that are considered representative (i.e. within 10 km of park boundaries). Monitoring sites can measure both PM2.5 and PM10 but there are sites that only measure PM2.5 or PM10. In those cases, parks may either have data for PM2.5 or PM10 or have two representative particulate matter monitors where one monitors PM2.5 and the other PM10. Federal Reference Method (FRM) or Federal Equivalent Method (FEM) monitors take precedence over in-park or nearby representative IMPROVE monitors. This is because IMPROVE data are collected only every 3 days resulting in less data for condition assessments. Note that the methodology for calculating annual concentration values from FRM/FEM and IMPROVE monitors differ. See Appendix D for particulate matter data acquisition and calculation methods. There are a handful of representative monitors that are no longer the closest monitor within a 10 km radius but are retained as the representative monitor to maintain consistent historic trend records. See Appendix B list of in-park and representative particulate monitoring sites.
Overall trends for particulate matter are determined using the following rules:
- Improving trends
- only one PM2.5 trend available and it is improving trend
- both PM2.5 trends are improving
- combination of one improving PM2.5 trend and PM2.5 trend is relatively unchanging
- where PM2.5 trends are not available, and the PM10 trend is improving
- Deteriorated
- only one PM2.5 trend available and it is deteriorating
- if both PM2.5 trends and both are deteriorating
- if 1 PM2.5 trend is deteriorating and 1 PM2.5 trend is relatively unchanging
- if PM2.5 trend is not available, and the PM10 trend is deteriorating
- Relatively unchanged
- one relatively unchanging PM2.5 trend
- both PM2.5 trends are relatively unchanging
- where PM2.5 trends are not available, and the PM10 trend is relatively unchanging
- Varied
- combination of PM2.5 trends where one is improving and one is deteriorating
- No trend
- No particulate matter trend information
▸ 4.6. Overall Air Quality Trend
Available air quality trends at each NPS unit are rolled into a single air quality trend. An overall air quality trend for a park is based on one or a combination of multiple parameter trends using the following rules:
- Improving trend (upward arrow symbol):
- only one trend available and it is improving
- combination of multiple parameter trends that are all improving
- combination of multiple parameter trends where the relatively unchanging trend(s) has a Good condition AND the improving trend(s) has a Poor/Fair/Good condition
- Deteriorating trend (downward arrow symbol):
- only one trend available and it is deteriorating
- combination of multiple parameter trends that are all deteriorating
- combination of multiple relatively unchanging trend(s) AND deteriorating trend(s)
- Relatively unchanging trend (flat arrow symbol):
- only one trend available and it is relatively unchanging
- combination of multiple trends that are all relatively unchanging
- Varied trend (upward and downward arrow symbol):
- combination of multiple trends where some are improving and some are deteriorating
- combination of multiple trends where the relatively unchanging trend(s) has Fair/Poor condition(s) AND other trend(s) is improving
- No trend
- no trend information is available for any of the parameters
5. Assigning Confidence
▸ 5.1. Confidence in Condition
The degree of confidence for each condition assessment is based on how well the nearest monitoring site(s) represents air quality for a park. The representativeness of a monitor depends on the pollutant, network, distance from the park, and local site characteristics. The degree of confidence is rated as either High or Medium.
The confidence is High if condition assessments are based on:
- estimated values for parks that have in-park or nearby representative monitors
- visibility, ozone, and deposition monitor-specific values directly reported from on-site or nearby representative monitors
- particulate matter monitor-specific values directly reported from in-park or nearby representative FRM/FEM monitors
The confidence is Medium if condition assessments are based on:
- estimated values for parks with more distant monitors
- particulate matter monitor-specific values directly reported from in-park or nearby representative IMPROVE monitors
Low confidence is not assigned for ozone, visibility, nitrogen deposition, sulfur deposition, or particulate matter condition assessments.
The degree of confidence in the overall condition is assigned a High rating only when all individual indicators have High confidence ratings. In all other instances, the overall degree of confidence is rated as Medium.
In air quality summary tabs, the degree of confidence in assigned condition is represented by the thickness of the outside line of the condition symbol (circle). A thick outline represents High confidence, a thin outline represents Medium confidence, and a dashed outline represents Low confidence.
Table 10. Symbology for confidence categories
Confidence category |
Confidence Symbol |
---|---|
High |
|
Medium |
|
Low |
▸ 5.2. Confidence in Trend
The degree of confidence for all available air quality trend analyses is rated High because trends are only computed for parks with an in-park or representative monitor.
6. Presenting Chemical Composition of Haze and Deposition
▸ 6.1. Haze
Speciated fine particulate matter and total mass are measured every third day at IMPROVE monitoring sites situated to represent air quality at 155 Class I areas and other rural environments. These data are used to estimate the contribution of the major chemical species to ambient particulate matter and in turn, their contribution to haze (i.e., visibility impairment). Monitored particulate matter constituents are ammonium sulfate, ammonium nitrate, organic mass, elemental carbon, fine soil, fine sea salt, and coarse mass.
Visibility impairment is reported as either light extinction or deciview. Light extinction has units of inverse megameters [1/Mm] and is a measure of the loss of image forming light from scenic features to the observer due to scattering and absorption by particles and gases per unit length. Light extinction is dependent on the optical properties of the particulate matter and varies by its chemical constituents. For example, a given mass of ammonium sulfate will scatter more light than the same quantity of soil particles. Stacked bar charts show the contribution of each constituent to overall light extinction for a given time period.
Composition and visibility data are grouped by haziest days (sampled days with the highest 20% of aerosol calculated light extinction) and clearest days (sampled days with the lowest 20% of aerosol calculated light extinction) in each year. Data are displayed by sample day, month, and year. Also the 5-year average of the clearest and haziest days are compared to the respective estimated natural visibility conditions on clearest or haziest days. Note that annual IMPROVE Regional Haze Rule datasets report values rounded to one hundred-millionth place (8 decimals). For chemical composition of haze, light extinction values are rounded to the hundredth decimal place (2 decimals).
▸ 6.2. Nitrogen and Sulfur Deposition
Wet and dry components make up inorganic nitrogen and sulfur deposition. The nitrogen and the sulfur portion of each component is reported using annual or five-year average statistics in kg/ha/yr. Molecular weight ratios are used to calculate nitrogen and sulfur portions of each component using the following equations:
- Nitrogen portion of nitrate concentration (NO3) = NO3 * 0.2259
- Nitrogen portion of ammonium concentration (NH4+) = NH4+* 0.7778
- Nitrogen portion of nitric acid concentration (HNO3) = HNO3 * 0.2223
- Sulfur portion of sulfate concentration (SO42-) = SO42- * 0.3338
- Sulfur portion of sulfur dioxide concentration (SO2) = SO2 * 0.5005
Wet deposition data (ammonium, nitrate, sulfate) are measured at NADP-NTN monitoring sites. Dry deposition data (nitric acid, ammonium, nitrate, sulfate and sulfur dioxide) are derived using the Total Deposition (TDEP) model (for more details on the methodology see Schwede and Lear 2014) and measurements from CASTNET sites. Note that five-year averages are not reported for end-years of 2000 thru 2003. Also, dry deposition data for Denali National Park & Preserve are derived using the Multi-Layer Model (MLM) and measurements from CASTNET site. Note that for 2015 there were missing data. The following steps were taking to run the MLM model:
- Used valid meteorological data from the CASTNET database
- Updated relative humidity and precipitation data from Air Resource Specialists for 8/1/13– 6/30/15
- Where data were still missing and NADP data available, updated with NADP hourly precipitation data for the same time period
- Where original data were invalid, updated with Bowker replacement solar radiation data for 6/1/15–11/30/15.
7. Computing Ozone and Particulate Matter Exceedances
Exceedance charts provide an annual snapshot of the number of days that exceeded the National Ambient Air Quality Standard for either ozone or particulate matter.
The current (2015) ozone standard is an 8-hour average ozone concentration of 70 ppb. This standard is violated when the 3-year average of the annual 4th highest daily maximum 8-hour average ozone concentration is greater than or equal to 71 ppb. An exceedance day occurs when the daily maximum 8-hour average ozone concentration is 71 ppb or higher for a calendar day. The total number of exceedance days in a calendar year is reported for monitoring sites having valid data for 60 to 75% of the months within an ozone season. A a month is considered in this analysis if there is at least one daily max 8-hour average ozone concentration. Table 11 shows the minimum number of months required to meet the 60 to 75% ozone season completeness criteria.
Table 11. Ozone season completeness criteria for annual ozone exceedance information.
Number of months in an ozone season | Minimum number of months with at least one daily max value |
---|---|
4 | 3 |
5 | 3 |
6 | 4 |
7 | 5 |
8 | 6 |
9 | 6 |
10 | 7 |
11 | 8 |
12 | 9 |
The current 24-hour fine particulate matter standard is a 24-hour average PM2.5 concentration of 35 μg/m3. This standard is violated when the 3-year average of the 98th percentile 24-hour PM2.5 concentration is greater than or equal to 35.5 μg/m3. An exceedance day occurs when the 24-hour average PM2.5 concentration is 35.5 μg/m3 or higher for a calendar day. The total number of exceedance days in a calendar year is reported for monitoring sites having valid data for 7 out of 12 months in a calendar year. A month is considered valid when there is at least one 24-hour average PM2.5 concentration.
The current 24-hour particulate matter standard is a 24-hour average PM10 concentration of 150 μg/m3. This standard is violated when the 24-hour PM10 concentration exceeds 155 μg/m3 more than once per year on average over 3 years. An exceedance day occurs when the 24-hour average PM10 concentration is 155 μg/m3 or higher for a calendar day. The total number of exceedance days in a calendar year is reported for monitoring sites having valid data for 7 out of 12 months in a calendar year. A month is considered valid when there is at least one 24-hour average PM10 concentration.
8. Charting Distribution of Clearest and Haziest Days
Distribution of clearest and haziest days charts on the NPS park conditions & trends web page display the total number of haziest or clearest sample days by month in a calendar year. Distribution information provides insight about the seasonality of clearest and haziest days.
As part of IMPROVE visibility monitoring 24-hour particulate samples are collected every third day. These days are the sampled days. Haziest days are the 20% of sampled days where visibility is most limited and clearest days are the 20% where visibility is most clear. Daily sample data are annually retrieved from the IMPROVE Regional Haze Rule (RHR) summary data page. Daily values are filtered either for clearest (group 10) or haziest (group 90) days. For each filtered group, the count of days is summed within a calendar year.
9. Analysis Methods for Long Trails and Rivers
The National Park Service includes long trail and river units with available air quality information. For longer units, air quality conditions & trends are presented for sections of the trail or river rather than the entire unit. Air quality data summarized for sections are more representative of local air quality than a summary for the entire unit would be. The NPS-ARD selects sections for long trails and rivers based on either park designations, availability of monitoring, and/or ecosystem types. Sections for the Appalachian National Scenic Trail were assigned using the regions found in the Appalachian National Scenic Trail official centerline.
On the conditions & trends web page, air quality values for ozone, visibility, deposition, and particulate matter in each section of the long trail or river is shown as a range. Condition assessments for ozone, visibility, and deposition rely on the maximum estimated values identified in each section as described in Section 2. Condition assessments for particulate matter rely on the maximum monitor-specific value directly reported from available representative monitoring sites for each section of the trail or river. Longer parks often display a wide range of air quality conditions resulting in significantly different maximum and minimum values. The maximum value provides a more conservative context for condition assessment while the minimum value may indicate the degree to which portions of the park have lower levels of air pollution and potentially lower risk. Conditions are available for each section of the trail or river. An overall condition for the entire trail or river is not provided but can be calculated using the methods discussed in 3.7 Overall Air Quality Condition.
Each section for a trail or river may have one or many representative monitoring sites. See Appendix B for list of representative sites. Where available or appropriate, representative monitors for each section are selected to:
- Provide balanced spatial distribution across the trail or river.
- Draw on an extensive monitoring record. Monitoring sites with longer and complete records are preferred over newer and or seasonal monitoring sites.
- Present air quality conditions across varied elevations.
- Present air quality conditions across different population densities. If the park transects different population densities (e.g. urban, suburban, or rural), monitoring sites are selected to represent the transected population densities. If the majority of the each a trail or river section is within lower population densities and high population density areas are only transected for short distances, than monitoring sites in urban areas are less representative and are avoided.
- Minimize the influence of air pollution sources that may not be representative. For example, a potential representative monitoring site is at a local airport. Air quality conditions at that monitoring site, may not be as representative of the park as a monitoring site that is of equal distance but is near a state park. Note that monitoring sites near air pollution sources may be representative of park air quality conditions depending on location of the source and the transport of pollutants.
- Offer opportunities for interpreting air quality data for multiple air pollutants from the same location. Monitoring sites that are co-located with sites measuring different pollutants are given preference.
Data from all available representative monitors are trended over a 10-year period and the entire data record. Within a section, the overall trend for each parameter is available in the summary tabs on the NPS air quality conditions and trends webpage. This is calculated using methods discussed in 4.6 Overall Air Quality Trend. Trend information is available for each section of the trail or river. An overall parameter trend for the entire trail and river is not provided but, can be calculated using the methods discussed in 4.6 Overall Air Quality Trend. The overall trend for all parameter can also be calculated for long units using the methods discussed in 4.6 Overall Air Quality Trend after calculating overall parameter trends, where available.
Confidence is assigned using methods described in Section 5.
Appendix A: Geospatial Estimation Model
Air quality monitoring sites are not evenly distributed across the US. Spatial interpolation is used to estimate air quality measures in non-monitored locations and locations with multiple monitors. NPS-ARD uses Inverse Distance Weighting (IDW) to estimate air quality measures for NPS units within the contiguous US based on available monitoring data.
IDW, as applied by NPS-ARD, uses a weighted average from the 12 closest air quality monitoring sites for a given parameter to estimate air quality conditions for all locations across the contiguous US. The weight given to each monitor is a decreasing function of distance. The monitor’s influence on estimated values diminishes with the inverse distance-squared (i.e., 1/distance2) from locations. The closer a monitoring site is, the more influence, or weight, it has in the averaging process. For example, if a park has an air quality monitoring site within park boundaries, that monitor will have more influence than monitoring sites outside park boundaries. The output is a continuous raster surface with a 2.5 km resolution covering the contiguous US. For individual parks, the minimum and maximum values within park boundaries are reported from this national analysis. For parks with surface area less than 12.5 square kilometers (i.e. less than two grid cells), the centroid value is reported from this analysis.
Uncertainty in spatial interpolation is an unavoidable part of the process. Because inverse distance weighting is a deterministic technique, it does not take into account the arrangement of the monitoring sites. Higher levels of uncertainty are expected for regions with large topographic variability, near urban and industrial areas, and in regions isolated from monitoring sites. For example, air quality estimates in urban areas where monitors are clustered or in rural areas where monitors are sparse may be less representative of the air quality within a park.
Generating air quality estimates using IDW
- Monitoring site data preparation:
- Calculate averages using parameter specific completeness criteria detailed in Section 2
- Eliminate collocated sites by selecting the site with the highest completeness criteria
- Eliminate duplicate sites (e.g. LYBR_RHTS in the IMPROVE network)
- Eliminate urban sites in the NADP and IMPROVE networks
- NADP: CO06, CO11, CO84, CO85, CO86, CO87, KY19, MA22, MA98, MD99, NC41, NJ30, NJ39, NJ99, NY06, NY43, NY92
- IMPROVE: BIRM1, FRES1, PHOE1, PUSO1, ATLA1, DETR1, PITT1
- Keep Canada sites in the IMPROVE and NADP networks
- Convert calculated averages at monitoring sites to shapefiles (EPSG: 6350 [NAD_1983_2011_Contiguous_USA_Albers])
- Note that in some cases monitoring networks report latitude and longitude values in different Geodetic datums. All datums were standardized to NAD83(2011)
- National Park Service boundaries preparation:
- Import Administrative Boundaries of National Park System Units
- Incorporate boundaries missing from Administrative Boundaries file. The following boundary files were provided by region or park: Harriet Tubman Underground Railroad National Historic Park, Harriet Tubman national Historic Park, Grand Canyon-Parashant National Monument, Blackstone River Valley National Historic Park, and Tule Lake National Monument)
- Merge Sequoia National Park and Kings Canyon National Park into one unit since it is managed as one unit (Sequoia and Kings Canyon National Parks)
- Merge NPS unit boundaries for park units with differing designations which are managed as one unit, such as Denali National Park and Denali National Preserve
- Project to EPSG: 6350 [NAD_1983_2011_Contiguous_USA_Albers
- Isolate contiguous US by removing the following states: AK, AS, GU, HI, MP, PR, VI
- Template preparation:
- Load boundary data into QGIS
- Create new surface layers for parks with polygon area less than 12.5 kilometers and parks with polygon area greater than and equal to 12.5 kilometers
- Use RealCentroid tool in QGIS to calculate centroids for parks with area less than 2 pixels
- Create a buffer file for setting extent and aligning raster layers
- Download TIGER boundary file from the United States Census Bureau.
- Import shapefile into QGIS and projected to EPSG: 6350 [NAD_1983_2011_Contiguous_USA_Albers
- Use dissolve tool in QGIS to dissolved state lines
- Use rasterize tool in QGIS to create a 100 km buffer around the dissolved contiguous border
- Convert vector to raster specifying resolution based on georeferenced units (2500 x 2500) and the desired layer extent
- Visibility air quality estimates generation:
- Load templates into QGIS
- Load 5-year average visibility values (haze index on clearest days, haziest days, and mid-range days in deciviews) and annual visibility values (standard visual range on clearest days and haziest days in miles) into QGIS
- Run v.surf.idw tool using 12 points, power 2, output raster cell size set to 2.5 km, and extent set to match that of the buffer file
- Run SAGA in QGIS to add raster value to points using centroids for parks with less than 2 pixels
- Run zonal statistics tool to derive maximum and minimum values
- Merge zonal statistics output with raster value points output
- Ozone air quality estimates generation:
- Load templates into QGIS
- Load 5-year average ozone concentration values (4th-highest daily maximum 8-hour average ozone concentrations in ppb and 3-month maximum 12-hour W126 index ppm-hrs) into QGIS
- Run v.surf.idw tool using 12 points, power 2, output raster cell size set to 2.5 km, and extent set to match that of the buffer file
- Run System for Automated Geoscientific Analyses (SAGA) in QGIS to add raster value to points using centroids for parks with less than 2 pixels
- Run zonal statistics tool to derive maximum and minimum values
- Merge zonal statistics output with raster value points output
- Wet deposition air quality estimates generation:
- Load templates into QGIS
- Load 5-year average wet concentration values (precipitation-weighted mean concentrations of ammonium, nitrate, sulfate, nitrogen, and sulfur in mg/L) and 3-year average wet concentration values (precipitation-weighted mean concentrations of mercury in ng/L) into QGIS
- Run v.surf.idw tool using 12 points, power 2, output raster cell size set to 2.5 km, and extent set to match that of the buffer file
- Import 30 year annual precipitation normals in millimeters with a 800 meter spatial resolution were downloaded from the PRISM Climate Group into QGIS
- Resample PRISM data to 2500 pixel size and projected the raster to EPSG: 6350 [NAD_1983_2011_Contiguous_USA_Albers
- Align PRISM precipitation and interpolated raster layers to buffer layer
- Use raster calculator to multiply PRISM precipitation data by each of the interpolated wet concentration layers and divide by 100 (or 1000 for mercury) using buffer layer as reference layer
- Run SAGA in QGIS to add raster value to points using centroids for parks with less than 2 pixels
- Run zonal statistics tool to derive maximum and minimum values
- Merge zonal statistics output with raster value points output
- Natural visibility estimates generation
- Load templates into QGIS
- Load natural visibility values (haze index on mid-range days in deciviews and standard visual range on clearest days and haziest days in kilometers) into QGIS
- Run v.surf.idw tool using 12 points, power 2, output raster cell size set to 2.5 km, and extent set to match that of the buffer file
- Run SAGA in QGIS to add raster value to points using centroids for parks with less than 2 pixels
- Run zonal statistics tool to derive mean values
- Merge zonal statistics output with raster value points output
- Convert natural visibility values from kilometers to miles and round to the nearest whole number
Appendix B: List of In-Park and Nearby Representative Monitors
There are over 240 monitors located in national parks and 380 that meet the criteria to be considered representative of park air quality. Our monitoring locations webpage displays these monitors and monitor-specific information on an interactive map. See table with in-park and nearby representative air quality monitors for NPS units.
In-park and representative monitors are used for trends and conditions. Trends are only computed from in-park or nearby representative monitoring data. Conditions are generally not derived using monitor-specific data from in-park and nearby representative monitors and rely on estimated values. However, where available, in-park monitoring data are used for visibility conditions. Also, particulate matter conditions are solely derived using data from in-park and nearby representative monitors.
Ozone
Representative ozone monitors are within 10 km of park boundaries. Monitors operated by NPS take precedence over other nearby monitors. In cases where the park has more than one monitor operated by the NPS, the monitor with the longest monitoring history is selected to represent the park. There are a handful of representative monitors that are no longer the closest monitor within a 10 km radius but are retained as the representative monitor to maintain a consistent historic record of trends.
Visibility
Representative IMPROVE monitoring sites are within +/- 100 feet or 10% of maximum and minimum elevation of the park and at a distance of no more than 150 kilometers. IMPROVE representative monitors are not assigned to parks with an urban land-use category unless the monitor is also within the urban land-use category (e.g. Puget Sound monitoring site [PUSO1] is representative of Klondike Gold Rush – Seattle Unit). Land-use categories were derived from the National Land Cover Database 2011 edition. There are monitoring sites that are classified as in-park but are slightly outside of park boundaries (i.e. within 1 km boundaries).
Wet Deposition
Representative NADP/NTN or NADP/MDN wet deposition monitors are within 16 km of park boundaries. Note that for end years 2009 to 2015, the Gates of the Arctic NP & PRES representative monitor (NADP/NTN ID: AK06) was an exception to the distance criteria because it was located 26 km outside of park boundaries for accessibility reasons.
Particulate Matter
Representative particulate matter monitors are within 10 km of park boundaries. In-park or nearby representative monitoring sites can measure both PM2.5 and PM10 but there are sites that only measure PM2.5 or PM10. In those cases where both PM2.5 and PM10 are not available from one site, parks may have two representative particulate matter monitors where one represents PM2.5 and the other PM10. Note that sometimes supplementary representative monitoring sites monitors both PM2.5 and PM10 but only the missing PM is used from that monitor. If there is not a nearby representative site that could supplement the missing data, the park will only have PM2.5 or PM10. FRM or FEM monitors take precedence over in-park or nearby representative IMPROVE monitors. This is because IMPROVE data are collected only every 3 days resulting in less data for condition assessments. There are a handful of representative monitors that are no longer the closest monitor within a 10 km radius but are retained as the representative monitor to maintain consistent historic trend records.
Appendix C: Acquisition and Calculation of Ozone Statistics
Ozone is monitored across the US through air quality monitoring networks operated by the NPS, Environmental Protection Agency (EPA), states, and others. Aggregated hourly ozone concentration data are acquired from the EPA Air Quality System (AQS) database. Note that prior to 2012, monitoring data for selected sites were obtained from the EPA Clean Air Status and Trends Network (CASTNet) database. NPS-ARD evaluates Ground-level ozone using two statistics (4th Highest Daily maximum 8-hour average ozone concentration and 3-month maximum 12-hour W126 index) that are described in sections below.
4th Highest Daily Max 8-Hour Average Ozone Concentration Calculation Methods
2015 Ozone Standard Methods Used for 2014 and Later End-Years
- For a given ozone monitor, obtain all valid hourly ozone concentration data and calculate daily maximum 8-hour average ozone concentrations for the entire period of data collection within a calendar year using the instructions from 40 CFR Appendix U to Part 50 section 3 “Data Reporting and Data Handling Conventions” (a)-(d):
- Hourly average ozone concentrations shall be reported in parts per million (ppm) to the third decimal place, with additional digits to the right of the third decimal place truncated. Each hour shall be identified using local standard time (LST).
- Moving 8-hour averages shall be computed from the hourly ozone concentration data for each hour of the year and shall be stored in the first, or start, hour of the 8-hour period. An 8-hour average shall be considered valid if at least 6 of the hourly concentrations for the 8-hour period are available. In the event that only 6 or 7 hourly concentrations are available, the 8-hour average shall be computed on the basis of the hours available, using 6 or 7, respectively, as the divisor. In addition, in the event that 5 or fewer hourly concentrations are available, the 8-hour average shall be considered valid if, after substituting zero for the missing hourly concentrations, the resulting 8-hour average is greater than the level of the National Ambient Air Quality Standard (NAAQS), or equivalently, if the sum of the available hourly concentrations is greater than 0.567 ppm. The 8-hour averages shall be reported to three decimal places, with additional digits to the right of the third decimal place truncated. Hourly ozone concentrations that have been approved under § 50.14 as having been affected by exceptional events shall be counted as missing or unavailable in the calculation of 8-hour averages.
- The daily maximum 8-hour average ozone concentration for a given day is the highest of the 17 consecutive 8-hour averages beginning with the 8-hour period from 7:00 a.m. to 3:00 p.m. and ending with the 8-hour period from 11:00 p.m. to 7:00 a.m. the following day (i.e., the 8-hour averages for 7:00 a.m. to 11:00 p.m.). Daily maximum 8- hour average ozone concentrations shall be determined for each day with ambient ozone monitoring data, including days outside the ozone monitoring season if those data are available.
- A daily maximum 8-hour average ozone concentration shall be considered valid if valid 8-hour averages are available for at least 13 of the 17 consecutive 8-hour periods starting from 7:00 a.m. to 11:00 p.m. In addition, in the event that fewer than 13 valid 8-hour averages are available, a daily maximum 8-hour average ozone concentration shall also be considered valid if it is greater than the level of the NAAQS. Hourly ozone concentrations that have been approved under § 50.14 as having been affected by exceptional events shall be included when determining whether these criteria have been met.
- For annual values: Report the fourth highest maximum 8-hour average ozone concentration for each year. Annual 4th highest daily maximum 8-hour average ozone concentrations must meet minimum data completeness requirements in order to be considered valid. This requirement is met if valid daily maximum 8-hour average ozone concentrations are available for at least 75% of the days within the ozone monitoring season in any one calendar year. Note that all available monitoring data from a site are used to calculate daily maximum 8-hour average ozone concentrations. Ozone monitoring seasons are only used to evaluate annual completeness criteria. Also, note that if a monitoring site has multiple co-located instruments (i.e. an AQS ID with multiple Parameter Occurrence Codes [POCs]), use 4th highest daily maximum 8-hour average ozone concentration from the POC that has the highest annual completeness criteria for that year. Report annual concentration values in parts per billion (ppb).
- For a 5-year average: Average annual values from each of the 5 calendar years. End year of the 5-year averaging period is the reporting year. Three of the five annual values must be valid in order to calculate the 5-year average. Round the 5-year average to the nearest tenth decimal (e.g. 68.343 to 68.3 or 60.362 to 60.4).
2008 Ozone Standard Methods Used for 2009–2013 End-Years
- For a given ozone monitor, obtain all valid hourly ozone concentration data and calculate daily maximum 8-hour average ozone concentrations for the entire period of data collection within a calendar year using the instructions from 40 CFR Appendix P to Part 50 section 2.1 “Data Reporting and Handling Conventions”:
- Computing 8-hour averages. Hourly average concentrations shall be reported in parts per million (ppm) to the third decimal place, with additional digits to the right of the third decimal place truncated. Running 8-hour averages shall be computed from the hourly ozone concentration data for each hour of the year and shall be stored in the first, or start, hour of the 8-hour period. An 8-hour average shall be considered valid if at least 75% of the hourly averages for the 8-hour period are available. In the event that only 6 or 7 hourly averages are available, the 8-hour average shall be computed on the basis of the hours available using 6 or 7 as the divisor. 8-hour periods with three or more missing hours shall be considered valid also, if, after substituting one-half the minimum detectable limit for the missing hourly concentrations, the 8-hour average concentration is greater than the level of the standard. The computed 8-hour average ozone concentrations shall be reported to three decimal places (the digits to the right of the third decimal place are truncated, consistent with the data handling procedures for the reported data).
- Daily maximum 8-hour average concentrations. (a) There are 24 possible running 8-hour average ozone concentrations for each calendar day during the ozone monitoring season. The daily maximum 8-hour concentration for a given calendar day is the highest of the 24 possible 8-hour average concentrations computed for that day. This process is repeated, yielding a daily maximum 8-hour average ozone concentration for each calendar day with ambient ozone monitoring data. Because the 8-hour averages are recorded in the start hour, the daily maximum 8-hour concentrations from two consecutive days may have some hourly concentrations in common. Generally, overlapping daily maximum 8-hour averages are not likely, except in those non-urban monitoring locations with less pronounced diurnal variation in hourly concentrations.
- (b) An ozone monitoring day shall be counted as a valid day if valid 8-hour averages are available for at least 75% of possible hours in the day (i.e., at least 18 of the 24 averages). In the event that less than 75% of the 8-hour averages are available, a day shall also be counted as a valid day if the daily maximum 8-hour average concentration for that day is greater than the level of the standard.
- For annual values: Report the fourth highest maximum 8-hour average ozone concentration for each year. Annual 4th highest daily maximum 8-hour average ozone concentrations must meet minimum data completeness requirements in order to be considered valid. This requirement is met if valid daily maximum 8-hour average ozone concentrations are available for at least 75% of the days within the ozone monitoring season in any one calendar year. Note that all available monitoring data from a site are used to calculate daily maximum 8-hour average ozone concentrations. Ozone monitoring seasons are only used to evaluate annual completeness criteria.
- For a 5-year average: Average annual values from each of the 5 calendar years. End year of the 5-year averaging period is the reporting year. Three of the five annual values must be valid in order to calculate the 5-year average. Round the 5-year average to the nearest tenth decimal (e.g. 68.343 to 68.3 or 60.362 to 60.4).
The W126 metric evaluates risk to vegetation health based on exposure. The W126 metric equation uses a sigmoidal function to preferentially weight the higher ozone concentrations that are more likely to cause plant damage. It sums all of the weighted concentrations during daylight hours as this is when the majority of gas exchange occurs between plants and the atmosphere.
Methods for 2014 and Later End-Years
The following steps outline the methodology used to calculate the W126 index for 2014 and later condition and trend assessments.
- For a given ozone monitor, obtain the 12 hourly ozone concentrations (ppm) for the daytime hours spanning from 8 a.m. to 8 p.m. It should be noted that the hourly data are timestamped with the beginning hour; therefore, the hourly data used are actually the 12 hours labeled 8 a.m. to 7 p.m. Retain all decimal digits through the last step.
- Transform hourly concentration values to weighted concentration values using the following formula, where ozone is the hourly ozone concentration in ppm:
- Sum the 12 hourly weighted concentration values for each day to derive the Daily Index (D.I.) value reported in ppm-hr.
- Sum all of the D.I. values for each month to derive monthly values (ppm-hrs). This is the monthly index (M.I) value:
- Determine whether the M.I. values meet the monthly percent completeness criterion by adding the number of hourly values (8am to 8pm) in the month and dividing that sum by the number of possible reporting hours (8am to 8pm) for the month. Multiply by 100. If the result is 50 or greater, then the completeness criterion is met. If the result is less than 50, then the month should be excluded.
- M.I. values with 50 to 100% completeness are adjusted for missing data. Multiply the number of days in the calendar month by 12 and divide by the number of reported hourly values (8am to 8pm) in the month. Multiply the result from step 4 by this ratio. (Note: If the data are 100% complete, the ratio is 1.)
- Sum the M.I. values for every consecutive 3-month period.
- Determine whether the 3-month period meets the 75 percent completeness criterion by adding the number of hourly values (8am to 8pm) in the 3-month period and dividing that sum by the number of possible reporting hours (8am to 8pm) for the 3 months. Multiply by 100. If the result is 75 or greater, then the completeness criterion is met. If the result is less than 75, then the 3-month period should be excluded.
- Determine whether the season meets the 75 percent completeness criterion by adding the number of hourly values (8am to 8pm) in the March to September growing season and dividing that sum by the number of possible reporting hours (8am to 8pm) for the growing season. Multiply by 100. If the result is 60 or greater, then the completeness criterion is met. If the result is less than 60, then the year should be excluded from calculations, i.e. stop at this step.
- For each calendar year, report the highest 3-month sum from March to September. Retain all decimal digits. Also, note that if a monitoring site has multiple co-located instruments (i.e. an AQS ID with multiple Parameter Occurrence Codes [POCs]), use 3-month max from the POC that has the highest annual completeness criteria for that year.
- For annual W126 index values: Round the reported 3-month max for each calendar year to the nearest tenth decimal (e.g. 10.343 to 10.3 or 10.362 to 10.4). Note that all decimal digits are retained until this step. Report annual W126 index values in parts per million-hours (ppm-hrs).
- For a 5-year average: Average non-rounded 3-month maximum values from each of the 5 calendar years (i.e. do not use rounded annual year values from step 11 to derive this 5-year average). End year of the 5-year averaging period is the reporting year. Three of the five annual values must be valid in order to calculate the 5-year average.
Methods for 2000 to 2013 End-Years
The following steps outline the methodology used to calculate the W126 index for 2009–2013 condition and trend assessments.
- For a given ozone monitor, obtain 12 hour concentrations (ppm) from 8 a.m. to 8 p.m. Note that the hourly data are timestamped with the beginning hour. Therefore, the hourly data used are actually the 12 hours labeled 8 a.m. to 7 p.m.
- Convert hourly data in ppb to ppm and truncate all to 3 decimals.
- Transform hourly concentration values to weighted concentration values using the following formula, where ozone is the hourly ozone concentration in ppm:
- Sum the 12 hourly weighted concentration values for each day to derive the Daily Index (D.I.) value reported in ppm-hr.
- Sum all of the D.I. values for each month to derive monthly values (ppm-hrs). This is the monthly index (M.I) value:
- Determine whether the M.I. values meet the 75 percent completeness criterion by adding the number of hourly values (8am to 7pm) in the month and dividing that sum by the number of possible reporting hours (8am to 7pm) for the month. Multiply by 100. If the result is 75 or greater, then the completeness criterion is met. If the result is less than 75, then the month should be excluded.
- M.I. values with 75 to 100% completeness are adjusted for missing data. Multiply the number of days in the calendar month by 12 and divide by the number of reported hourly values (8am to 8pm) in the month. Multiply the result from step 4 by this ratio. (Note: If the data are 100% complete, the ratio is 1.)
- Truncate monthly index to 3 decimals.
- Sum the M.I. values for every consecutive 3-month period.
- For annual W126 index values: Report the highest 3-month sum for each calendar year. Truncate the reported 3-month max for each calendar year to the nearest tenth decimal (e.g. 10.343 to 10.3).
- For a 5-year average: Average truncated 3-month maximum values from each of the 5 calendar years. End year of the 5-year averaging period is the reporting year. Three of the five annual values must be present in order to calculate the 5-year average.
Ozone Monitoring Seasons and Waivers
Although all available monitoring data are used to calculate annual ozone statistics, ozone monitoring seasons are used to determine if the data meet completeness criteria. Ozone monitoring seasons are retrieved from the EPA’s ozone seasons webpage. States can also petition the EPA’s regional office to waive ozone monitoring season requirements for specific monitors. Information about ozone season waivers is also found in the EPA’s ozone seasons webpage. Ozone statistic for end-years starting with 2017 use the 2015 ozone standard monitoring season requirements and waivers.
Prior to the 2017 end-year, 2008 ozone standard monitoring season requirements were used. The data were initially retrieved from the EPA’s ozone seasons webpage. The 2008 ozone standard monitoring season requirements are no longer available online. Note that some ozone monitors received ozone season waivers for the 2008 standard but were not found on the EPA’s ozone seasons webpage. These waivers will not apply to the 2015 ozone standard monitoring season requirements that take effect in 2017. States with existing waivers will need to pursue new waivers through the EPA.
See tables of archived 2008 ozone standard monitoring seasons and monitoring season waivers.
Appendix D: Acquisition and Calculation of Particulate Matter Statistics
Particulate matter is monitored across the US through air quality monitoring networks operated by the NPS, Environmental Protection Agency (EPA), states, and others. Aggregated hourly particulate matter concentration data are acquired from Federal Reference Method (FRM) or Federal Equivalent Method (FEM) monitors in the EPA Air Quality System (AQS) database. In addition, daily particulate matter data from the IMPROVE network are sometimes used when there are no FRM or FEM data available. Either hourly or daily data are used to calculate annual concentration statistics measured in micrograms per cubic meter of air (μg/m3). The methodology for calculating annual concentration values from FRM/FEM and IMPROVE monitors differ (see sections below for more information).
Data from Federal Reference Method (FRM) or Federal Equivalent Method (FEM) monitoring sites
- Download annual concentration by monitor data file for the latest three consecutive end-years from the EPA’s Pre-Generated Data Files. Note that data files with complete data are available in June for the prior year. Calculation methods for annual concentrations are found in 40 CFR Appendix N to Part 50 - Interpretation of the National Ambient Air Quality Standards for PM2.5.
- Select data rows where:
- pollutant standard is either PM2.5 24-hour 2012, PM2.5 Annual 2012, or PM10 24-hour 2006;
- completeness indicator is Y;
- there are no events or events are included; and
- the certification indicator is not equal to "Requested but denied"
- If there are co-located monitoring sites (i.e. an AQS ID with multiple Parameter Occurrence Codes [POCs]), choose the POC with highest observation percent for that year.
- Select annual appropriate statistic for each standard. If pollutant standard is:
- PM2.5 24-hour 2012 then use 98th percentile;
- PM2.5 Annual 2012 then use arithmetic mean; and
- PM10 24-hour 2006 then use 2nd max value.
- Calculate 3-year average for each statistic at monitoring sites with at least 3 years of annual data values.
- Round 3-year average concentrations to the nearest 10th. (i.e. 0.1) microgram.
Data from IMPROVE monitoring sites
- Download the latest Regional Haze Rule IMPROVE daily values dataset.
- Calculate 3-year average of 98th percentile PM2.5 concentrations using the RCFM variable (reconstructed PM2.5):
- For each monitoring site, flag each quarter that meets completeness criteria of having equal to or greater than 74.5% data values.
- For each monitoring site, flag complete years where all quarters meet completeness criteria.
- Within each flagged complete year, sort all the daily values by descending value for each year at each monitoring site.
- Select the nth maximum value depending on number of valid daily values within a year using the table below or the Table I in 40 CFR Appendix N to Part 50 - Interpretation of the National Ambient Air Quality Standards for PM2.5.
Annual number of creditable sample for year
The 98th percentile for year is the nth maximum daily value for the year where n is the listed number
1 to 50
1
51 to 100
2
101 to 150
3
151 to 200
4
201 to 250
5
251 to 300
6
301 to 350
7
351 to 366
8
- Calculate 3-year average at each monitoring sites with at least 3 years of annual data values.
- Round 3-year average concentrations to the nearest 10th. (i.e. 0.1) microgram.
- Calculate 3-year average of annual mean PM2.5 concentrations using the RCFM variable (reconstructed PM2.5):
- For each monitoring site, flag each quarter that meets completeness criteria of having equal to or greater than 74.5% data values.
- For each monitoring site, flag complete years where all quarters meet completeness criteria.
- Within each flagged complete year, calculate arithmetic mean from daily values for each quarter.
- Calculate annual arithmetic mean from quarterly means.
- Calculate 3-year average at each monitoring sites with at least 3 years of annual data values.
- Round 3-year average concentrations to the nearest 10th. (i.e. 0.1) microgram.
- Calculate 3-year average of 2nd max PM10 concentrations using the MT variable (PM10).
- For each monitoring site, flag each quarter that meets completeness criteria of having equal to or greater than 74.5% data values.
- For each monitoring site, flag complete years where all quarters meet completeness criteria.
- Within each flagged complete year, sort all the daily values by descending value for each year at each monitoring site.
- Select 2nd max value.
- Calculate 3-year average at each monitoring sites with at least 3 years of annual data values.
- Round 3-year average concentrations to the nearest 10th. (i.e. 0.1) microgram.
Appendix E: Parks in EPA Designated Nonattainment Areas
Starting with 2014 end-year, nonattainment information has no influence on either the ozone or particulate matter conditions. For information purposes, the park conditions & trends web page indicates if any part of the park falls into an EPA designated nonattainment area for either the 2015 ground-level ozone, fine (PM2.5) particulate matter, or coarse (PM10) particulate matter standards. If 15% or less of a park falls into an EPA designated nonattainment area, then the text will indicate that a “Small portion” of the park falls into a nonattainment area. If greater than 15% and less than 50% of a park falls into an EPA designated nonattainment area, then the text will indicate that “Part” of the park falls into a nonattainment area. If greater than 50% of the park falls into an EPA designated nonattainment area, then the text will simply state the park’s name.
EPA nonattainment area designation shapefiles are obtained from the Green Book GIS Download web page and are compared to the NPS units boundaries.
For end-years 2009–2013, the human health ozone condition was adjusted based on nonattainment designation. If an NPS unit was located within an area designated by the EPA as nonattainment for the ground-level ozone standard the ozone condition was adjusted to the Poor condition. For end-years 2009–2013, the overall air quality condition was also adjusted to the Poor condition when an NPS unit fell within an EPA designated nonattainment area for either the 2008 ground-level ozone, fine (PM2.5) particulate matter, or coarse (PM10) particulate matter standards. Note: Great Smoky Mountains NP was initially designated nonattainment for the 2008 ozone primary health standard but was re-designated as attainment in 2015. The ozone condition was adjusted from Fair to Poor for 2013 end-year only.
See table with NPS units in EPA designated nonattainment areas for ozone and particulate matter standards.
Appendix F: Natural Visibility
Natural visibility reflects the range of visibility conditions expected in the absence of human-caused air pollution. Natural visibility is location specific and varies across the U.S.Natural visibility is estimated using two different methods. For parks with in-park IMPROVE monitoring and Class I parks with assigned IMPROVE monitoring sites, site-specific natural visibility values are calculated using IMPROVE data for mid-range days (group 50) following methods in the Guidance for Estimating Natural Visibility Conditions Under the Regional Haze Rule (EPA 2003), updated in 2007 (Copeland et al., 2007). Data were downloaded from the IMPROVE Regional Haze Rule (RHR) summary data page. For all other parks in the contiguous US, natural visibility values are interpolated using the IDW estimation method. The estimated natural visibility value (group 50) for an individual park is the average value within a park’s boundaries derived from this national analysis.
The NPS park conditions & trends web page also displays a range in natural visibility using standard visual range in miles. The minimum value (shortest distance) of the range is the natural visibility on haziest days (group 90) and the maximum value (longest distance) is natural visibility on clearest days (group 10). As above, Class I parks with assigned IMPROVE monitoring sites and parks with in-park IMPROVE monitoring use site-specific natural visibility values calculated following methods in the in the Guidance for Estimating Natural Visibility Conditions Under the Regional Haze Rule (EPA 2003). Data were downloaded from the IMPROVE Regional Haze Rule (RHR) summary data page. For all other parks in the contiguous US, natural visibility values in standard visual range on clearest and haziest days are interpolated using the IDW estimation method. The estimated natural visibility on clearest and haziest days for individual parks are the average values within park boundaries derived from this national analysis. For the NPS park conditions & trends web page standard visual range information is converted from kilometers to miles and rounded to the nearest whole number.
Trend charts on the NPS park conditions & trends web page display the IMPROVE monitoring site-specific estimated natural visibility haze index on haziest days (group 90), clearest days (group 10), and mid-range days (group 50).
See table with natural visibility for NPS units.
Appendix G: Nitrogen and Sulfur Deposition Condition Adjustments
Park nitrogen or sulfur condition may be adjusted based on results from national assessment reports that identified ecosystems and resources at risk to acidification and excess nitrogen enrichment in national parks. These reports provide a relative risk assessment of acidification and nutrient enrichment impacts from atmospheric nitrogen and sulfur deposition for parks with identified natural resources in 32 inventory & monitoring networks.
Ecosystem sensitivity ratings to acidification from atmospheric deposition were based on percent sensitive vegetation types, number of high-elevation lakes, length of low-order streams, length of high-elevation streams, average slope, and acid-sensitive areas within the park (Sullivan et al. 2011a). Ecosystem sensitivity ratings to nutrient enrichment effects were based on percent sensitive vegetation types and number of high-elevation lakes within the park (Sullivan et al. 2011b).
If park ecosystems are ranked Very High in sensitivity to acidification or nutrient enrichment effects from atmospheric deposition relative to all inventory & monitoring parks, the condition category is adjusted to the next most conservative condition category. This adjustment does not apply to parks that already have a Poor condition based on wet deposition levels.
See table with NPS units with “very high” ecosystem sensitivity ranking for acidification or nutrient enrichment impacts.
References
Source: Data Store Collection 7813. To search for additional information, visit the Data Store.
Tags
- air quality
- air pollution
- national park service
- air resources division
- ard
- nps
- methods
- statistical analysis
- conditions
- trends
- status
- visibility
- particulate matter
- methylmercury
- mercury
- toxics
- wet deposition
- nitrogen
- sulfur
- ozone
- w126
- human health
- vegetation health
- ecosystem impacts
- acidification
- nutrient enrichment