Part of a series of articles titled Park Paleontology News - Vol. 14, No. 1, Spring 2022.
Article
The Hagerman Paleontology, Environments, and Tephrochronology (PET) Project Expands its Scope
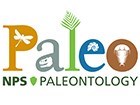
Kari A. Prassack, PhD
Archaeologist, Glen Canyon National Recreation Area/Rainbow Bridge National Monument
Former Senior Paleontologist, Hagerman Fossil Beds National Monument
Co-Lead, Hagerman Paleontology, Environments, and Tephrochronology Project
Hagerman Fossil Beds National Monument. The name probably doesn’t ring any bells, unless you are a paleontologist, but this understated series of brown bluffs abutting the Snake River of southern Idaho preserves an impressive record of life from the Pliocene Epoch. The Pliocene was an exciting time when a mix of animals whose descendants are familiar today, like horses, beavers, ducks, and peccaries, roamed alongside now-extinct beasts like saber toothed cats, giant ground sloths, and mastodons. These and nearly 200 other species of animals are preserved here. Hagerman is most famous for its large accumulation of early single-toed horses but is also known for several species first described from here (referred to as that species’ holotype), including some that are rare or otherwise unknown from elsewhere in the world (e.g., Bjork, 1979; Prassack, 2016).
Having a list of some 200 species is impressive, but those species range in age from 3.07 Ma to as old as 4.8 Ma and did not all co-exist. A species list also cannot tell us much about Hagerman’s environment and animal community, including how they both changed over that nearly 1.73 million years of time. To do that, we must first know the geologic context of the Hagerman Fossil Beds. Luckily, such work is the focus of the Hagerman Paleontology, Environments, and Tephrochronology (PET) Project.
A Paleontologist Dreams of Africa
Prior to working at Hagerman, I was part of an interdisciplinary team working at Olduvai Gorge, in Tanzania, called the Olduvai Landscape Archaeology and Paleoanthropology (OLAPP) Project. The focus of this and other ongoing projects there is to reconstruct the ancient environments used by Pleistocene hominins and to understand what they were doing in different environments. Topography and dates of sites were determined using a complex landscape model based on volcanic ash deposits (Stanistreet, 2012; McHenry and Stanistreet 2018), the identification of post-and synsedimentary faulting events (Stollhofen and Stanistreet, 2012), and the effects of lake cyclicity and river rerouting (Stanistreet et al. 2020). Paleoenvironmental and archaeological indicators were added to recreate how these ancient landscapes may have looked (e.g., Njau and Blumeschine, 2006; Bamford et al. 2006; Blumenschine et al. 2012a, b; Bennett et al. 2012; Prassack 2010, 2014; Uno et al. 2018). This allowed for the study of geologically short periods of deposition: a few thousand years of time termed “living floors” or “paleolandscapes” rather than the longer, time-averaged deposits more typically considered when studying the past.
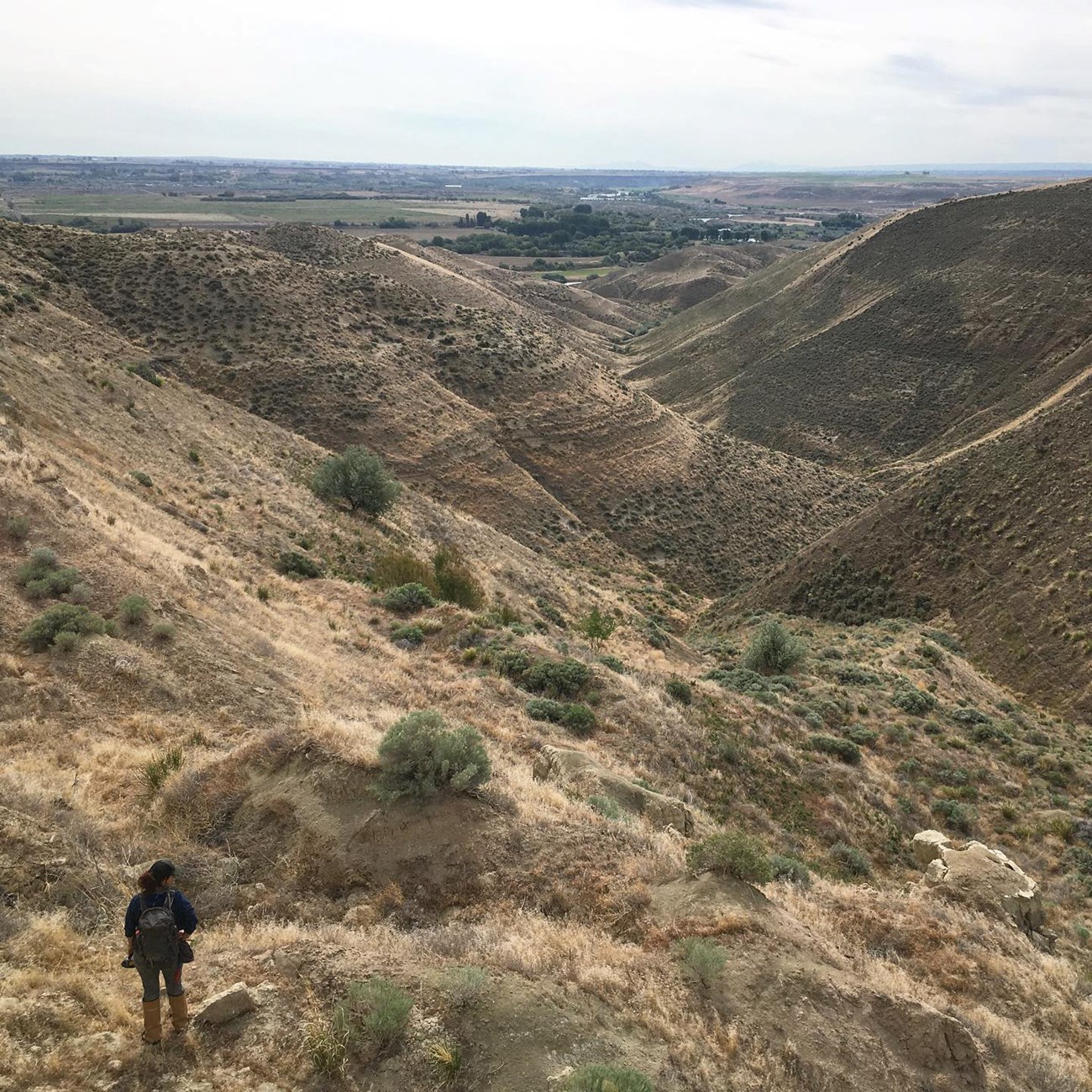
As I looked out at the Hagerman bluffs for the first time (Fig. 1), I saw many parallels to Olduvai. An ancient lake and river would have likely created the type of “landscape mosaic” of lake, wetland, grassland, and open woodland settings often inferred for African sites, but with little known about how these settings were distributed, how they changed over time with changes in climate, hydrology, and tectonic activity, or what effect such changes may have had on the faunal communities that lived there. There was a diverse mix of ancient and familiar species and a rather reasonable slice of time to work with (back then thought to be a mere 500,000 years). Finally, there were numerous volcanic ashes that could provide us with relative dates to separate out these bluffs into a series of time slices. There was opportunity to not just reconstruct a singular time-averaged paleocommunity, but a chance to look at how the faunal composition here changed over time. Most pieces to Hagerman’s puzzle were missing, and those we did have didn’t quite piece together, but Hagerman had potential for understanding animal behavior, community-level structure, and climate change during the Pliocene. The idea for the Hagerman Paleontology, Environments, and Tephrochronology Project was born.
The PET Project Team
- Kari A. Prassack, PhD (Hagerman Fossil Beds National Monument)
- Laura C. Walkup, MS (USGS Tephrochronology Project)
- Elmira Wan, MS (USGS Tephrochronology Project, emeritus)
- William K. Hart, PhD (Miami University, emeritus)
- Mark Stelten, PhD (USGS Argon Geochronology Laboratory)
- Margaret Avery, PhD (USGS Rocks and Paleomagnetics Laboratory)
- Anthony Pivarunas, PhD (USGS Rocks and Paleomagnetics Laboratory)
- Lydia Staisch, PhD, (USGS Geology, Energy, Minerals and Geophysics Science Center)
- Scott Starratt, PhD, (USGS Geology, Minerals, Energy, and Geophysics Science Center)
A Piece of Cake
Laura C. Walkup, MS (USGS Tephrochronology Project), Elmira Wan, MS (USGS Tephrochronology Project, emeritus), William K. Hart, PhD (Miami University, emeritus)
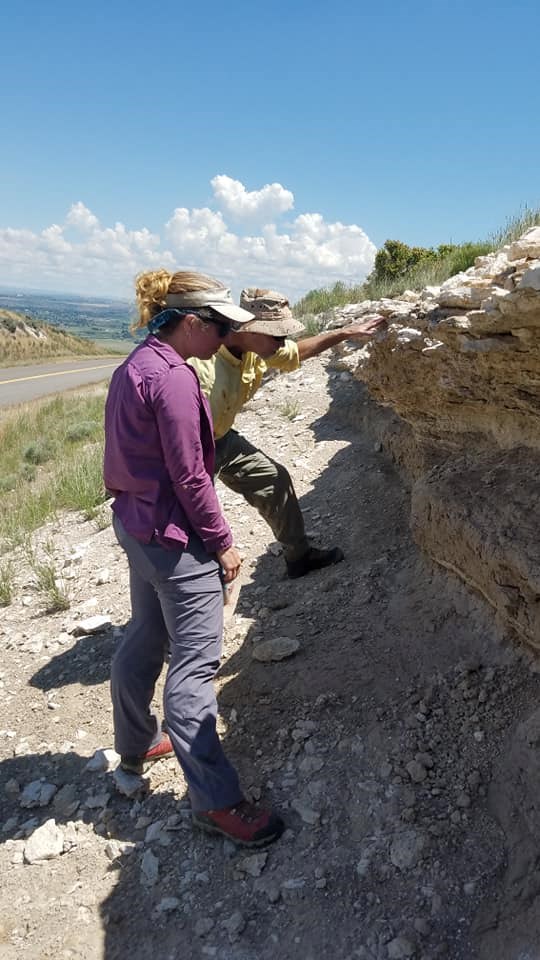
If stratigraphic layers of sediment are akin to a multi-layered cake, then the delicious layers of frosting separating those layers are volcanic ash. Volcanic ashes are important because they are how we date our fossils. The identification and mapping of volcanic ashes at Hagerman began as a short pilot study by Bill Hart and his students in the late 1990s. Fittingly, it was one of Bill’s former students, Laura C. Walkup (Fig. 2), who picked up the reins and took over in 2016. Laura had cut her teeth on the ashes of the Middle Awash of Ethiopia, part of an interdisciplinary project not dissimilar to the one I worked on in Tanzania and was the perfect choice for this project. Laura is easy to find in the field. Her curly blonde hair is often tinged with a bright primary splash of blue or green; but you also just need to look for the sampling sites Laura has dug across the Monument (Fig. 3) in her search for the volcanic ashes that periodically blanketed southern Idaho during the Pliocene.
Volcanic ash, also called tephra, has several attributes that make it useful for establishing chronology. It is made up mostly of volcanic glass, which was liquid magma at the time of the eruption and has a unique chemical fingerprint allowing us to match discontinuous deposits from the same eruption by analyzing the chemical makeup of the glass. This is done using a variety of geochemical techniques including Electron Microprobe Analysis (EMA), Inductively Coupled Plasma Mass Spectrometry (ICP-MS), and Thermal Ionization Mass Spectrometry (TIMS). Volcanic ash also sometimes contains minerals such as feldspars and zircons that were formed in magma chambers and can be directly dated.
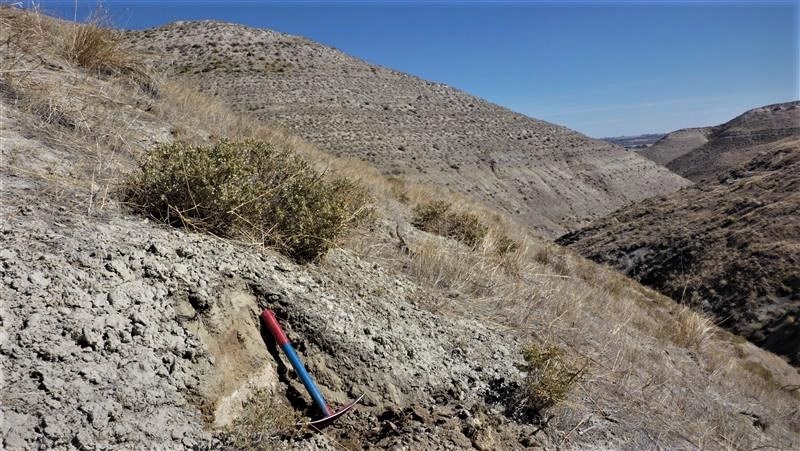
Mapping these ashes across the landscape has allowed for three important things. First, the mapping helped reveal Hagerman’s paleo-topography, which, contrary to past assumptions, was anything but flat. Erosional and depositional events and tectonic activity shaped the land in many ways making precise mapping of these ashes, and the fossil-bearing sediments they sandwich, even more essential. Second, by separating Hagerman’s million years of deposits into a series of more manageable chronologically constrained sections (Fig. 4), we can look at the fossil animals and environments for a given time slice and begin to reconstruct that actual community and landscape. Third, dating of more recent fossils can be done with carbon-14 dating, but the carbon in Hagerman’s fossils has since been replaced by other minerals and thus cannot be directly dated. For older sites like Hagerman, volcanic ashes can instead be dated, providing indirect ages for fossils. This type of analysis is called tephrochronology.
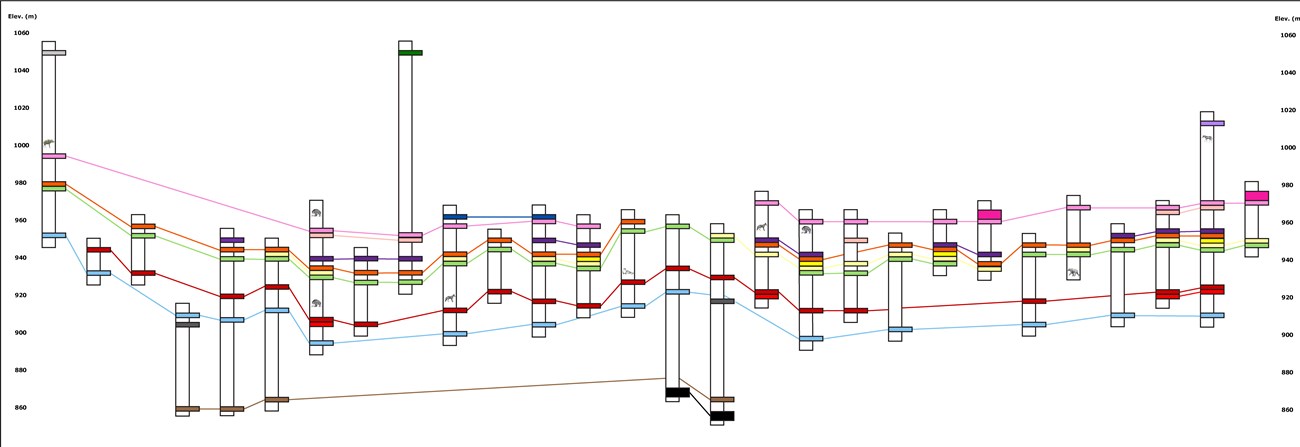
Fig. 4. A fence diagram representing the series of volcanic ashes from distinct volcanic events that were traced and mapped across the landscape by Laura Walkup, USGS. Differences in how these ashes map on the horizontal reflect differences in depositional and erosional rates and in topography, including from tectonic faulting events.
Swiping Right
Mark Stelten, PhD (USGS Argon Geochronology Laboratory) and Laura C. Walkup, MS (USGS Tephrochronology Project)
Tephrochronology allows us to determine both relative and absolute ages of ancient deposits, no matter what the depositional environment or surrounding rock type. This is particularly useful for paleontology because fossils are often found in rock types that cannot otherwise be directly dated. Volcanic ashes can be dated by measuring the rate of decay of argon 40 to argon 39 (40Ar/39Ar) in feldspar in a way not unlike how carbon starts to decay when an animal dies, only these isotopes begin to decay after a volcano spews ash across the landscape.
Hagerman previously relied on two dated ashes. Bed G (represented in orange in Fig. 4), an ash found around midway down most of the slopes, is dated at 3.8 Ma (Hart and Brueseke, 1999) while an isolated occurrence of the Horse Quarry Ash found at the top of the beds is dated to around 3.07 Ma (Hart, unpublished data; lavender ash isolate point in Fig. 4). A maximum date for Hagerman is yet to be determined but is now thought to be as old as 4.8 Ma, expanding the time frame for Hagerman to around 1.7 million years. Laura has processed dozens of ash samples that are now in the hands of Mark Stelton. We expect results in early April.
Flip-Flopping
Margaret Avery, PhD and Anthony Pivarunas, PhD (USGS Rocks and Paleomagnetics Laboratory)
We anxiously await our argon-argon results, but also recognize that accurate dates require the right minerals to be present in the first place and for there to be excellent mineral preservation, so we might get a few duds. But there are plenty of fish in the sea, or in this case dating techniques using rocks, and we have kept our options open.
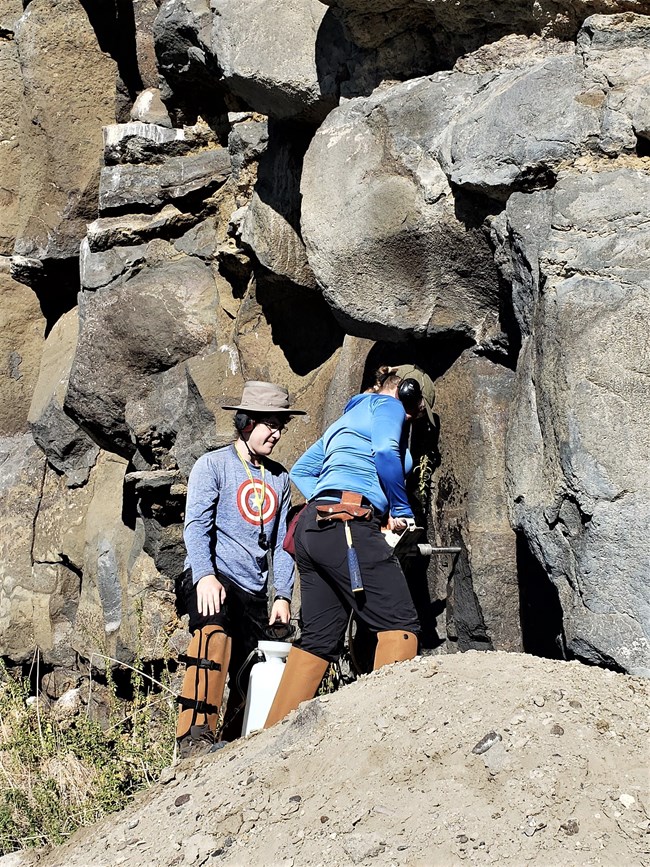
Enter Team Paleomagnetism, who arrived on the scene in 2021, decked out in noise canceling headphones and carrying drills specially designed to bore into the hard basalt rock of past volcanic flows (Fig. 5). Margaret and Anthony are experts in two techniques that can derive geochronological (deep time) data from rock. One is called polar reversal-based dating (paleomagnetism), which is based on our understanding of polar reversals, or essentially what happens when the Earth’s magnetic field flip-flops and the North and South magnetic poles reverse (Avery, 2017; Pivarunas et al. 2021). Volcanic rock is magnetic, which means it records the orientation of the Earth’s magnetic field at the time the lava flow cooled into solid rock, and since these events take several thousand years to complete, you can measure the angle of polarity and map out where in the flip a particular lava flow event occurred, further refining its utility. Hagerman’s deposits occurred during the Gauss normal and Gilbert reverse polarity chrons. While not the kind of precise dates we can get from dating ash, this type of dating can also help us to identify basalt flows on the monument and match them to their sources.
Anthony and Margaret have another, related trick up their sleeves: cyclostratigraphy, which is the study of astronomically forced global-scale changes in sediments that are due to changes in the Earth’s orbit and rotational axis. These changes, which can occur at 40,000- or 100,000-year cycles depending on a variety of factors, are called “Milankovitch Cycles” (Schwarzacher, 1993) and are recorded in the geologic record back at least one billion years. Here, Margaret and Anthony are using cyclostratigraphy to determine how these cycles may have altered the magnetic susceptibility of sediments deposited on the monument. Dating sediments is difficult to do, but this is one way that a general chronology (time sequence) can be hinted at using sediments.
It Also Takes Chemistry
Lydia Staisch, PhD (USGS Geology, Energy, Minerals and Geophysics Science Center)
Volcanic activity can tell us a lot about the timing of events and ages of animals, but not about the environment itself. We know that the Hagerman Beds are part of the Glenns Ferry Formation and consist of fluvial-lacustrine silt, sand, and mud brought in by paleoLake Idaho and the ancestral Snake River and interbedded by layers of volcanic ash. We have long thought that the earliest deposits represented a lower lacustrine plain during a period of lake transgression that was replaced by a warm and wet period with an extensive marshy floodplain, followed by increasing aridity and cooling. This, coupled with what is thought to have been a major lake regression, produced an upper lacustrine plain towards the top of the sequence (McDonald et al., 1996). But that is where our knowledge of the lake ends, and it turns out that things are probably more complicated than that. The oldest deposits at Hagerman may in fact represent deep lake deposits, so we have a lot more to do on that front. Knowing where the lake was is important because lakes have major impacts on the landscape, alternatively scouring out basins as they retreat or depositing sediments as they progress and in shaping what types of floral and faunal communities can be established and thrive across the landscape. We also don’t have the orientation of the ancestral Snake River figured out. There are several techniques that we can apply to these fluvial-lacustrine deposits to better reconstruct where the lake, river, and various other environments were. Some of this will take a lot more work and specialists to reconstruct, while others are being tackled currently.
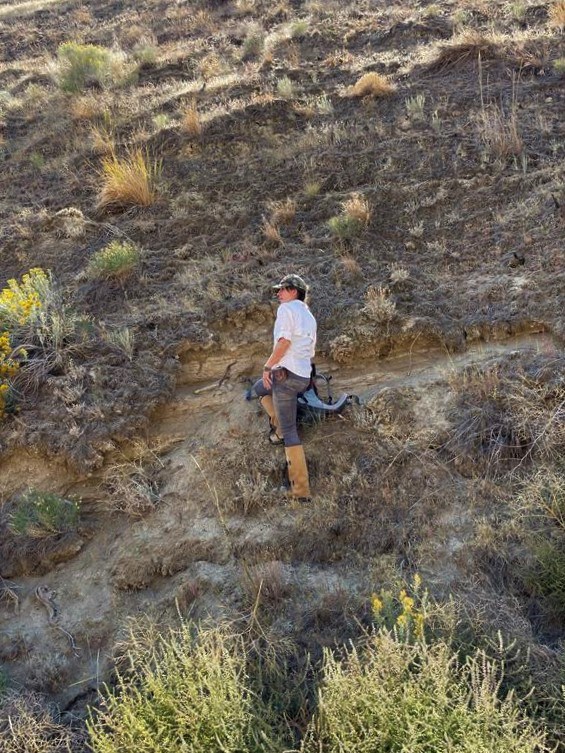
The evolution of a landscape is a function of tectonic, volcanic, climatic, and hydrologic processes. Analyses of stable isotopes and U-Pb of detrital zircons can help to identify the sources of sediments deposited by fluvial processes and the origins of drainage basin shifts that may have been caused by one of those processes. In laymen’s terms, it’s looking for the source of sands and figuring out how they got there, which in turn tells us about how the lake behaved over time. PaleoLake Idaho was once an expansive lake that covered much of western Idaho; its presence here at Hagerman can help us to tie drainage reorganization and related paleoenvironmental proxy data to better understand how these landscapes changed over time, across not only Hagerman’s landscape but all of southern Idaho (Staisch et al. 2021). This is where, in 2021, Lydia Staisch got involved, collecting sand grains that can then be dated, providing an age for when they were deposited.
Lydia also spent much of the field season balanced on steep slopes collecting delicate clam and gastropod (snail) shells (Fig. 6) to analyze for stable isotope geochemistry and clumped isotope geochemistry. These analysis looks at the different atomic numbers of a given element to understand changes in the environment or diet of an animal. Here, she is using the stable isotope differences of oxygen and carbonates in shell to measure the paleotemperature from the time the shell was being formed. This can be used to interpret regional paleoclimate in terrestrial sedimentary environments, such as at Hagerman. This is important because changes in temperature can lead to changes in rates of sedimentation and water temperatures, leading to differences in the types of plants and animals that live in an area.
Size and Shape Matters
Scott Starratt, PhD (USGS Geology, Minerals, Energy, and Geophysics Science Center)
Mastodons and giant ground sloths may capture the imagination of young and old alike, but it’s the smallest of animals that often pack the biggest of punches when it comes to helping us understand the past. Diatoms are single-celled algae that live in shells made of silica (Fig. 7). Primarily aquatic, but with some terrestrial forms, they come in a variety of sizes and shapes related to their specific habitat. This makes them excellent environmental indicators that can reveal water chemistry, depth, and flow. Pick up a handful of clay and it could be chock full of diatoms. You won’t be able to see them without a high-powered microscope, but trust Scott when he tells you they are there. Here at Hagerman, Scott is using diatoms to answer questions about lake depth, wetland distribution, and river flow, including at some of our target sites discussed below.
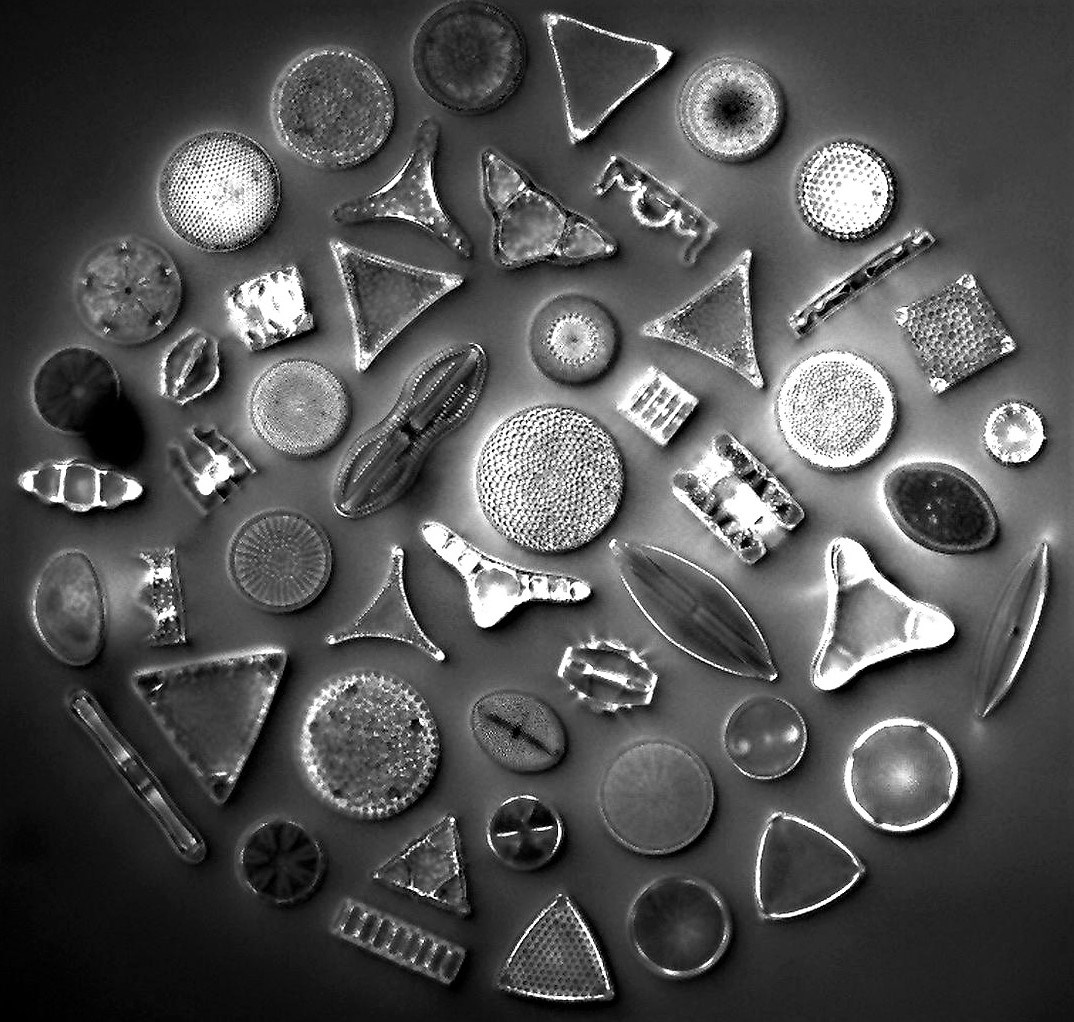
USGS photo.
It’s All About the Bones
Kari A. Prassack, PhD (Hagerman Fossil Beds National Monument)
Hagerman is not the largest of sites, but it encompasses some 1.7 million years spread out across 4,200 acres of steeply sided slopes. For that reason, we initiated this project with the goal of mapping out a series of what were deemed as important sites. These sites are colloquially named Horse, Bear, Otter, Dog, Badger, and Peccary, and we are already able to say a lot more about these sites than before. Horse and Peccary, long thought to be contemporaneous, are not, with the peccary site notably older. The Bear site is confirmed to be at least as old as previously thought (Samuels et al. 2009). The other three, well, let’s discuss them in more detail here.
Otter. In 2016, I had to rely on the now outdated method of projecting an isolated outcrop of Bed G across several bluffs to confirm the antiquity of a new fossil otter, Lontra weiri (Prassack, 2016). This method was flawed in that it assumed that the elevation of a known dated ash could be confidently used to date a similar elevation elsewhere in the park. As is clear by now, it’s an inexact method with a lot of room for error and I had some serious issues in using it, despite it being the method others had used before me. Luckily, when the PET Project later mapped Bed G (Fig. 4) we were able trace it to the exact bluff that the otter was discovered. It was not only above the otter site but even further above than what I had projected. This little otter was being touted as the origin of the genus Lontra here in the Americas, so getting the date right was important.
Dog. That same year, I recognized a robust canid jaw in our collections that was very different from the other, gracile canid jaws we had from a species called Canis ferox, and instead more in line with Canis lepophagus. Canis lepophagus was a larger canid not otherwise recorded at Hagerman and elsewhere was largely found in younger deposits. This would have been a very robust and older example of this species. Recognizing the importance of getting an accurate date, I patiently sat on my find until we were able to map out the bluff from which it was collected. We were able to confirm an age of around 3.9 Ma, making it one of the oldest but also most robust examples of this species (Prassack and Walkup, 2022).
Badger. All the digging around by Laura resulted in some important new finds, including the lower jaw of our enigmatic giant badger, Ferinestrix. Ferinestrix was first described at Hagerman (Ferinestrix vorax, Bjork 1970) and has since only been confidently described from one other locality (Ferinestrix rapax, Wolson and Sotnikova, 2013; Udunga, Chikoi Formation, Western Transbaikal) in Russia. What’s more is that this new jaw was found far down one of our tallest bluffs, conveniently laying atop volcanic ash bed F, giving it a date of between 4.2 Ma and 3.9 Ma which is considerably older than F. vorax, which dates to between 3.4 Ma to 3.0 Ma. We continue to call it by its genus name only, as our analysis thus far suggests it may constitute a new species. We anxiously wait for more precise dates on bed F and the Peters Gulch Ash, either one of which will help us to better refine this specimens age.
Saying Goodbye, For Now. As you may have noted in my title, I have left my role as paleontologist at Hagerman Fossil Beds National Monument to work as an archaeologist at Glen Canyon National Recreation Area. How a paleontologist shifted to a career in archaeology is a story for another day. Importantly, I am continuing my role co-leading my favorite of pet projects, the Hagerman PET project, and while Scott, Margaret, and Anthony are busy with their analysis, Laura and Lydia are getting ready to conduct some work in the similarly aged Ringold Formation of Washington. This work is planned to improve our understanding of the Pliocene in nearby areas. Maybe Laura will stumble upon a red panda, whose fossils are known from that formation; she certainly has a knack for finding carnivoran fossils at Hagerman!
On behalf of the PET team, we look forward to sharing more exciting scientific discoveries as our research continues. Qualified researchers in currently unrepresented fields (e.g., sedimentology, ostracods, plants, and rodents) are encouraged to consider joining us as we continue to unravel the mysteries of the Pliocene at Hagerman Fossil Beds National Monument. Contact Kari Prassack.
References
- Avery, M.S., 2017. Long-Term Geomagnetic Variations: Linking Paleomagnetic Observations, Statistical Analyses, and Numerical Geodynamic Simulations. Dissertation, University of California, San Diego.
- Bamford, M.K., Albert, R.M. and Cabanes, D., 2006. Plio–Pleistocene macroplant fossil remains and phytoliths from Lowermost Bed II in the eastern palaeolake margin of Olduvai Gorge, Tanzania. Quaternary International, 148(1):95–112.
- Bennett, C.E., Marshall, J.D. and Stanistreet, I.G., 2012. Carbonate horizons, paleosols, and lake flooding cycles: Beds I and II of Olduvai Gorge, Tanzania. Journal of Human Evolution, 63(2): 328–341.
- Blumenschine, R.J., Masao, F.T., Stollhofen, H., Stanistreet, I.G., Bamford, M.K., Albert, R.M., Njau, J.K. and Prassack, K.A., 2012. Landscape distribution of Oldowan stone artifact assemblages across the fault compartments of the eastern Olduvai Lake Basin during early lowermost Bed II times. Journal of Human Evolution, 63(2):384–394.
- Blumenschine, R.J., Stanistreet, I.G., Njau, J.K., Bamford, M.K., Masao, F.T., Albert, R.M., Stollhofen, H., Andrews, P., Prassack, K.A., McHenry, L.J. and Fernández-Jalvo, Y., 2012. Environments and hominin activities across the FLK Peninsula during Zinjanthropus times (1.84 Ma), Olduvai Gorge, Tanzania. Journal of Human Evolution, 63(2):364–383.
- Bjork, P.R., 1970. The Carnivora of the Hagerman local fauna (late Pliocene) of southwestern Idaho. Transactions of the American Philosophical Society, 60(7):3–54.
- Hart, W.K. and Brueseke, M.E., 1999. Analysis and dating of volcanic horizons from Hagerman Fossil Beds National Monument and a revised interpretation of eastern Glenns Ferry Formation chronostratigraphy. National Park Service Report, 1443:1–37.
- Liutkus, C.M., Wright, J.D., Ashley, G.M. and Sikes, N.E., 2005. Paleoenvironmental interpretation of lake-margin deposits using δ13C and δ18O results from early Pleistocene carbonate rhizoliths, Olduvai Gorge, Tanzania. Geology, 33(5):377–380.
- McDonald, H.G., Link, P.K. and Lee, D.E., 1996. An overview of the geology and paleontology of the Pliocene Glenns Ferry Formation, Hagerman Fossil Beds National Monument. Northwest Geology, 26:16–45.
- McHenry, L.J. and Stanistreet, I.G., 2018. Tephrochronology of Bed II, Olduvai Gorge, Tanzania, and placement of the Oldowan–Acheulean transition. Journal of Human Evolution, 120:7–18.
- Njau, J.K. and Blumenschine, R.J., 2006. A diagnosis of crocodile feeding traces on larger mammal bone, with fossil examples from the Plio-Pleistocene Olduvai Basin, Tanzania. Journal of Human Evolution, 50(2):142–162.
- Pivarunas, A.F., Meert, J.G., Katusin, K.D., Pandit, M.K., Miller, S.R., Craver, A., Roderus, K.M. and Sinha, A., 2021. Paleomagnetic results from the Singhbhum Craton, India: Remagnetization, demagnetization, and complication. Precambrian Research, 359: 106165.
- Prassack, K.A., 2010. Late Pliocene avifauna from the hominid bearing Zinjanthropus land surface at Olduvai Gorge, Tanzania. Records of the Australian Museum, 62: 185–192.
- Prassack, K.A., 2014. Landscape distribution and ecology of Plio-Pleistocene avifaunal communities from Lowermost Bed II, Olduvai Gorge, Tanzania. Journal of Human Evolution, 70: 1–15.
- Prassack, K.A., 2016. Lontra weiri, sp. nov., a Pliocene River Otter (Mammalia, Carnivora, Mustelidae, Lutrinae) from the Hagerman Fossil Beds (Hagerman Fossil Beds National Monument), Idaho, USA. Journal of Vertebrate Paleontology, 36(4): p.e1149075.
- Prassack, K.A. and Walkup, L.C., 2022. Maybe So, Maybe Not: Canis lepophagus at Hagerman Fossil Beds National Monument, Idaho, USA. Journal of Mammalian Evolution,1–21.
- Samuels, J.X., Meachen-Samuels, J.A. and Gensler, P.A., 2009. The first mid-Blancan occurrence of Agriotherium (Ursidae) in North America: a record from Hagerman fossil beds National Monument, Idaho. Journal of Paleontology, 83(4): 597–603.
- Staisch, L.M., O’Connor, J.E., Cannon, C.M., Holm-Denoma, C., Link, P.K., Lasher, J. and Alexander, J.A., 2021. Major reorganization of the Snake River modulated by passage of the Yellowstone Hotspot. GSA Bulletin.
- Stanistreet, I.G., 2012. Fine resolution of early hominin time, Beds I and II, Olduvai Gorge, Tanzania. Journal of Human Evolution, 63(2): 300–308.
- Stanistreet, I.G., Doyle, C., Hughes, T., Rushworth, E.D., Stollhofen, H., Toth, N., Schick, K. and Njau, J.K., 2020. Changing depocentre environments of Palaeolake Olduvai and carbonates as marker horizons for hiatuses and lake-level extremes. Palaeogeography, Palaeoclimatology, Palaeoecology, 560:110032.
- Stollhofen, H. and Stanistreet, I.G., 2012. Plio-Pleistocene synsedimentary fault compartments, foundation for the eastern Olduvai Basin paleoenvironmental mosaic, Tanzania. Journal of Human Evolution, 63(2): 309–327.
- Schwarzacher, W., 1993. Cyclostratigraphy and the Milankovitch theory. Elsevier.
- Uno, K.T., Rivals, F., Bibi, F., Pante, M., Njau, J. and de la Torre, I., 2018. Large mammal diets and paleoecology across the Oldowan–Acheulean transition at Olduvai Gorge, Tanzania from stable isotope and tooth wear analyses. Journal of Human Evolution, 120: 76–91.
- Wolsan, M. and Sotnikova, M., 2013. Systematics, evolution, and biogeography of the Pliocene stem meline badger Ferinestrix (Carnivora: Mustelidae). Zoological Journal of the Linnean Society, 167(1): 208–226.
Related Links
- Hagerman Fossil Beds National Monument, Idaho—[Geodiversity Atlas] [Park Home] [npshistory.com]
- NPS—Fossils and Paleontology
- NPS—Geology
Last updated: March 31, 2022