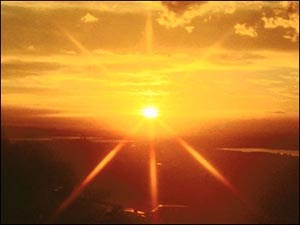
NASA
Learning more about the science of light and human vision will help us understand the value and fragility of natural lightscapes. During the day, the surface of the planet is bathed in light from the sun. The energy in sunlight drives weather, the water cycle, and ecosystems. But at night, in the absence of bright sunlight and clouds, our atmosphere turns transparent and allows us to see beyond our planet into the vastness of the cosmos.
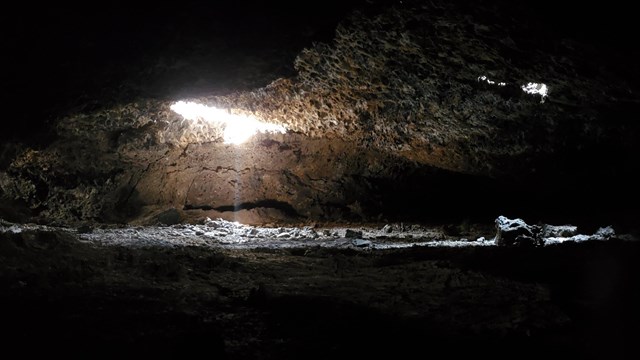
How Light Works
Lava tube at Craters of the Moon National Monument and Preserve, Idaho
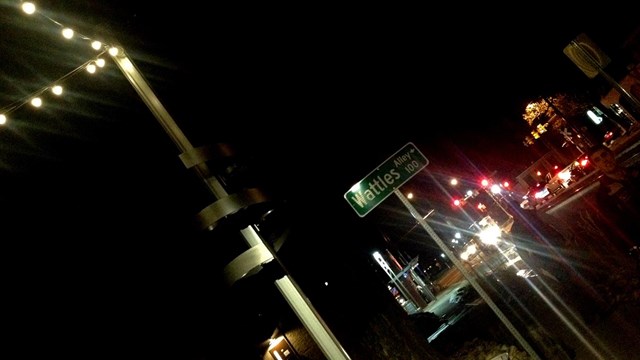
Light Pollution
Click on image above to learn about light pollution.
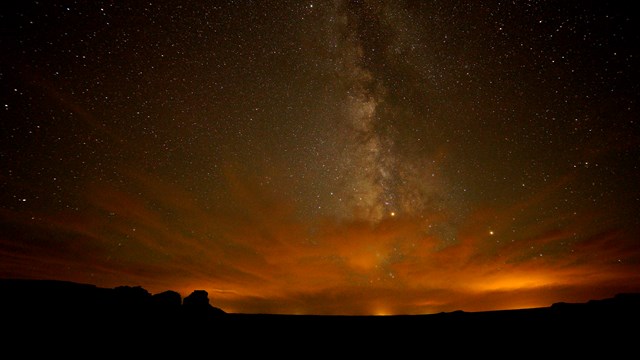
Natural Light in the Night Sky
Natural Light in the Night Sky over Chaco Canyon
Last updated: June 17, 2024