Part of a series of articles titled Yellowstone Science - Volume 27 Issue 1: Vital Signs - Monitoring Yellowstone's Ecosystem Health.
Article
Vital Sign Monitoring is Good Medicine for Parks
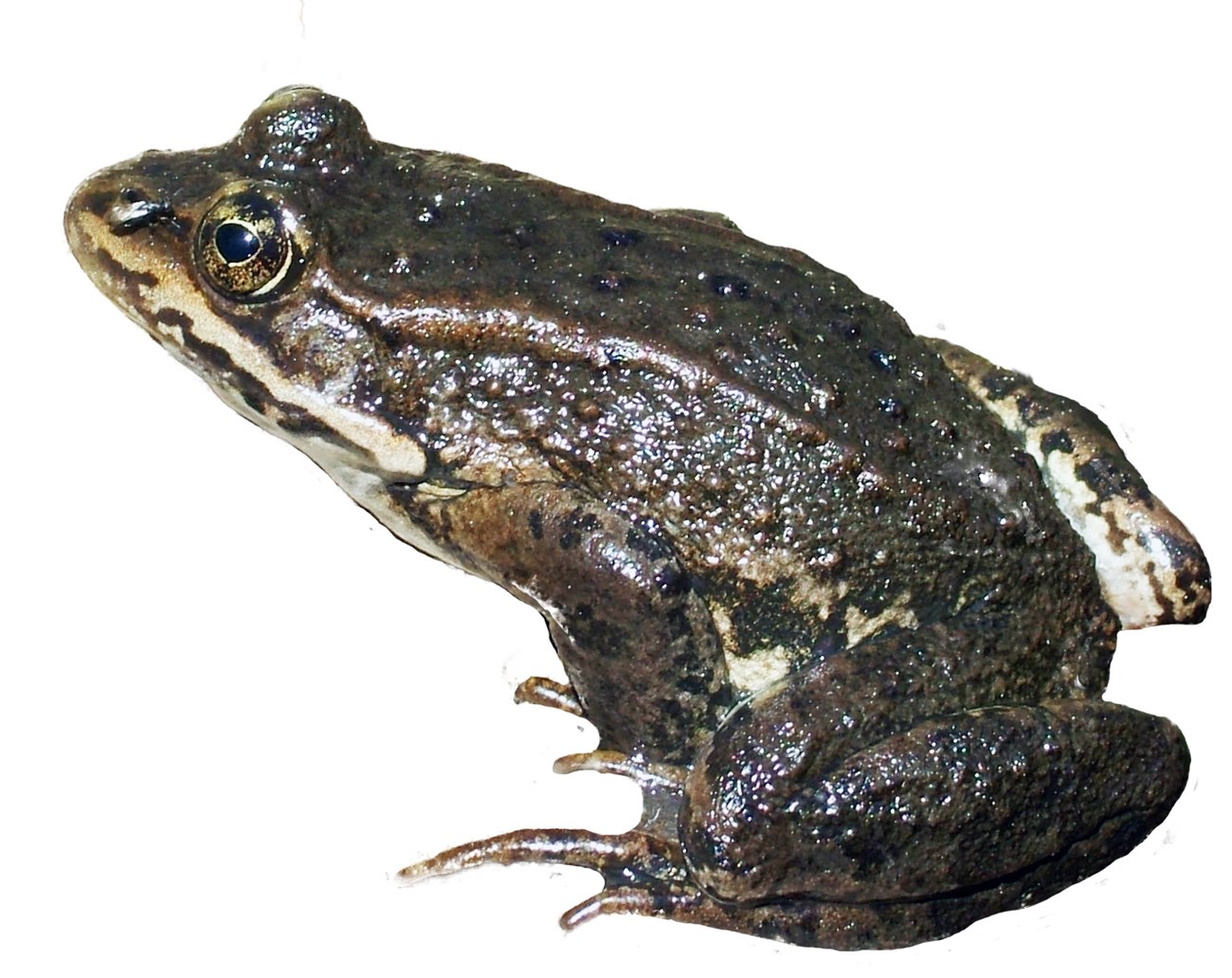
Photo copyright Dave Kazyak
Vital Sign Monitoring is Good Medicine for Parks
by Andrew Ray, David Thoma, Kristin Legg, Robert Diehl, Adam Sepulveda, Mike Tercek, & Robert Al-Chokhachy
Nearly 70 years ago, a young ranger naturalist working in Yellowstone National Park (YNP), Frederick B. Turner, became fascinated with the abundance of frogs next to his cabin at “Soldier Creek” (known as Lodge Creek today). This interest blossomed into Turner’s PhD research and his publication in 1960 about the local population of Columbia spotted frogs (shown to the right) became a classic for herpetologists. Unfortunately the frog population Turner studied had a less positive future in store. Research by Idaho State University biologists in the 1990s revealed that the population had dwindled by 80%. Now, most of Turner’s study area is bereft of spotted frogs, and the remnant population near Yellowstone Lake is affected by a steep decline in reproduction and frequent disease outbreaks. Meanwhile, amphibian population declines documented worldwide, including in national parks of the western U.S., have become common. In YNP and neighboring Grand Teton National Park, we face the important questions of whether widespread amphibian declines are occurring here and if so, what they portend. Can amphibians inform us about the health and changing conditions of wetlands in YNP? What are the implications of diminishing wetlands for other water-dependent species? And, more broadly, can amphibian and other living organisms serve as reliable indicators or vital signs for park health? In this issue of Yellowstone Science, we discuss how ecosystem science is taking cues from the medical field by relying on vital sign monitoring programs to assess ecosystem health.
Medicine is almost universally recognized as the scientific practice of identifying, preventing, and curing disease. The word "medicine" comes from med, meaning thought or meditation in Latin and give attention to or think about in Greek (Charen 1951). Those who practice medicine should, therefore, have sound judgement and the ability to see, think about, and treat patients as a whole while understanding there is natural variation in vital signs among individuals and, to some extent, individualized responses to both disease and treatment. Practitioners must also have humility, a willingness to acknowledge uncertainties, and the capacity to consider and evaluate all of the available evidence of illness in the context of family history and environmental influences.
Ecosystems, like the human body, are complex arrangements of distinct components working together to function properly and respond to outside stimuli. In ecosystem medicine, disease can be considered more broadly as a departure from the normal range of variation and a process which transforms ecosystems to a state where associated health and vitality are diminished (Rapport et al. 1979). As with medicine, a misdiagnosis is possible, but science reduces uncertainty by helping understand how interacting components of complex systems work together. Today, vital human and ecosystem characteristics are monitored using select biological, chemical, and physical indicators. Because of the utility of indicators for monitoring human and ecosystem health, the term "vital signs" has been embraced by both medical and ecological disciplines.
Vital Signs
In medicine, vital signs such as blood pressure, body temperature, and pulse rate are simple routine measurements used to quickly assess health. Although the normal range of human vital signs varies across age groups and ethnicities, when tracked over time vital sign measurements serve as individual baselines or reference points, contribute to diagnoses, and support decisions concerning the response of patients to medical treatments. Slight abnormalities in vital sign measurements (e.g., elevated body temperature) are usually not critical but may warrant additional screening, whereas extremely abnormal vital signs may indicate a life-threatening condition requiring an immediate medical response.
In ecology, vital signs such as snowpack depth, air temperature, and water flows and chemistry serve as similarly simple and routine measures that assess ecosystem health. Because of their value to decision makers, vital signs and broader biological monitoring ("Surrogate Species: Piecing Together the Whole Picture,” this issue) efforts are being widely used to understand and measure the health of plant and animal populations, ecosystems, parks, and even the Earth itself. The overarching goal of vital signs monitoring is to characterize a “safe operating space” or natural or historical range of variation from which future departure can be assessed and, where possible, changes can be mitigated (Röckstrom et al. 2009, Scheffer et al. 2015). The most practical vital sign indicators support management decisions and policies that promote ecological health and track changes in resources that people value (see inset - Supporting Management Decisions which Promote Ecological Health). For example, the U.S. Geologic Survey and U.S. Bureau of Reclamation have monitored river flows and reservoir levels across the Greater Yellowstone Ecosystem (GYE) for decades. Monitoring data have proven valuable for flood prediction and for the allocation and delivery of irrigation water to downstream users but also for informing management strategies that protect native trout and other cold water species. Vital signs monitoring can also contribute to a deeper understanding of past management actions (see inset - Documenting Responses to Past Management Actions) and uncover associations that support forecasting of future conditions (see inset - Forecasting Future Change). As an example, snowpack declines over the last century have been well documented (Pederson et al. 2011). Forecasts for YNP’s snowpacks indicate earlier melting and the continuation of snowmelt declines. While declines are expected to be widespread, regional variation will be considerable (Tercek and Rodman 2016); this understanding will provide decision makers options for minimizing impacts to winter recreation. Finally, vital signs monitoring can serve to document current conditions providing baselines from which future departure can be assessed. For example, scientists in YNP and across the National Park Service (NPS) are actively working to characterize the natural soundscapes of parks (“What We're Listening to: How Sound Inventories Can Contribute to Understanding Change,” this issue). Today, acoustic monitoring techniques are helping park staff understand and fully characterize biodiversity (Buxton et al. 2018), develop noise mitigation programs, and document contemporary conditions (Lynch et al. 2011) from which future change can be evaluated. As parks continue to witness expansion of infrastructure and urbanization on neighboring lands and brace for a future with even more visitors, these baseline datasets and others described in this issue will become increasingly valuable.
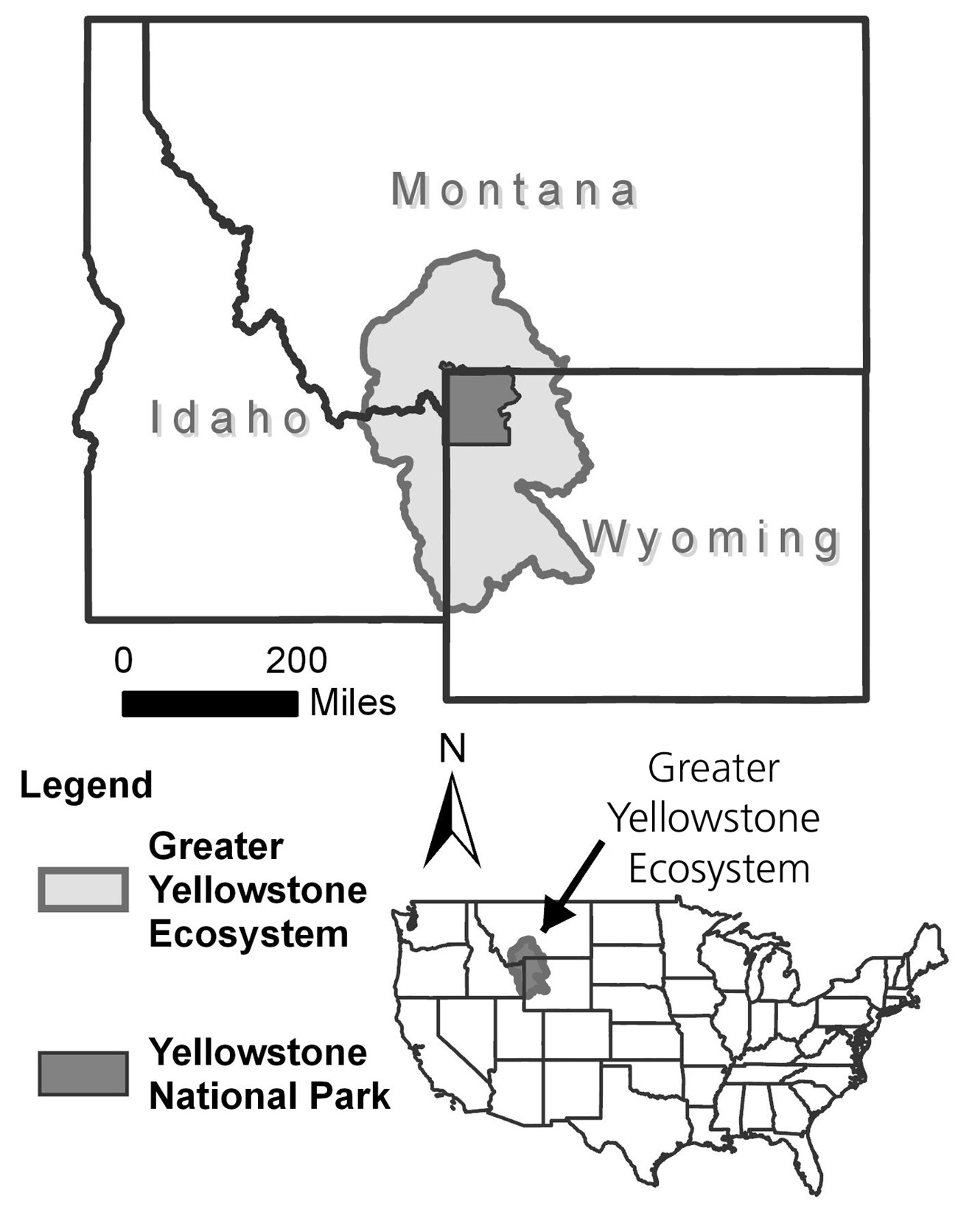
Grizzly bears and whitebark pine are the only non-game biological vital signs monitored across such a large part of the landscape.
Long-term Monitoring at Ecologically Relevant Scales
Long-term vital signs monitoring when conducted at ecologically relevant temporal and spatial scales can help shed light on shifts in the timing (i.e., phenology) of biological processes; changes in composition of biological communities; variations in the abundance, spatial coverage, and duration of annual snowpacks; and fluctuations in a multitude of other important ecological processes. Well-crafted vital signs monitoring programs can also help attribute changes in vital signs or vital sign responses to multiple, potentially interacting changes to climate drivers (e.g., snow, air temperatures) and the addition of other modern stressors (e.g., establishment of invasive species, increased recreational use, and novel diseases). These changes can occur simultaneously or be greatly exacerbated through a single pulse such as the introduction of invasive species (“Yellowstone Convenes Science Information Sharing Panel on Aquatic Invasive Species,” this issue). Although there are many nuances associated with the discussion of monitoring and scale, long-term ecological monitoring at ecosystem or regional levels is increasingly needed for assessing the health and carefully managing large protected areas for the benefit of future generations (Hansen and Phillips 2018, Watson et al. 2018).In particular, broadscale monitoring at ecosystem or regional scales has revealed unexpected findings about the spatial variation of past change and offers discoveries at scales that cannot be manipulated in traditional lab or field experiments (Sagarin and Puchard 2010). As examples, consider how our current understanding of antelope, mule deer, elk, and grizzly bears has been shaped by monitoring animals throughout annual migrations and documenting long distance movement patterns across the GYE. The early studies of grizzly bears documented regular movements outside of park boundaries and helped launch conversations about the need for coordinated monitoring and management of the species at a regional scale (figure 1). Since 1973, federal, state, and tribal scientists have been working collaboratively as the Interagency Grizzly Bear Study Team to monitor grizzly bear dynamics and share information on the distribution and habits of grizzly bears across the region (van Manen et al. 2017). Like grizzly bears, many species move freely across portions of the GYE and similarly benefit from coordinated, long-term monitoring efforts. Monitoring migratory pronghorn, mule deer, and elk (Roth 2018) has contributed to the protection of important summer and winter range as well as the identification of key migratory routes. Similarly, regional monitoring has led to the identification and protection of regional strongholds for native grayling and cutthroat trout (Gresswell 2011), and the long-term conservation of one of the GYE’s most iconic species, whitebark pine, will continue to benefit from coordinated monitoring and boundary-spanning conservation strategies (“An Uncertain Future: the Persistence of Whitebark Pine in the Greater Yellowstone Ecosystem,” this issue).
Today, ecological vital signs monitoring programs are working at park, regional, and national spatial scales (Rodhouse et al. 2016) to employ reliable and standardized measurements to assess whether physical or biological indicators are within a natural range of current or historic variation (Röckstrom et al. 2009) or whether they are nearing boundaries that should not be crossed (Radeloff et al. 2015). In YNP the detection of non-native lake trout in the relatively simple Yellowstone Lake food web represented the crossing of an ecological boundary that warranted an immediate and intense response and one that prevented an ecosystem from “tipping” into an undesirable and unrecoverable condition. Long-term vital signs monitoring can have even greater value than short-term monitoring as it can result in a multi-decadal to multi-century window of scientific observations; observations critically needed to identify key drivers of ecosystem and biological community change that can be subtle or slow to unfold (“Past Warm Periods Provide Vital Benchmarks for Understanding the Future of the Greater Yellowstone Ecosystem” and “The Spatial Footprint and Frequency of Historic Snow Droughts in Yellowstone,” this issue). Taken together, the benefits of long-term vital signs monitoring are numerous; moreover, the scientific and management communities recognize the value of long-term vital signs data sets for informing land management decisions and setting landmark environmental policy (Hughes et al. 2017).
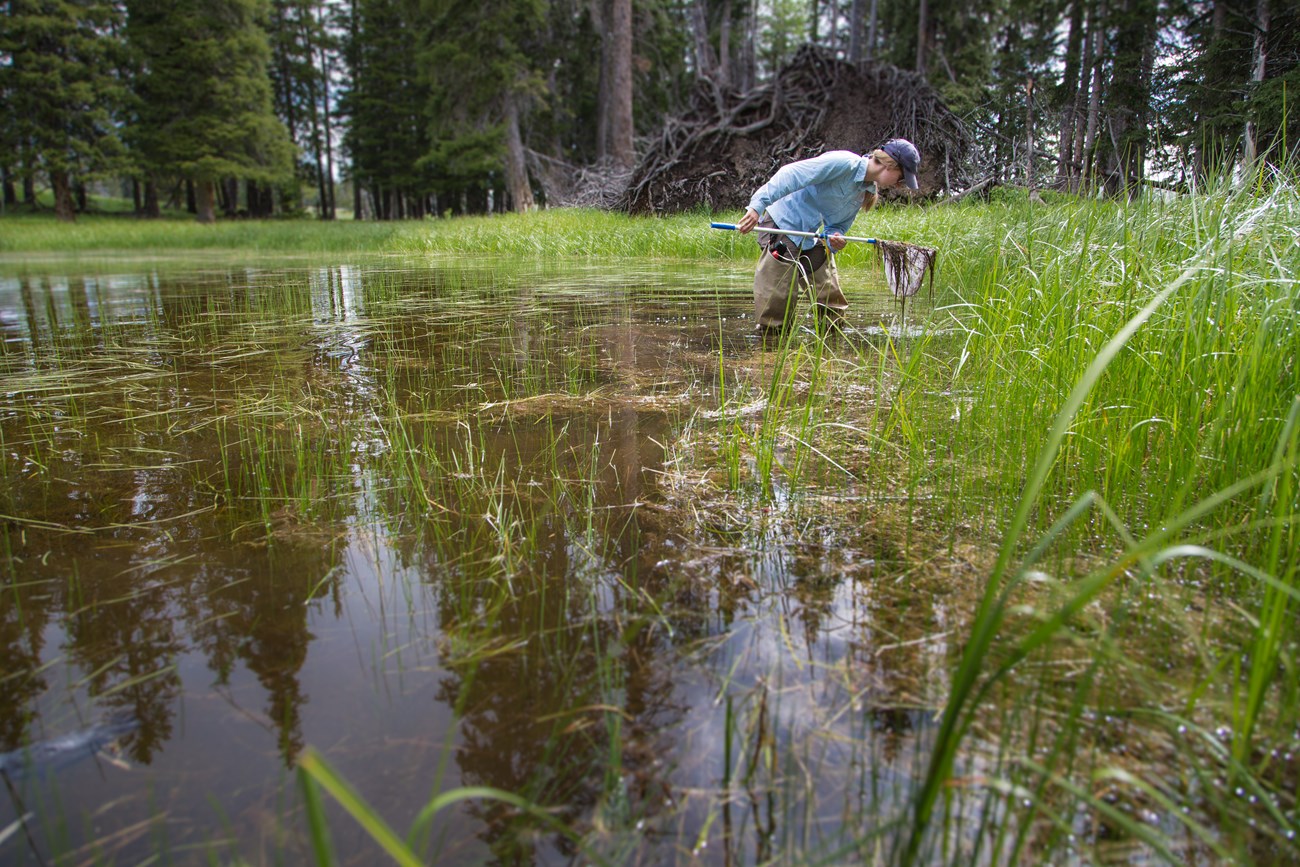
NPS Photo
Vital Signs Monitoring in Yellowstone
In this issue, Legg and Haas (“Understanding Dynamic Ecosystems: the Pursuit of the Greater Yellowstone Network”) describe the Greater Yellowstone Network’s (GRYN’s) Vital Signs Monitoring Program, one of 32 networks monitoring vital signs across the National Park Service. GRYN and other networks monitor vital park resources to document and continually update scientifically sound information on the status and long-term trends of park health (Fancy et al. 2009). Although consistency is a hallmark of monitoring programs, the articles in this issue also communicate why continued flexibility in monitoring approaches is a necessary part of successful long-term monitoring programs. Flexibility, in a monitoring sense, means embracing the use of emerging technological and statistical tools and regularly reevaluating the information needs of current and future park managers (Sergeant et al. 2012). Contributing authors to this issue also identify indicator groups that currently go unmonitored (e.g., insects; “Insects as a Vital Sign in the Greater Yellowstone Ecosystem,” this issue) or are only informally monitored (e.g., bats and aquatic plants; “Yellowstone Bats: an Important Indicator of Ecosystem Health” and “Aquatic Vascular Macrophytes as Vital Signs: Ecological Importance and Management Considerations for the Greater Yellowstone Ecosystem,” this issue) and offer recommendations on establishing specific measurable objectives, share examples of existing protocols, and describe partnership opportunities (“Citizen Science Engagement: a Vital Part of Yellowstone Science,” this issue) that would ensure the long-term success of a vital signs monitoring program for YNP and the region (“Assessing the Ecological Health of the Greater Yellowstone Ecosystem,” this issue).NPS I&M networks and partnering scientists rely on a number of sophisticated (e.g., “Patterns of Primary Production and Ecological Drought in Yellowstone,” this issue) and traditional tools (“Invasive Plants as Indicators of Ecosystem Health” and “Taking the Pulse of Wetlands: What Are We Learning from the Amphibian Vital Sign?,” this issue as examples) and techniques to monitor park vital signs and track ecosystem health. Collectively, these monitoring activities generate reams of data; however, scientists continue to grapple with the most effective ways to rapidly translate these data into information meaningful to resource managers (Schlesinger 2010) and, ultimately, management actions. Having long struggled with ways to translate timely medical data and discoveries from research universities to patients (i.e., from bench to bedside), medical practitioners now follow translational medicine practices. These translational practices focus on the “big picture” and consider the very best ways to leverage large data streams for practical patient-focused applications.
We argue that a similar kind of coordination and vision is needed to leverage the most relevant scientific discoveries and current vital signs data to impact park and ecosystem health by way of coordinated, structured decision-making in parks. Coordinated participation and open dialog between park managers, scientists, and stakeholders is already part of the culture at YNP; this “all hands on deck” approach will be crucial to interpreting change in vital signs, making sense of forecast data, and managing America’s first national park during a period of rapidly accelerating human and climatic pressures (Hansen and Philips 2018). And just as patients can be passive recipients of medicine or medical treatments, patients can also play an active role if lifestyle choices are used to increase the chances of an improved health outcome. Similarly, park managers can be active participants in improving management outcomes through the judicious use of science-based prescriptions for ecosystem health.
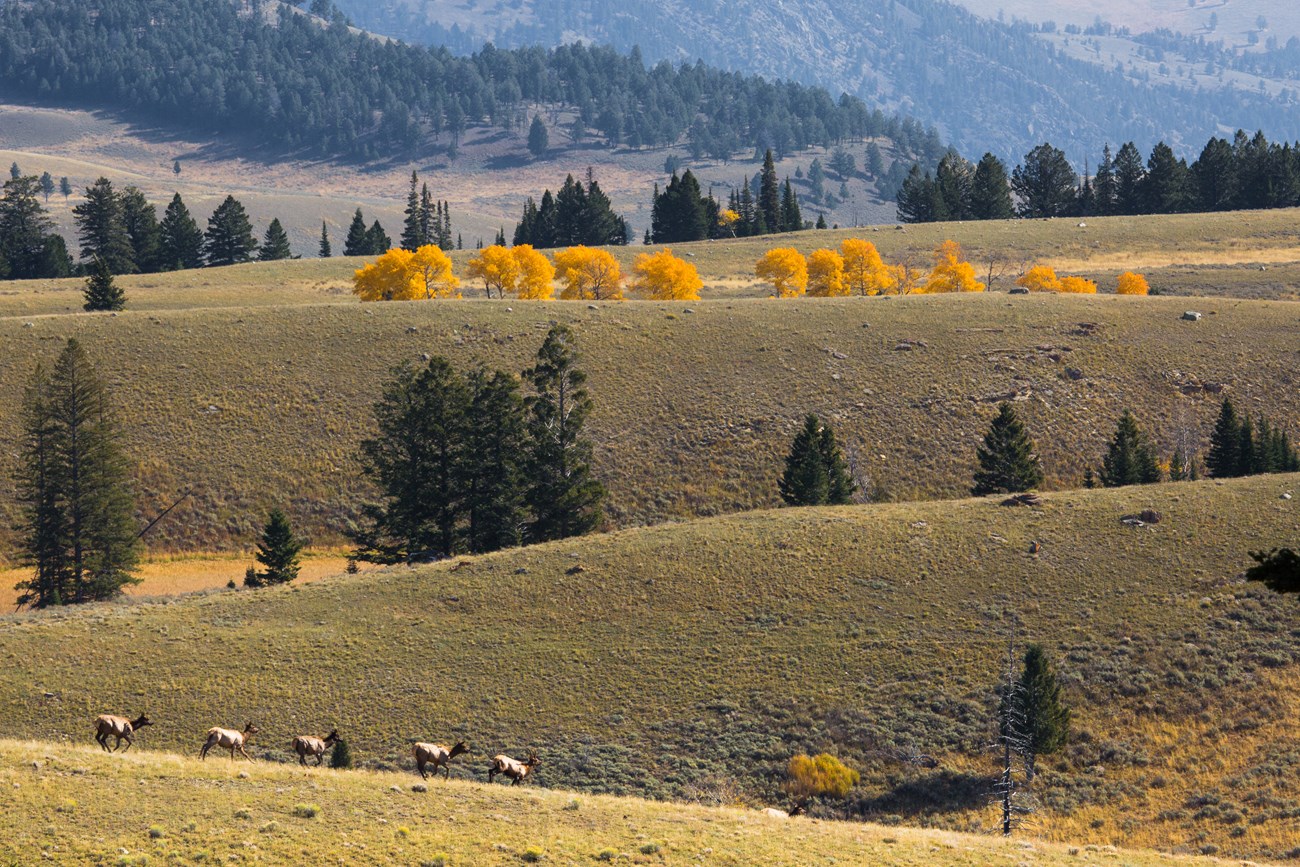
The Vital Sign Special Issue
The articles included in this special issue emphasize why business-as-usual science, separate and apart from long-term monitoring and park decision-making, is no longer sufficient for managing complex park ecosystems through an era of rapid environmental change (National Park System Advisory Board Science Committee 2012). Instead, coordinated monitoring approaches between scientists, stakeholders, and decision makers with clear, mutually-defined goals and sustained financial commitments are needed to support robust and informative long-term vital signs monitoring programs. These vital signs monitoring programs will be key to exploring and describing how modern stressors like exurban development, novel diseases (“The Yellowstone River Fish-Kill: Fish Health Informs and Is Informed by Vital Signs Monitoring,” this issue), the establishment and spread of invasive species, increased recreation, and climate change interact (Enquist et al. 2017). These types of programs are also uniquely positioned to provide a mountain top view of complex ecological conditions for decision makers and serve as underpinnings for a shared and fact-based understanding of ecosystem health, a foundation for establishing sound policies, and a catalyst for partner and public engagement.Scienceless decisions are the fastest way to the impairment of NPS resources.
– Cameron Sholly, Superintendent Yellowstone National Park,
July 17, 2018, Mountain Journal
This issue of Yellowstone Science highlights the importance of vital signs monitoring that informs park condition by putting up-to-date information in appropriate context and offers clear examples of how vital signs data can be marshalled to address some of the most pressing management issues. Never before have we had access to so much information on physical vital signs (e.g., climate, river flow) via technology. Biological data offering historical context, however, is far less common but increasingly necessary for understanding population health, characterizing long-term change, and forecasting future change. Today scientists are explicitly linking physical (e.g., snow, temperatures, and moisture deficit) and biological vital signs. The responses of biological indicators (see Ray et al. 2016, Shanahan et al. 2017; “Patterns of Primary Production and Ecological Drought in Yellowstone,” this issue) define the sensitivity of biological vital signs to past change and support forecasts of impending change.
Although Fredrick Turner’s early studies provided a unique historical backdrop for the evaluation of the spotted frog population at Lodge Creek, we don’t yet know from this isolated study how spotted frogs throughout YNP or the GYE have fared. Follow-up surveys at Lodge Creek in the 1990s documented a dramatic decline of the historic population at Lodge Creek (Patla and Peterson 1999). While follow-up surveys could not undo spotted frog declines, the Lodge Creek case study served as a launch point for annual, parkwide amphibian monitoring efforts and a more comprehensive and scientifically defensible vital signs monitoring campaign for YNP. Modern advances in statistics and modeling support determinations of causation and forecasting at scales that were hardly possible in Turner’s time and without parkwide and regional monitoring efforts. The benefits of these ongoing monitoring efforts are difficult to fully assess today, but this monitoring will serve as an enduring resource and foundation for future, currently unimaginable, science and the basis for data-driven decisions for generations to come.
Literature Cited
Al-Chokhachy, R., A.J. Sepulveda, A.M. Ray, D.P. Thoma, and M.T. Tercek. 2017. Evaluating species-specific changes in hydrologic regimes: an iterative approach for salmonids in the Greater Yellowstone Area (USA). Reviews in Fish Biology and Fisheries 27:425-441.
Buxton, R.T., M.F. McKenna, M. Clapp, E. Meyer, E. Stabenau, L.M. Angeloni, K. Crooks, and G. Wittemyer. 2018. Efficacy of extracting indices from large-scale acoustic recordings to monitor biodiversity. Conservation Biology 32:1174-1184.
Charen, T. 1951. The etymology of medicine. Bulletin of the Medical Library Association 39:216-221.
Enquist, C.A.F., S.T. Jackson, G.M. Garfin, F.W. Davis, L.R. Gerber, J.A. Littell, J.L. Tank, A.J. Terando, T.U. Wall, B. Halpern, J.K. Hiers, T.L. Morelli, E. McNie, N.L. Stephenson, M.A. Williamson, C.A. Woodhouse, L. Yung, M.W. Brunson, K.R. Hall, L.M. Hallett, D.M. Lawson, M.A. Moritz, K. Nydick, A. Pairis, A.J. Ray, C. Regan, H.D. Safford, M.W. Schwartz, and M.R. Shaw. 2017. Foundations of translational ecology. Frontiers in Ecology and the Environment 15:541-550.
Fancy, S.G., J.E. Gross, and S.L. Carter. 2009. Monitoring the condition of natural resources in US National Parks. Environmental Monitoring and Assessment 151:161–174.
French, B. 2016. Fish kill closes 183 miles of Yellowstone River, tributaries to all recreation. Billings Gazette. https://billingsgazette.com/lifestyles/recreation/fish-kill-closes-miles-of-yellowstone-river-tributaries-to-all/article_1a20edf6-6e28-5c62-9566-0c62c343f7dc.html
Gresswell, R.E. 2011. Biology, status, and management of the Yellowstone cutthroat trout. North American Journal of Fisheries Management 31:782-812.
Hamming, C.A. 2016. Yellowstone National Park and the winter use debate: community resilience and tourism impacts in the gateway community of West Yellowstone, MT. Thesis, Montana State University, Bozeman, Montana, USA.
Hansen, A.J., and L. Phillips. 2018. Trends in vital signs for Greater Yellowstone: application of a Wildland Health Index. Ecosphere 9(8):e02380.
Henderson, T., A. Ray, P. Penoyer, A. Rodman, M. Levandowski, A. Yoder, S. Matolyak, M.B. Marks, and A. Coleman. 2017/2018. Mine-tailings reclamation project improves water quality in Yellowstone’s Soda Butte Creek. Park Science 34:9-21.
Hughes, B.B., R. Beas-Luna, A.K. Barner, K. Brewitt, D.R. Brumbaugh, E.B. Cerny-Chipman, S.L. Close, K.E. Coblentz, K.L. de Nesnera, S.T. Drobnitch, J.D. Figurski, B. Focht, M. Friedman, J. Freiwald, K.K. Heady, W.N. Heady, A. Hettinger, A. Johnson, K.A. Karr, B. Mahoney, M.M. Moritsch, A.-M. K. Osterback. J. Reimer, J. Robinson, T. Rohrer, J.M. Rose, M. Sabal, L.M. Segui, C. Shen, J. Sullivan, R. Zuercher, P.T. Raimondi, B.A. Menge, K. Grorud-Colvert, M. Novak, and M.H. Carr. 2017. Long-term studies contribute disproportionately to ecology and policy. BioScience 67:271-281.
Koel, T.M., J.L. Arnold, L.A. Baril, K.A. Gunther, D.W. Smith, J.M. Syslo, and L.M. Tronstad. 2017. Non-native Lake Trout induce cascading changes in the Yellowstone Lake ecosystem. Yellowstone Science 25:42-50.
Lindenmayer, D.B., and G.E. Likens. 2010. The science and application of ecological monitoring. Biological Conservation 143:1317-1328.
Lynch, E., D. Joyce, and K. Fristrup. 2011. An assessment of noise audibility and sound levels in U.S. National Parks. Landscape Ecology 26:1297-1309.
Lovett, G.M., D.A. Burns, C.T. Driscoll, J.C. Jenkins, M.J. Mitchell, L. Rustad, J.B. Shanley, G.E. Likens, and R. Haeuber. 2007. Who needs environmental monitoring? Frontiers in Ecology and he Environment 5:253–260.
NPS. 2017. Yellowstone National Park fishing regulations. https://www.nps.gov/yell/planyourvisit/fishing.htm#fish_regs
National Park System Advisory Board Science Committee. 2012. Revisiting Leopold: resource stewardship in the national parks. National Park Service, Washington, D.C., USA.
Patla, D.A., and C.R. Peterson. 1999. Are amphibians declining in Yellowstone National Park? Yellowstone Science 7:2-11.
Pedersen, G.T., S.T. Gray, C.A. Woodhouse, J.L. Betancourt, D.B. Fagre, J.S. Littell, E. Watson, B.H. Luckman, and L.J. Graumlich. 2011. The unusual nature of recent snowpack declines in the North American Cordillera. Science 333:332-335.
Radeloff, V.C., J.W. Williams, B.L. Bateman, K.D. Burke, S.K. Carter, E.S. Childress, K.J. Cromwell, C. Gratton, A.O. Hasley, B.M. Kraemer, A.W. Latzka, E. Marin-Spiotta, C.D. Meine, S.E. Munoz, T. M. Neeson, A.M. Pidgeon, A.R. Rissman, R.J. Rivera, L.M. Szymanski, and J. Usinowicz. 2015. The rise of novelty in ecosystems. Ecological Applications 25:2051-2068.
Rapport, D.J., C. Thorpe, and H.A. Regier. 1979. Ecosystem medicine. Bulletin of the Ecological Society of America 60:180-182.
Ray, A.M., W. Gould, B. Hossack, A. Sepulveda, D. Thoma , D. Patla, R. Daley, and R. Al-Chokachy. 2016. Influence of climate drivers on extinction and colonization rates of wetland-dependent species. Ecosphere 7(7): e01409.
Röckstrom J., W. Steffen, K. Noone, A. Persson, et al. 2009. Planetary boundaries: exploring the safe operating space for humanity. Ecology and Society 14.
Rodhouse, T.J., C.J. Sergeant, and E.W. Schweiger. 2016. Ecological monitoring and evidence-based decision making in America’s National Parks: highlights of the Special Feature. Ecosphere 7(11):e01608.
Roth, A. 2018. Epic Yellowstone migrations gain new bipartisan protections. National Geographic. https://www.nationalgeographic.com/animals/2018/08/wyoming-yellowstone-pronghorn-migration-news/
Sagarin, R., and A. Pauchard. 2010. Observational approaches in ecology open new ground in a changing world. Frontiers in Ecology and the Environment 8:379-386.
Scheffer, M., S. Barrett, S.R. Carpenter, C. Folke, A.J. Green, M. Holmgren, T.P. Hughes, S. Kosten, I. A. van de Leemput, D.C. Nepstad, E.H. van Nes, E.T.H.M. Peeters, and B. Walker. 2015. Creating a safe operating space for iconic ecosystems. Science 347:1317-1319.
Schlesinger, W.H. 2010. Translational ecology. Science 329:609.
Schook, D.M., and D.J. Cooper. 2014. Climatic and hydrologic processes leading to wetland losses in Yellowstone National Park, USA. Journal of Hydrology 510:340–352.
Sergeant, C.J., B.J. Moynahan, and W.F. Johnson. 2012. Practical advice for implementing long-term ecosystem monitoring. Journal of Applied Ecology 49:969–973.
Shanahan, E., K.M. Irvine, D. Thoma, S. Wilmoth, A. Ray, K. Legg, and H. Shovic. 2017. Whitebark pine mortality related to forest disease, insect outbreak, and water availability. Ecosphere 7(12): e01610.
Tercek, M., and A. Rodman. 2016. Forecasts of 21st century snowpack and implications for snowmobile and snowcoach use in Yellowstone National Park. PLoS ONE 11(7): e0159218.
Turner, F.B. 1960. Population structure and dynamics of the Western Spotted Frog, Rana p. pretiosa Baird & Girard, in Yellowstone Park, Wyoming. Ecological Monographs 30:251-278.
van Manen, F.T., M.A. Haroldson, and B.E. Karabensh, editors. Yellowstone grizzly bear investigations: annual report of the Interagency Grizzly Bear Study Team, 2017. U.S. Geological Survey, Bozeman, Montana, USA.
Watson, J.E.M., O. Venter, J. Lee, K.R. Jones, J.G. Robinson, H.P. Possingham, and J.R. Allan. 2018. Protect the last of the wild. Nature 563:27-30.
Andrew Ray is an Ecologist with the National Park Service’s Greater Yellowstone Network. Andrew has a PhD from Idaho State University, MS from Northern Michigan University, and BS from Purdue University. He works on wetland and water quality monitoring projects in Grand Teton and Yellowstone national parks and Bighorn Canyon National Recreation Area.
David Thoma is a scientist with the National Park Service Inventory and Monitoring Program in Bozeman, MT. He uses a water balance model and satellite remote sensing to understand broad-scale relationships between climate and biology.
Last updated: September 16, 2019