Part of a series of articles titled Yellowstone Science - Volume 27 Issue 1: Vital Signs - Monitoring Yellowstone's Ecosystem Health.
Article
SHORT: Past Warm Periods Provide Vital Benchmarks for Understanding the Future of the Greater Yellowstone Ecosystem
Past Warm Periods Provide Vital Benchmarks for Understanding the Future of the Greater Yellowstone Ecosystem
by Cathy Whitlock & Steve HostetlerThe wildlife, vegetation, and ecosystems discussed in this issue of Yellowstone Science are vital signs of the Greater Yellowstone Ecosystem (GYE) that warrant continued monitoring. Determining which organisms and processes are truly vital ecosystem components requires both an understanding of modern ecological interactions and insight into the resilience of organisms and processes to stressors in the past. The time frame for evaluating past dynamics is often short, but ideally it should be decades or longer in order to span several life cycles of particular organisms or occurrences of a particular process. In a perfect world, we would have information that covers centuries or millennia to assess the consequences of environmental changes that occur over multiple time scales before the influence of recent human activity. Such long-term insights are indeed possible through two approaches. Paleoecological, geological, and archeological data can be used to reconstruct biotic and landscape history. Climate and ecological models help identify the mechanisms linking physical and ecosystem processes in the past and provide simulations of plausible conditions in the future. The challenge with incorporating a paleo-perspective into a discussion of vital signs is that the past is incompletely known and seen through an imperfect lens. Ecological phenomena of interest in the present are often poorly registered over longer time scales.
Future climate projections for the GYE suggest that mean annual temperatures will be 6˚F-13˚F (3˚C-7˚C) higher by the end of the century, and warming will occur throughout the year (figure 1). Winters will be wetter but with a transition to more rain instead of snow, and summers will be drier (figure 1). The changes in temperature and precipitation will have many consequences—earlier snowmelt and peak runoff, shorter springs, less water for streamflow and soils in summer, and greater deficits in evaporative demand, which is indicative of more fires and fire-related erosion. Projected changes in temperature and the hydrologic cycle will alter patterns of animal movement and shift plant distribution and abundance. New organisms that are better adapted to warm conditions and frequent disturbances will move into the region, replacing those that are disturbance sensitive and drought intolerant. High stream temperatures at lower elevations will exceed critical levels for native salmonids leading to their decline, whereas presently cold streams at high elevation may warm sufficiently to support salmonids (Al-Chokhachy et al. 2013).
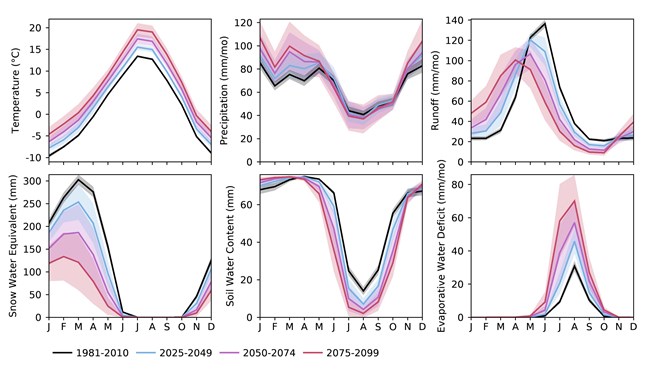
Three periods in the past, characterized by rapid climate change or high temperatures, serve as baselines for evaluating potential climatic and ecological change in the GYE. The first period is the late-glacial to early-Holocene transition (~16,000-11,500 years ago) when temperatures rose about 5˚F-7˚F (from -15˚C to -13˚C) at the end of the last ice age. The difference between warming then and now is that late-glacial warming occurred in summer, and the rate of warming was about 50 times slower than what has been observed in recent history. The second period is the early Holocene (~11,500-7,000 years ago) when summer temperatures were on average 3 to 4˚F (1.6 to 2.2˚C) warmer than present, and winters were colder by a similar amount, as a result of increased summer and decreased winter solar radiation in the Northern Hemisphere. Although warming in early-Holocene summers is a reasonable analog for future conditions in the GYE, the colder winters are not. The third period (800-1,200 years ago) is termed the Medieval Climate Anomaly (MCA). This interval in the GYE was characterized by years to decades of extreme drought. These three benchmark periods represent a set of warm periods by which to assess whether or not current climate conditions and ecosystem dynamics in the GYE are moving into uncharted territory.
Warming at the end of the last ice age melted the Yellowstone ice cap from its full extent, which covered most of the GYE, into remnant alpine glaciers. In response to lengthening growing seasons and developing soils, conifers colonized areas previously covered by ice. The first conifer to appear in the GYE was juniper, probably Juniperus communis, which established in a relatively open tundra-like landscape. Next came Engelmann spruce, followed by whitebark pine, limber pine, and subalpine fir (Krause and Whitlock 2017). Lodgepole pine was widespread after 11,000 years ago, and Douglas-fir was the last conifer to arrive, expanding after 9,000 years ago. This sequence of forest development shows that GYE conifers have the ability to adapt to steadily rising temperatures and changes in moisture by adjusting their range and abundance. Similar biotic responses are taking place now and will continue in the future, although we are starting from a warmer baseline than in the past. Some native species may no longer find suitable climate conditions in the GYE, becoming regionally extirpated as suitable habitat shrinks, and new species (e.g., Gambel oak, western larch, ponderosa pine) may move in if opportunities for dispersal become available (Bartlein et al. 1998). A concern is that non-native weedy species may benefit the most, given their ability to respond rapidly to new environmental conditions.
The early-Holocene warm period was a time of expanded lodgepole pine forest and more Douglas-fir and aspen than at present. Paleoecological records suggest that upper treeline was at a lower elevation and lower treeline was at a higher elevation compared with today, and humans and bison utilized forests that grew above present-day treeline (Craig Lee, CU-Boulder, personal communication, 2018). Many of northern Yellowstone’s small lakes and wetlands dried during the early Holocene, and fires were more frequent. Yellowstone Lake likely had thicker ice cover in winter but also experienced more rapid ice-off in spring and longer open-water conditions in fall (Thompson et al. 1998).
The five-needle pines (whitebark pine and limber pine) show a dynamic history in the GYE during the early Holocene (Iglesias et al. 2015). Following an initial period of tundra and spruce parkland, limber or whitebark pine or both became a significant component of the vegetation at all elevations from 12,000 to 7,000 years ago (figure 2). The expansion of five-needle pines was associated with heightened fire activity, pointing to the ability of these species to withstand drought and frequent burning. These pines became restricted to high and low elevations as summers cooled, winters warmed and became drier, and fires became less frequent and more severe. These paleoecological observations raise concerns that high-elevation whitebark pine forests will continue to decline as warming forces optimal habitat to ever-higher locations (Chang et al. 2014). Although the paleorecord offers evidence of robust adjustments to warmer summers in the past, we have little insight about how five-needle pines will adjust to warmer-than-present winters, a condition that has not occurred during the late-glacial or Holocene periods. This realization alone justifies careful monitoring of whitebark and limber pine populations in the years ahead.
In contrast to the dynamic history of high- and low-elevation forests, lodgepole pine forests on the central rhyolite plateaus have been surprisingly stable during the Holocene, even as climate and fire frequency have varied. This stability is due to the constraints imposed by the relatively infertile well-drained volcanic soils, which tend to limit the presence of other conifers. These lodgepole pine forests developed after 11,000 years ago, replacing a sparse grassland and/or tundra. Once established, these pine forests were unaltered by early-Holocene warm dry conditions or by fires that were 3-4 times more frequent than in recent centuries (Millspaugh et al. 2004). Such resilience suggests that lodgepole pine will persist in the GYE for decades to come, if fires do not become too frequent.
The MCA featured very short springs, and winters transitioned rapidly into summer conditions that were warmer and drier than present. Tree-ring records suggest that drought conditions during the MCA persisted for years to decades in the West (Cook et al. 2004) and led to more fires, desiccation of small lakes, an upslope shift in upper treeline, and stream incision in the GYE (Meyer et al. 1995, Millspaugh et al. 2004,). Vital species, such as beaver, also changed in distribution and abundance (Persico and Meyer 2009). Although it was relatively short in duration, the intensity of drought during the MCA is not unlike conditions projected for the future.
In summary, these benchmark periods clearly show that GYE species have been highly resilient to a range of climate conditions in the past. These species have survived periods when summers were warmer than at present and periods of prolonged and extreme drought. In addition, the vital ecological processes that we monitor today have a long history in the GYE, although their frequency and intensity have varied under different climate conditions. For example, forests have accommodated a range of fire activity in the past, and fire occurrence was several times more frequent than today during warm periods. Although this gives us some confidence in ecological resilience in the future, there are some striking differences between the past and present. We are currently experiencing winters and nights that are warmer than in recent decades. These changes, which are projected to continue, have little historical precedence in the GYE. In contrast, springs that transition abruptly into late-summer drought, as in 2017, have occurred in the past and were likely an enduring feature of the early Holocene and MCA. Similar rapid seasonal transitions are projected for the future (figure 1), and information on past ecological responses to seasonal aridity offers clues as to how future conditions will affect plant and animal phenology, hydrology, and disturbance.
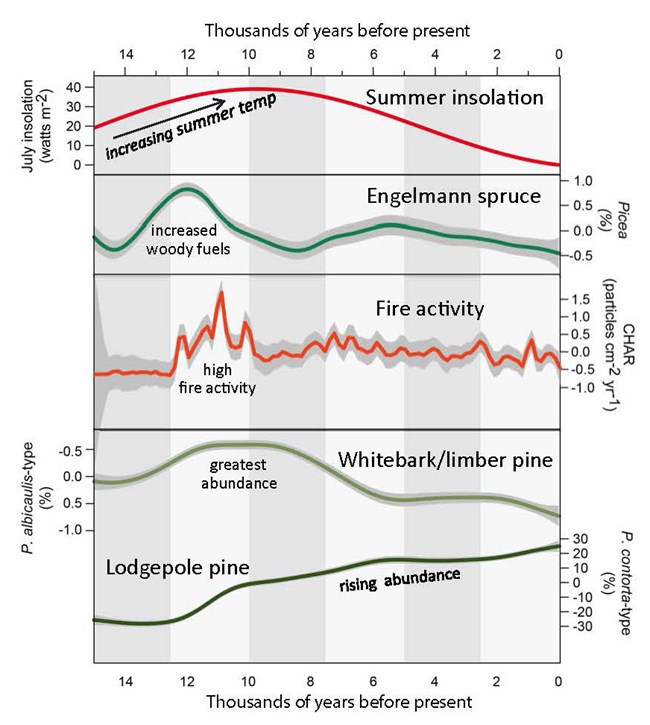
Literature Cited
Al-Chokhachy R., J. Alder, S. Hostetler, R. Gresswell, and B. Shepard. 2013. Thermal controls of Yellowstone cutthroat trout and invasive fishes under climate change. Global Change Biology 19:3069-3081.
Bartlein, P.J., C. Whitlock, and S.L. Shafer. 1998. Future climate in the Yellowstone National Park region and its potential impact on vegetation. Conservation Biology 11:782-792.
Chang, T., A. Hansen, and N. Piekielek. 2014. Patterns and variability of suitable bioclimate habitat for Pinus albicaulis under multiple projected climate models. PLoS ONE 9(11):e111669.
Cook, E.R., C.A. Woodhouse, C.M. Eakin, D.M. Meko, and D.W. Stahler. 2004. Long-term aridity changes in the western United States. Science 306:1015–1018.
Hostetler, S.W., and J.R. Alder. 2016. Implementation and evaluation of a monthly water balance model over the U.S. on an 800 m grid. Water Resources Research 52:9600-9620.
Iglesias, V., T. Krause, and C. Whitlock. 2015. Complex response of Pinus albicaulis to past environmental variability increases understanding of its future vulnerability. PLoS ONE 10(4):e0124439.
Krause, T.R., and C. Whitlock. 2017. Climatic and non-climatic controls shaping early postglacial conifer history in the northern Greater Yellowstone Ecosystem, USA. Journal of Quaternary Science 32:1022-1036.
Meyer, G.A., S.G. Wells, and A.J.T. Jull. 1995. Fire and alluvial chronology in Yellowstone National Park: climatic and intrinsic controls on Holocene geomorphic processes. Geological Society of America Bulletin 107:1211-1230.
Millspaugh, S.H., C. Whitlock, and P.J. Bartlein. 2004. Postglacial fire, vegetation, and climate history of the Yellowstone-Lamar and Central Plateau provinces, Yellowstone National Park. Pages 10-28 in L. Wallace, editor. After the fires: the ecology of change in Yellowstone National Park. Yale University Press, New Haven, Connecticut, USA.
Persico, L., and G. Meyer. 2009. Holocene beaver damming, fluvial geomorphology and climate in Yellowstone National Park. Quaternary Research 71:340-353.
Thompson, R.S., S.W. Hostetler, P.J. Bartlein, and K.H. Anderson. 1998. A strategy for assessing potential future changes in climate, hydrology, and vegetation in the western United States. U.S. Geological Survey Circular 1153. U.S. Government Printing Office, Washington, D.C., USA.
Cathy Whitlock is a Regents Professor of Earth Sciences at Montana State University and a Fellow of the Montana Institute on Ecosystems. She has been studying the vegetation, fire, and climate history of the Greater Yellowstone since 1979.
Steve Hostetler has been a Research Scientist at the USGS since 1987. He investigates the interaction of climate with fire, hydrology, and biota in the West.
Last updated: September 16, 2019