Last updated: March 31, 2022
Article
Potential impacts of projected climate change on vegetation management in Hawai`i Volcanoes National Park
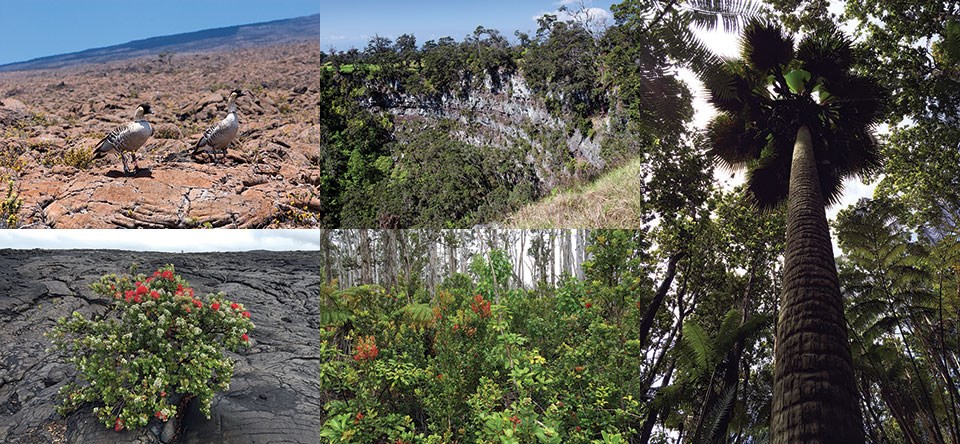
(All) Hawaii Volcanoes National Park/Mark Wasser
Figure 1 (clockwise from top left). Each of the habitat types pictured here was part of the modeling: (A) Lowland dry habitat where native vegetation is projected to contract. (B) Mesic habitat where species richness may decrease by half. (C) Wet habitat with plants that may contract to outside the park. (D) Wet habitat expected to transition to mesic habitat. (E) Dry habitat expected to become mesic and more favorable.
More than 1,000 native plant species exist in the state of Hawai`i, most of which are found nowhere else on Earth. Nearly one-third of these are listed as endangered or threatened by the US Fish and Wildlife Service. Hawai`i is also host to numerous invasive plant species that have spread across the landscape, often excluding natives that evolved in their absence. Because patterns of rainfall and temperature in the Hawaiian Islands are shifting and expected to continue to change into the future, managers at Hawai`i Volcanoes National Park want to know how climate change may shift plant distributions, especially in the park’s highly managed Special Ecological Areas (SEAs), focal sites managed for perpetuating native plant communities and endangered species (Loh et al. 2014) (fig. 1). Under future climate conditions, these protected areas may no longer be suitable for the native species that currently inhabit them. In addition, park managers want to know if expanding invasive species may pose a threat to areas where native plants predominate, thus requiring additional management and resources. To address these concerns, we used bioclimatic envelope models to determine future habitat suitability. Bioclimatic envelope models are based on associations between climate conditions and species’ occurrences to estimate the habitats suitable to maintain viable populations (Araújo and Peterson 2012).
Global climate conditions, including but not limited to increased global temperatures, changing circulation and precipitation patterns, increased ocean acidification, and sea-level rise, are changing (IPCC 2014). These changing conditions result in changes to physical, biological, and human-managed systems. Future climate conditions are projected with global climate models (GCMs) using atmospheric and oceanographic factors. GCMs have a coarse horizontal resolution of 100 km (62 mi) or more. Thus the typical horizontal resolution of GCMs does not adequately represent the small-scale topographic features and climate variation of the Hawaiian Islands (Giambelluca et al. 1986). Accurately characterizing diverse and complex island microclimates requires downscaling of the GCM grid to regional and local scales. Dynamic downscaling is a technique that uses the large-scale conditions provided by the GCMs to drive a local-scale meteorological model with a much higher resolution (Gutmann et al. 2012). The International Pacific Research Center (School of Ocean and Earth Science and Technology, University of Hawai`i at Mānoa) recently completed dynamic downscaling of GCMs for the present day and the end of the 21st century for the Hawaiian Islands (Zhang et al. 2012, 2016a, 2016b).
Changes in climate conditions will drive changes in species distribution, resulting in changes to the composition of plant communities (Price et al. 2012). A recent study by Fortini et al. (2013) examining climate-based species distribution shifts in the context of habitat area, quality, and distribution illustrated that many native Hawaiian plants may be particularly vulnerable to climate change, resulting in major range changes for much of the Hawaiian flora. Furthermore, Foden et al. (2013) and Fortini et al. (2013) have found the Hawaiian plant species most vulnerable to climate change also tend to be those that are most susceptible to existing nonclimatic threats (e.g., competition with or predation by invasive species, habitat loss due to changing land use), suggesting conservation challenges for these species will become increasingly difficult over time.
As a result of climate change and concomitant shifting habitat, resource managers at Hawai`i Volcanoes National Park have considered the need to adjust their current focal conservation areas to ensure that important species and plant communities continue to be protected over time. Intensive vegetation management at the park is focused on Special Ecological Areas (fig. 2), which are roughly configured to protect representative plant communities and important species by controlling the most invasive incipient and established invasive plant and animal species (Loh et al. 2014). Park managers therefore want to know if the current configuration of SEAs will continue to provide protection for focal plant communities and species of concern in the future. Likewise they want to know what new and possibly novel communities may occur in the future within, and adjacent to, the currently configured SEA boundaries. The answers to these questions are likely to suggest revisions to their strategies for future management and protection of the park’s important plant resources.
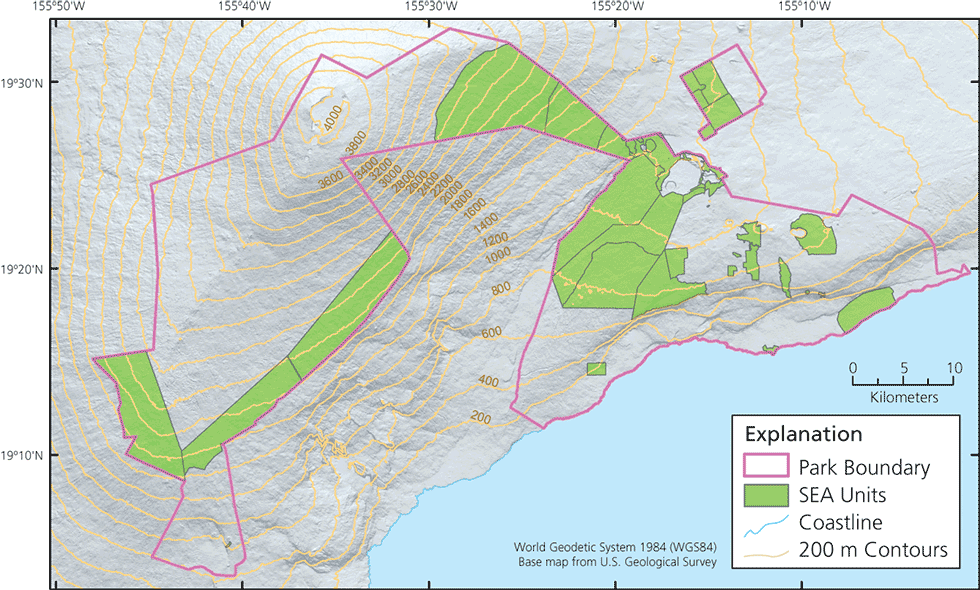
Methods
Figure 3 illustrates the modeling process used to compute species range by combining current (Giambelluca et al. 2013, 2014) and future (Zhang et al. 2012, 2016a, 2016b) climate conditions with records of plant species occurrence from the Price et al. (2012) range models. We modeled the ranges of 29 native and 10 invasive focal plant species statewide, focusing on changes within the national park SEAs (Camp et al. in press). We incorporated bioclimatic factors (volcano boundaries, substrate age, and elevation) from the Price et al. models with current and future projected rainfall and temperature to model species ranges. We obtained rainfall and temperature data from the Rainfall Atlas and Climate of Hawai`i (Giambelluca et al. 2013, 2014) based on the climatological period 1978–2007. For future projections, we used the projected differences in rainfall and temperature between the end-of-century (2080–2099; hereafter 2090) and current (1990–2009) dynamically downscaled climate models from the Hawaii Regional Climate Model (HRCM; Zhang et al. 2012, 2016a, 2016b). Under the A1B emission scenario (balanced energy sources) from the “Special Report on Emissions and the Coupled Model Intercomparison Project Phase 3” (CMIP3) protocol, the HRCM projects moderate warming of 2.5°C (4.5°F) and an increase in precipitation variability across most of Hawai`i by 2090. These climate projections allowed us to model the geographic ranges of plant species in 2090. Following Morrison and Hall (2002) we defined species range as the spatial arrangement of suitable habitats, and species distribution as the subset of range where species actually occur. Our projections therefore represent species range.
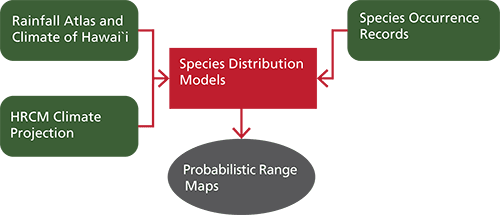
Figure 3. Flowchart depicting bioclimatic envelope model input data (present climate, future climate, and species occurrence records) and processing steps (species distribution models and scaling) used to produce probabilistic range maps. The Hawaii Regional Climate Model (HRCM) is a dynamically downscaled climate model exhibiting a moderately warmer and more variable precipitation future climate scenario. Probabilistic range maps were mapped at 80% threshold generated from 400 model iterations, based on different starting and ending climatic conditions. Round-edged boxes represent data, square-edged boxes represent processing steps or models, and the oval represents output.
We modeled the range of 39 species identified by park resource managers (Rhonda Loh) as influential to the management of ecologically sensitive areas such as Hawai`i Volcanoes National Park SEAs (table 1). Based on the HRCM, we interpolated projected temperature and rainfall for four points in time (beginning-of-century or current [2000], 2040, 2070, and end-of-century [2090]) following a linear trajectory between them.
Table 1. Plant species influential in the development of Hawai`i Volcanoes National Park management strategies for ecologically sensitive Special Ecological Areas | ||||
Scientific Name | Hawaiian/Common Name | % Suitable Park Area | Net % Change | |
Native species | ||||
Acacia koa | Koa | 13 | −13 | |
Alyxia stellata | Maile | 27 | 21 | |
Cheirodendron trigynum | ‘Ōlapa | 3 | −87 | |
Cibotium spp. | Hāpu‘u | 4 | −80 | |
Coprosma ernodeoides | Kūkaenēnē | 51 | −27 | |
Coprosma montana | Mountain pilo | 49 | 16 | |
Coprosma spp. | Pilo | 13 | −48 | |
Dicranopteris linearis | Uluhe | 6 | −87 | |
Diospyros sandwicensis | Lama | 14 | 75 | |
Dodonaea viscosa | ‘A‘ali‘i | 100 | 22 | |
Freycinetia arborea | ‘Ie‘ie | 4 | −77 | |
Ilex anomala | Kāwa‘u | 4 | −82 | |
Leptecophylla tameiameiae | Pukiawe | 66 | −27 | |
Metrosideros polymorpha | ‘Ōhi‘a lehua | 81 | 13 | |
Myoporum sandwicense | Naio | 100 | 16 | |
Myrsine lessertiana | Kōlea lau nui | 6 | −88 | |
Nestegis sandwicensis | Olopua | 15 | 38 | |
Osteomeles anthyllidifolia | ‘Ulei | 75 | 26 | |
Pandanus tectorius | Hala | 17 | 16 | |
Pipturus albidus | Māmaki | 6 | −86 | |
Pisonia spp. | Pāpala kēpau | 10 | −43 | |
Psychotria hawaiiensis | Kōpiko ‘ula | 4 | −79 | |
Psydrax odorata | Alahe‘e | 11 | 28 | |
Rubus hawaiensis | ‘Ākala | 10 | −59 | |
Sadleria cyatheoides | ‘Ama‘u | 47 | −20 | |
Santalum spp. | ‘Iliahi | 99 | 27 | |
Sophora chrysophylla | Māmane | 22 | −4 | |
Vaccinium calycinum | ‘Ōhelo kau lā‘au | 1 | −97 | |
Vaccinium reticulatum | ‘Ōhelo | 51 | −36 | |
Invasive species | ||||
Clidemia hirta | Koster’s curse | 3 | −55 | |
Falcataria mollucana | Albizia | 1 | −78 | |
Hedychium gardnerianum | Kahili ginger | 1 | −93 | |
Lantana camara | Lantana | 13 | 63 | |
Miconia calvescens | Miconia | 1 | −86 | |
Morella faya | Faya tree | 5 | −88 | |
Passiflora tarminiana | Banana poka | 0 | −100 | |
Psidium cattleianum | Strawberry guava | 4 | −72 | |
Rubus ellipticus | Himalayan raspberry | 0 | −100 | |
Schinus terebinthifolius | Christmas berry | 5 | −11 |
Notes: Average percentage suitable habitat in 2090 was calculated as the species-specific suitable habitat (area that remained suitable plus expansion) divided by the area of the park times 100%. Projected net percentage changes in range between 2000 and 2090 were computed as the difference between the 2090 and 2000 percentage of park suitable divided by the 2000 percentage of park suitable times 100% (note differences because of rounding of reported values).
We produced each range model using a series of grids (with a resolution of approximately 250 m [820 ft]), on which we performed logical and mathematical operations. Climate models exhibit a great deal of uncertainty (Lauer et al. 2013) in their predictions, which presents complications when applying them in ecological forecasts. To incorporate uncertainty, we modeled each possible change from 20 years of current climate (1990–2009) to 20 years of end-of-century climate (2080–2099), yielding a total of 400 possible change values over the century. We then used an 80% threshold to map species ranges at each time step (Epstein and Axtell 1996); we considered a pixel (location) to be within the species range if it had an 80% or higher probability of being suitable habitat—that is, if it was suitable in ≥320 of the 400 possible climate projections. Based on projected species ranges, we calculated the change in range between current and future projections.
For the purposes of this study, the 147 SEA management blocks represent too fine a unit for examining changes in species range. Based on discussions with Hawai`i Volcanoes National Park resource managers, we aggregated these management blocks into 37 SEAs representing appropriately sized units for analyzing shifts in species range.
We quantified the net percentage change in species range for the national park and each SEA, and classified the amounts of contraction and expansion as minimal (≤20% change), moderate (20–50% change), or substantial (>50% change). The most desirable levels of change for natives are minimal contraction and substantial expansion, whereas for invasives the reverse is preferred.
We further evaluated each SEA by assessing the change in species richness between the current and end-of-century projections for the 29 modeled native species. Although this group represents only a subset of native species found within Hawai`i Volcanoes National Park, our richness index may be indicative of wider community shifts. We considered SEAs that maintained or increased native richness as optimally situated for future conditions, whereas SEAs that lost more than half of their current native species richness may require further investigation.
Results
Under the moderately warmer and more variable precipitation climate scenario considered, the net percentage change in the 29 native species modeled range is expected to be negative across Hawai`i Volcanoes National Park (table 1) and within the 37 SEAs. In 15 of the SEAs, native species ranges may contract substantially, including the SEAs around the lower portion of Mauna Loa Strip and around Kīlauea Crater, areas that receive intense visitation and also are important for cultural practitioners. In six SEAs, native species contractions were spread among minimal, moderate, and substantial categories. In 14 SEAs (12 occurring below 1,200 m [3,937 ft] elevation), native species contractions were split about evenly between minimal and substantial contractions. Two SEAs showed predominantly minimal contractions.
We projected a negative percentage change for 9 of the 10 invasive species modeled across the national park (table 1) and in all 37 SEAs, except for Lantana camara and Schinus terebinthifolius, which showed mixed results ranging from substantial contractions to substantial expansions in several SEAs. Most invasive species showed minimal expansion under the future climate scenario considered. However, our projected models for the invasive plant species are likely conservative estimates of their potential range since many of these invasive species have yet to reach equilibrium in Hawai`i.
At present, good congruence exists between native species richness and SEA locations (top panel of fig. 4). The congruence, however, was projected to break down over time, and by the end of the century many of the existing SEAs occur in areas with limited habitat suitability for most native species of concern (bottom panel of fig. 4). Of particular interest were the forecasted remnant hotspots on the eastern edge of the Mauna Loa Southwest Rift tract, eastern portion of Olaa tract, and areas south and east of the East Rift tract, as we projected these areas to remain relatively rich (≥ 19 overlapping native species).
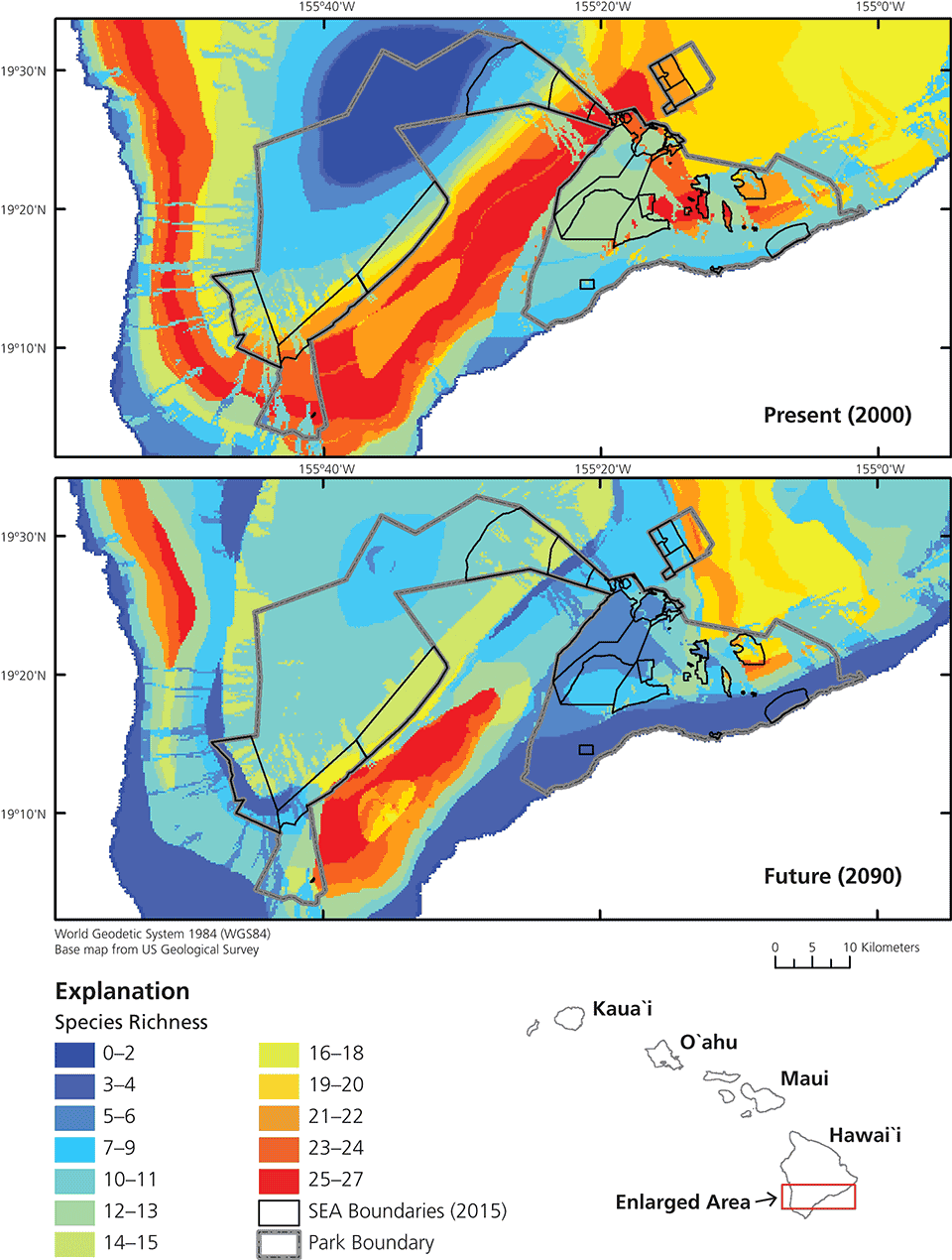
Figure 4. Native species richness (29 modeled species) using cool to warm colors to represent overlapping distributions of few to many species, respectively. The top panel shows that the present (year 2000) distribution of hotspots in the national park aligns well with the distribution of SEAs. As shown in the lower panel, projected species richness at the end of the century (year 2090) predominately recedes from the national park, resulting in few species hotspots within SEA boundaries.
A direct application of our projections is that they can be used to identify locations where major changes in habitat conditions are predicted (e.g., stable wet habitats transitioning to mesic habitats and even to dry habitats) and where plants are projected to respond under future conditions similar to the modeled climate scenario. We addressed this by computing the difference in species richness between the current and end-of-century projections (fig. 4) to generate a map of predicted species turnover (fig. 5). Areas with high species turnover occur where projected climate change effects are strongest.
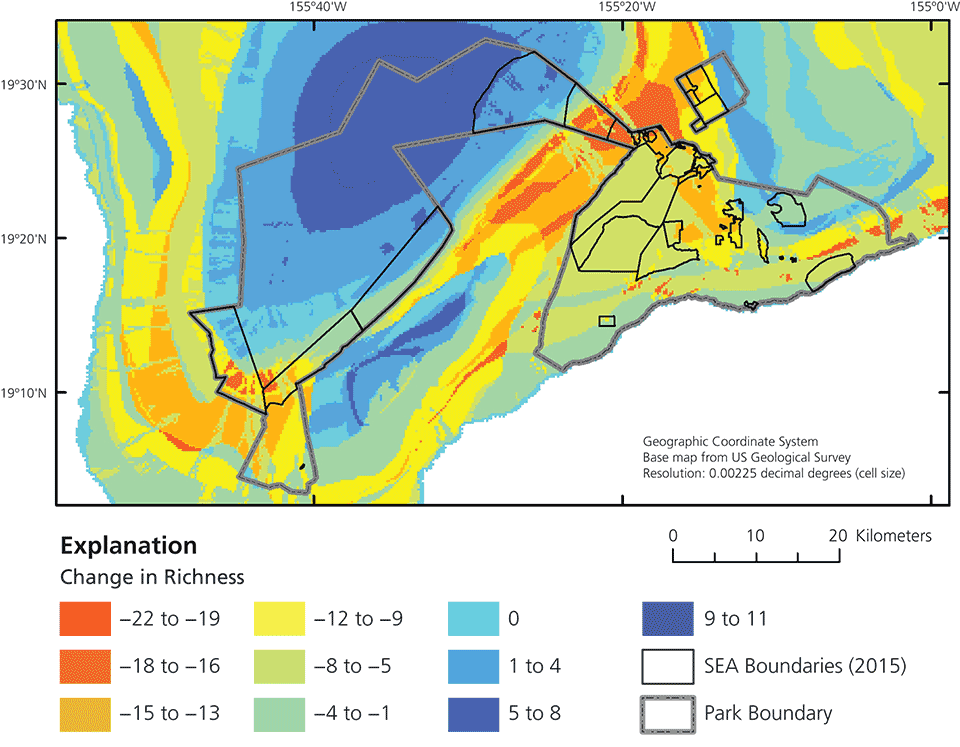
Discussion and management
Climate is a key determinant of species distribution. Geophysically explicit species range modeling offers a powerful option for evaluating plant species response to future climate conditions. Based on relationships of current climate conditions in which a species has been observed, models can be used to predict species responses to forecasted climates (Chen et al. 2011). Forecasted species ranges may be used to focus management on maintaining species where the climate is projected to threaten their existence, as well as to facilitate establishment of SEAs at Hawai`i Volcanoes National Park in areas where species may be expected to shift.
Our end-of-century forecasted species ranges were consistent with other species range modeling for Hawai`i (Price et al. 2012, 2015; Fortini et al. 2013) where species range depends largely on climate (i.e., precipitation and temperature), substrate age, and historical distribution. Assuming an A1B scenario, the HRCM dynamically downscaled end-of-century climate conditions are forecast to have generally warmer temperatures and more variable precipitation. Under this scenario, species range contractions generally are expected to occur in coastal areas and lower elevations, while expansion of suitable conditions is expected to occur primarily in upper-elevation montane and subalpine habitats.
At Hawai`i Volcanoes National Park approximately two-thirds of the modeled native species (18 of 29) were projected to exhibit net range contraction, while about one-third (11 of 29) showed expansion. The species that showed the largest contractions typically have restricted bioclimatic requirements under current conditions. Because of the predominance of range contractions and limited range expansions, we projected a majority of the current SEAs will lose most of the native species we modeled, especially those SEAs occurring below 1,200 m (3,397 ft) elevation. Net range expansion typically occurred for the limited number of species that colonize pioneer, young lava flows where subalpine and alpine environments were projected to become suitable habitat under future precipitation and temperature regimes, i.e., when bioclimatic requirements of the plants and climate change metrics matched (Garcia et al. 2016).
Within the national park, the forecasted amount of range contraction exceeded expansion for all but one modeled invasive species (Lantana camara). These contractions are expected to occur in most SEAs, including SEAs at low, middle, and high elevations. Suitable habitat for only 4 of the 10 invasive species (Clidemia hirta, Lantana camara, Morella faya, and Schinus terebinthifolius) will likely persist throughout most of the park’s SEAs. These results for invasive species may help managers by reducing the need for control measures for this set of invasive species and benefiting some native species by reducing competition. However, any interpretation of range changes for invasive plant species should be made cautiously. The modeled ranges are limited to reported distributions in Hawai`i, and might not reflect the full physiological limits of these species. Additionally, other invasive species besides those modeled in this study are expected to continue to occupy these habitats, and their range may expand or contract in response to climate change (Vorsino et al. 2014).
Forecasted shifts in suitable habitat for native plant species will assist park managers in assessing configuration of and prioritizing future work in SEAs (Watson et al. 2013). Under forecasted end-of-century climate projections where drier areas become drier, wetter areas become wetter, and temperatures increase everywhere, but more so at high elevations (Elison Timm et al. 2011; Zhang et al. 2012, 2016a, 2016b), our results suggest that the congruence between species richness hotspots and SEAs will diminish over time. As such, by the end of the century many projected species hotspots occurred outside of current SEA boundaries.
While the trajectory and extent to which climate change and plant response remain to be validated, managers can reasonably consider expanding existing SEAs or establishing new SEAs in areas where future diversity hotspots are likely to occur. Increasing the size of SEAs, and improving habitat connectivity among them, would better accommodate range shifts of individual species and facilitate their dispersal into more hospitable environments.
Similarly, the results from this modeling can assist national park managers working with adjoining landowners and partner agencies to prioritize conservation work island-wide. Hawai`i Volcanoes National Park collaborates with several state, federal, and private landowners to protect more than a million acres (400,000 ha) of watershed on the island of Hawai`i as a member of the Three Mountain Alliance (TMA). Protection of native species diversity and invasive species management are among the TMA activities that would benefit directly from this modeling and its forecasted shifts in species ranges.
Obtaining additional habitat data in areas projected to experience the greatest climate change, for example deploying weather stations, will help managers understand the trajectory and extent of climate change. In addition, vegetation monitoring will provide information on how plants are responding to these measured changes in conditions.
While current resource management actions (e.g., fencing and control of ungulates, invasive species control, outplanting native plants) will continue to be critical for conservation of plant species and communities, the rate of climate change is an additional factor that will affect habitat suitability. If, for example, climate change is very rapid, the predicted changes in suitable habitat for some native species might grossly underestimate the realized changes in future distribution if these natives cannot adapt rapidly to changing conditions or compete effectively with invasives in order to realize their potential. Assisted colonization, the translocation of organisms outside their historically documented ranges in anticipation of more suitable future conditions, may be a conservation option for consideration. Candidate species, such as Diospyros sandwicensis, Nestegis sandwicensis, and Psydrax odorata, for assisted colonization include those that possess long generation times, have low reproductive rates, lack the dispersal capability needed to track rapidly changing climate conditions, or occur close to their physiological limits (Chauvenet et al. 2013; Rout et al. 2013; Gallagher et al. 2015). Once established, additional management actions such as supplementing introduced populations may be necessary to maintain viable populations and communities (Moir et al. 2012).
The tropics have a relatively small range of natural climate variability (Mora et al. 2013; Power 2014). However, in our models most species’ suitable habitat ranges changed substantially by century’s end. Based on which rate of climate change is being followed as the century unfolds, our projections allow managers to update their decisions at intermediate management cycles (Stephenson 2014).
Acknowledgments
We thank the Pacific Islands Climate Science Center (PICSC) for project funding and support (change of allocation MS3590, CM3801, and cooperative agreement G13AC00125). We also thank PICSC for organizing initial discussions and for collaboration with David Helweg, David Benitez, Colleen Cole, Tom Giambelluca, Christian Giardina, Kevin Hamilton, Sarah Nash, Delwyn Oki, Becky Ostertag, Julia Rowe, Gordon Tribble, Namaka Whitehead, and Sharon Ziegler-Chong. We acknowledge various sources for data used in our models: Rainfall Atlas and Climate of Hawai`i from Giambelluca et al. (2013, 2014); International Pacific Research Center from Zhang et al. (2012, 2016a, 2016b); and plant species range models from Price et al. (2012).[1] Jeff Burgett, Darcy Hu, and Adam Vorsino provided constructive comments on earlier versions of this manuscript. Any use of trade, product, or firm names is for descriptive purposes only and does not imply endorsement by the US government.
[1] Output data from the models used to assess potential impacts of projected climate change on vegetation management strategies are published at https://www.sciencebase.gov/catalog/item/5362af3ee4b0c409c6289bc7.
References
Araújo, M. B., and A. T. Peterson. 2012. Uses and misuses of bioclimatic envelope modeling. Ecology 93:1527–1539.
Camp, R. J., S. P. Berkowitz, K. W. Brinck, J. D. Jacobi, R. Loh, J. Price, and L. B. Fortini. n.d.Potential impacts of projected climate change on vegetation-management strategies in Hawai`i Volcanoes National Park. USGS Scientific Investigations Report. US Geological Survey, Reston, VA, USA. In press.
Chauvenet, A. L. M., J. G. Ewen, D. P. Armstrong, T. M. Blackburn, and N. Pettorelli. 2013. Maximizing the success of assisted colonizations. Animal Conservation 16:161–169.
Chen, I.-C., J. K. Hill, R. Ohlemuller, D. B. Roy, and C. D. Thomas. 2011. Rapid range shifts of species associated with high levels of climate warming. Science 333:1024–1026.
Elison Timm, O., H. F. Diaz, T. W. Giambelluca, and M. Takahashi. 2011. Projection of changes in the frequency of heavy rain events over Hawaii based on leading Pacific climate modes. Journal of Geophysical Research: Atmospheres 116:D04109.
Epstein, J. M., and R. Axtell. 1996. Growing artificial societies—Social sciences from the bottom up. The Brookings Institution Press, Washington, DC, 228 pages.
Foden, W. B., S. H. Butchart, S. N. Stuart, J.-C. Vie, H. R. Akçakaya, A. Angulo, L. M. DeVantier, A. Gutsche, E. Turak, L. Cao, S. D. Donner, V. Katariya, R. Vernard, R. A. Holland, A. F. Hughes, S. E. O’Hanlon, S. T. Garnett, Ç. H. Şekercioğlu, and G. M. Mace. 2013. Identifying the world’s most climate change vulnerable species—A systematic trait-based assessment of all birds, amphibians and corals. PLoS ONE 8:e65427.
Fortini, L., J. Price, J. Jacobi, A. Vorsino, J. Burgett, K. Brink, F. Amidon, S. Miller, S. Gon, G. Koob, and E. Paxton. 2013. A landscape-based assessment of climate change vulnerability for all native Hawaiian plants. University of Hawai`i at Hilo, Hawai`i Cooperative Studies Unit Technical Report HCSU-044, Hilo, Hawaii, USA. 134 pages.
Gallagher, R. V., R. O. Makinson, P. M. Hogbin, and N. Hancock. 2015. Assisted colonization as a climate change adaption tool. Austral Ecology 40:12–20.
Garcia, R. A., M. Cabeza, R. Altwegg, and M. B. Araujo. 2016. Do projections from bioclimatic envelope models and climate change metrics match? Global Ecology and Biogeography 25:65–74.
Giambelluca, T. W., M. A. Nullet, and T. A. Schroeder. 1986. Rainfall atlas of Hawai`i. Report R76. State of Hawai`i, Department of Land and Natural Resources Hawai`i, Honolulu, Hawai`i, USA. 267 pages.
Giambelluca, T. W., Q. Chen, A. G. Frazier, J. P. Price, Y.-L. Chen, P.-S. Chu, J. K. Eischeid, and D. M. Delparte. 2013. Online Rainfall Atlas of Hawai`i. Bulletin of the American Meteorological Society 94:313–316.
Giambelluca, T. W., X. Shuai, M. L. Barnes, R. J. Alliss, R. J. Longman, T. Miura, Q. Chen, A. G. Frazier, R. G. Mudd, L. Cuo, and A. D. Businger. 2014. Evapotranspiration of Hawai`i. Final report submitted to the US Army Corps of Engineers–Honolulu District, and the Commission on Water Resource Management, State of Hawai`i.
Gutmann, E. D., R. M. Rasmussen, C. Liu, K. Ikeda, D. J. Gochis, M. P. Clark, J. Dudhia, and G. Thompson. 2012. A comparison of statistical and dynamical downscaling of winter precipitation over complex terrain. Journal of Climate 25:262–281.
IPCC (Intergovernmental Panel on Climate Change). 2014. Climate Change 2014—Impacts, Adaptation, and Vulnerability, Part A—Global and Sectoral Aspects Contribution of Working Group II to the Fifth Assessment Report of the Intergovernmental Panel on Climate Change. C. B. Field, V. R. Barros, D. J. Dokken, K. J. Mach, M. D. Mastrandrea, T. E. Bilir, M. Chatterjee, K. L. Ebi, Y. O. Estrada, R. C. Genova, B. Girma, E. S. Kissel, A. N. Levy, S. MacCracken, P. R. Mastrandrea, and L. L. White, editors. Cambridge University Press, Cambridge, UK, 1,132 pages.
Lauer, A., C. Zhang, O. Elison-Timm, Y. Wang, and K. Hamilton. 2013. Downscaling of climate change in the Hawaii region using CMIP5 results—On the choice of the forcing fields. Journal of Climate 26:10006–10030.
Loh, R., J. T. Tunison, C. Zimmer, R. Mattos, and D. Benitez. 2014. A review of invasive plant management in Special Ecological Areas, Hawai`i Volcanoes National Park, 1984–2007. Technical Report No. 187. University of Hawai`i, Pacific Cooperative Studies Unit, Honolulu, Hawai`i, USA. 35 pages. Available at http://manoa.hawaii.edu/hpicesu/techrep.htm.
Moir, M. L., P. A. Vesk, K. E. C. Brennan, R. Poulin, L. Hughes, D. A. Keith, M. A. McCarthy, and D. J. Coates. 2012. Considering extinction of dependent species during translocation, ex situ conservation, and assisted migration of threatened hosts. Conservation Biology 26:199–207.
Mora, C., A. G. Fraizier, R. J. Longman, R. S. Dacks, M. M. Walton, E. J. Tong, J. J. Sanchez, L. R. Kaiser, Y. O. Stender, J. M. Anderson, C. A. M. Ambrosino, I. Fernantez-Silva, L. M. Giuseffi, and T. W. Giambelluca. 2013. The projected timing of climate departure from recent variability. Nature 502:183–187.
Morrison, M. L., and L. S. Hall. 2002. Standard terminology—Toward a common language to advance ecological understanding and application. Pages 43–52 in J. M. Scott, P. J. Heglund, M. L. Morrison, M. G. Raphael, W. A. Wall, and F. B. Samson, editors. Predicting species occurrences—Issues of accuracy and scale. Island Press, Washington, DC, USA.
Power, S. B. 2014. Expulsion from history. Nature 511:38–39.
Price, J. P., J. D. Jacobi, S. M. Gon, III, D. Matsuwaki, L. Mehrhoff, W. Wagner, M. Lucas, and B. Rowe. 2012. Mapping plant species ranges in the Hawaiian Islands—Developing a methodology and associated GIS layers. US Geological Survey Open-File Report 2012–1192. US Geological Survey, Reston, Virginia, USA. 34 pages. Available at http://pubs.usgs.gov/of/2012/1192/.
Price, J. P., T. Wong, and J. D. Jacobi. 2015. Modeling climate-driven changes to dominant vegetation in the Hawaiian Islands. Final report to Pacific Islands Climate Science Center. US Geological Survey, Reston, Virginia, USA. 10 pages. Available at https://nccwsc.usgs.gov/project-component/4f8c650ae4b0546c0c397b48/55e8552be4b0dacf699e66bd.
Rout, T. M., E. McDonald-Madden, T. G. Martin, N. J. Mitchell, H. P. Possingham, and D. P. Armstrong. 2013. How to decide whether to move species threatened by climate change. PLoS ONE 8:e75814.
Stephenson, N. L. 2014. Making the transition to the third era of natural resources management. The George Wright Forum 31:227–235.
Vorsino, A. E., L. B. Fortini, F. A. Amidon, S. E. Miller, J. D. Jacobi, J. P. Price, S. O. Gon, III, and G. A. Koob. 2014. Modeling Hawaiian ecosystem degradation due to invasive plants under current and future climates. PLoS ONE 9:e95427.
Watson, J. E. M., T. Iwamura, and N. Butt. 2013. Mapping vulnerability and conservation adaptation strategies under climate change. Nature Climate Change 3:989–994.
Zhang, C., Y. Wang, A. Lauer, and K. Hamilton. 2012. Configuration and evaluation of the WRF model for the study of Hawaiian Regional Climate. Monthly Weather Review 140:3259–3277.
Zhang, C., Y. Wang, K. Hamilton, and A. Lauer. 2016a. Dynamical downscaling of the climate for the Hawaiian Islands. Part I: Present day. Journal of Climate 29:3027–3048.
———. 2016b. Dynamical downscaling of the climate for the Hawaiian Islands. Part II: Projection for the late twenty-first century. Journal of Climate 29:8333–8354.
About the authors
Richard J. Camp is a statistician at US Geological Survey, Pacific Island Ecosystems Research Center, Hawai`i National Park, Hawai`i. He can be reached at e-mail us. Rhonda Loh is the chief of Natural Resources Management at Hawai`i Volcanoes National Park, and formerly was a biotechnician (1995–1998) and botanist (1998–2007) for the park. S. Paul Berkowitz is a GIS specialist at Hawai`i Cooperative Studies Unit, University of Hawai`i at Hilo, Hawai`i. Kevin W. Brinck is a quantitative ecologist at Hawai`i Cooperative Studies Unit, University of Hawai`i at Hilo, Hawai`i. James D. Jacobi is a botanist at US Geological Survey, Pacific Island Ecosystems Research Center, Hawai`i National Park, Hawai`i. Jonathan Price is an associate professor in the Department of Geography and Environmental Sciences, University of Hawai`i at Hilo, Hawai`i. Sierra McDaniel is a botanist at Hawai`i Volcanoes National Park, and previously was a biological science technician (2001–2004) and a horticulturalist (2006–2007) for the park. Lucas B. Fortini is a research ecologist at US Geological Survey, Pacific Island Ecosystems Research Center, Hawai`i National Park, Hawai`i.
Tags
- hawaiʻi volcanoes national park
- park science journal
- ps v34 n1
- research report
- bioclimatic envelope monitoring
- climate change
- hawaii volcanoes national park
- management strategies
- plant disbtributions
- precipitation
- protected area prioritization
- special ecological areas (seas)
- species range
- temperature
- environmental impacts
- science
- native plants