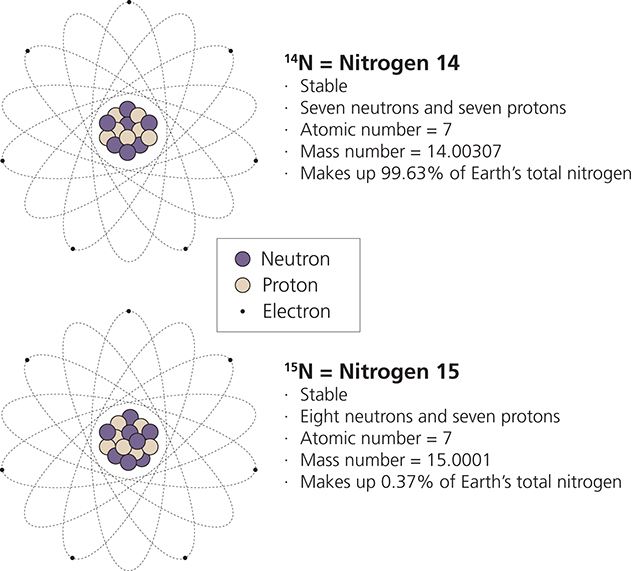
NPS/NRSS Office of Education and Outreach
SO WHAT IS AN ISOTOPE? Answering this question requires a review of basic atomic structure. Chemical elements are defined by the number of protons in the nucleus, called the atomic number. Hydrogen, for example, has one proton, so its atomic number is also one. Nitrogen has seven protons while oxygen has eight, so their atomic numbers are seven and eight, respectively. However, the nucleus also contains neutrons in addition to the protons. These two types of particles are effectively the same size and weight, and their sum constitutes an atom’s mass number. The number of protons and neutrons is often—but not always—equal. Two atoms having the same atomic number (i.e., belonging to the same element) but having a different mass number (i.e., different numbers of neutrons) are called isotopes (fig. 1). Oxygen (O), for example, has three naturally occurring isotopes, which can be written as 8/16O, 8/17 O, and 8/18O. In this example the atomic number (elemental identity) is displayed at the lower left of the element symbol, while the mass number (isotope) is displayed at the upper left.
There are two basic types of isotopes. Radioisotopes are unstable because of the extra neutron(s), and spontaneously release radiation in the form of particles and energy. This release, which is statistically predictable, can actually change the atom’s atomic number, resulting in the production of a different element. Stable isotopes, by contrast, have a nucleus that is stable indefinitely. They do not emit radiation but persist alongside the other isotopes. All three of the naturally occurring oxygen isotopes are stable, for example, so they do not spontaneously release radiation and decay into other elements.
Different isotopes of the same element all take part in chemical reactions, but at slightly different rates. “Lighter” isotopes move around more easily than “heavier” ones. This differential movement is called fractionation, and it affects the ratio of isotopes present in any given sample of water, soil, rock, tissue, or air. Scientists can measure isotope ratios in a laboratory. They usually present isotope data as “δ” values (often called “del” values for the Greek letter “delta”) in parts per mil (i.e., per thousand, ‰) relative to a standard reference sample. Samples may be described as either “depleted” or “enriched” in a given isotope (usually the heavier, less common one) relative to the standard. For example, the Pee Dee Belemnite (Belemnitella americana), or “PDB,” is a standard reference for carbon isotopes based on calcite crystals (calcium carbonate, CaCO3) found in fossils of this species collected from a specific locality in South Carolina. The international standard for nitrogen is atmospheric air, which is very well mixed around the globe.
The science of isotopes—whether they are naturally occurring or created artificially in a laboratory—is well understood and widely applied in our world today (USGS 2007). Radioisotopes are widely used for energy sources in medicine and nuclear engineering as well as for “clocks” (like 14C, or carbon-14) for dating natural samples. Many people, however, are surprised to learn how many ways scientists use stable isotopes—especially in the natural sciences. First, scientists use isotopic ratios, which are also called “signatures” or “fingerprints,” to characterize, classify, and constrain distinct sources for atoms in different samples. Second, scientists can measure fractionation under controlled conditions to make inferences about how samples are affected by specific processes such as evaporation or photosynthesis. Third, they can learn about complex changes through space and time by carefully mapping stable isotopes or monitoring artificial isotope-labeled “tracers” in systems such as flowerpots, watersheds, fossils, tree rings, and sediment layers.
In the present study, nitric acid particles created from fossil fuel combustion contain isotopes of nitrogen and oxygen that differ from natural sources. Combustion temperatures and atmospheric reactions create distinct isotopic signatures that can be measured against background values to determine their origin. In this case, the article authors sampled nitrogen and oxygen isotopes of nitric acid deposition in Joshua Tree National Park and compared patterns to interpret two distinct areas where oxygen isotopes are a little heavier than natural “background” values. This finding is interpreted to reflect two artificial sources of the burning of fossil fuels.
Reference
USGS (U.S. Geological Survey). 2007. Resources on isotopes. USGS Isotope Tracers Project, Menlo Park, California, USA. Accessed 8 February 2016 from https://wwwrcamnl.wr.usgs.gov/isoig/isopubs.
Download: PDF of this article
This article published
Online: 6 May 2016; In print: 25 March 2016
URL
https://www.nps.gov/ParkScience/articles/parkscience32_2_67_shelley_3839.htm
Suggested citation
Shelley, D. C. 2016. Isotopes in environmental science. Park Science 32(2):67.
This page updated
5 May 2016
Site navigation
• Back to Volume 32, Number 2
• Back to Park Science home page
Last updated: March 21, 2021