The forests are also needed for mitigating extreme climatic fluctuations, holding the soil on the slopes, retaining the moisture in the ground … Franklin D. Roosevelt, on receiving the Schlich Forestry Medal from the Society of American Foresters, 29 January 1935
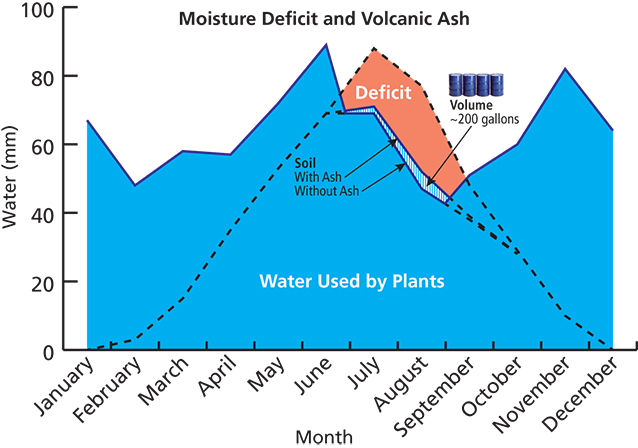
Key words: climate, Glacier National Park, soil, water balance
THE SHADOWY, BEAUTIFUL FOREST along the shore of Lake McDonald is the image that many visitors take home with them and remember from Glacier National Park, Montana. These forests along the Going-to-the-Sun highway host a famous lodge, popular campgrounds, and favorite hiking trails as well as important habitats for a diversity of plants and animals. Both forests and soils evolved on this once-glaciated and barren landscape. Today a lush cedar and hemlock forest flanks the lakeshore, sustaining natural ecosystems and human activities to enjoy them. But what lies beneath this forest, what sustains it, and what will the future hold?
This year, 2015, is the International Year of Soil, a time to highlight the critical—but often overlooked—roles that soil plays in the ecological processes of natural landscapes (Food and Agriculture Organization of the United Nations 2015). In this article we describe how soil properties interacting with climate and terrain affect the seasonal availability of moisture for vegetation in Glacier National Park. Our approach uses a mathematical water balance model that integrates soil survey, digital terrain, and climate data to gain insight into the interaction between physical factors and climate. This method is possible using freely available gridded climate and soil survey data (Thornton et al. 2014; NRCS 2013). The soil survey of Glacier is being updated, so we use it as a timely example that showcases the integration of soil survey data with climate and modeling tools to illuminate important seasonal ecological processes—namely variation in soil moisture.
Land managers, soil scientists, and even presidents have long recognized that soil plays an important role in temporarily storing water as it moves through the hydrologic cycle. More challenging is to quantify that role and how it affects vegetation patterns in remote and rugged landscapes like Glacier. Understanding variation in soil moisture can help us comprehend drought and wildfire probability, severity, and recovery, all processes that shape vegetation distributions. Indirectly, soil moisture also affects competition, forest disease, and vegetation response to extreme weather events, all of which contribute to the vegetation pattern in Glacier and other national parks (Kane et al. 2015; Stephenson 1998).
Climate interactions with terrain and soil properties are complex, but a water balance model helps integrate these factors quantitatively to estimate the persistence of moisture in soil (Lutz et al. 2010). If soil is considered a natural bank account and water is currency, then, like balancing a checkbook, water balance models track water input and loss. Each spring the deep winter snowpack melts, replenishing the soil moisture supply. What does not drain to groundwater or run off to fill rivers and lakes remains in the soil to be used by plants. As water is consumed over the course of the following summer, rain may occasionally replenish soil moisture. If not, however, then moisture deficits accrue. Deficit is the difference between water used and water needed by plants to grow optimally. Although different species are adapted to tolerate typical deficits where they grow (think cactus in deserts), an accrual of deficit above a species’ tolerance can cause wilting or death. Following the banking analogy, a water deficit is akin to an increasing overdraft that stresses plants as the duration and magnitude of deficit grow during dry spells. In Glacier National Park a greater soil water-holding capacity confers an advantage through dry periods because plants have a greater volume of stored water to use and thus a greater chance of surviving through a hot and dry period with minimal stress.
Terrain influences water storage
Terrain also plays a role in the variation of soil moisture through the effects of slope steepness and aspect. The aspect of a slope refers to the orientation or direction the slope faces, which determines its potential for solar gain (sun exposure). The significant effect of aspect is apparent in sun-sheltered, north-facing slopes that afford protection for glaciers and snowfields. On the other hand, south-facing slopes at the same elevation have more solar gain and energy to drive melt and evapotranspiration, which cause soil in those locations to dry more quickly. Plant species distributions follow patterns across landscapes that have similar water balance characteristics (Stephenson 1998). Plants use the effects of terrain to compensate for limitations of energy at higher elevations and for limitations of moisture at lower elevations. Evidence of this compensatory effect in Glacier is apparent not only for trees, but also for grasses like Idaho fescue (Festuca idahoensis), an important forage species for ungulates. This species can be found on north-facing slopes at lower elevations, where it minimizes moisture stress, and is only found on warm, south-facing slopes at higher elevations, where it maximizes the energy-limited conditions of lower temperatures through increased solar gain.
Soil properties influence water storage
A fascinating soil feature in Glacier that affects water storage is the presence of volcanic ash derived from the former Mount Mazama, which collapsed to form the caldera that holds Crater Lake some 885 km (550 miles) to the southwest in Crater Lake National Park (Oregon). An eruption 7,600 years ago dispersed a tremendous volume of ash that blanketed large areas of North America (Zdanowicz et al. 1999). As a windborne material, volcanic ash consists of silt-sized glass particles that are nutrient-poor but are very porous and retain more water than typical soil particles of the same size class. Since deposition, surface runoff and erosion have redistributed the once-uniform blanket of ash to create the patchy distribution with varying thicknesses as described by soil scientists working in the region today. In Glacier, Mazama ash varies in thickness from 15 to 60 cm (5.9 to 23.6 in) where it occurs, but is generally thicker west of the Continental Divide. Due to its porous nature, ash overlying a rocky and coarser-textured material such as glacial till results in a mulching effect that boosts water storage and availability for plants (McDaniel et al. 2005).
A case study in nuance
A 34-year monthly average climate diagram for a red cedar (Thuja plicata) and western hemlock (Tsuga heterophylla) stand along the shore of Lake McDonald shows that this location typically experiences a period of soil moisture deficit during the summer months, the period that most strongly determines the distribution of western red cedar and western hemlock (fig. 1, top of page; Mathys et al. 2014).
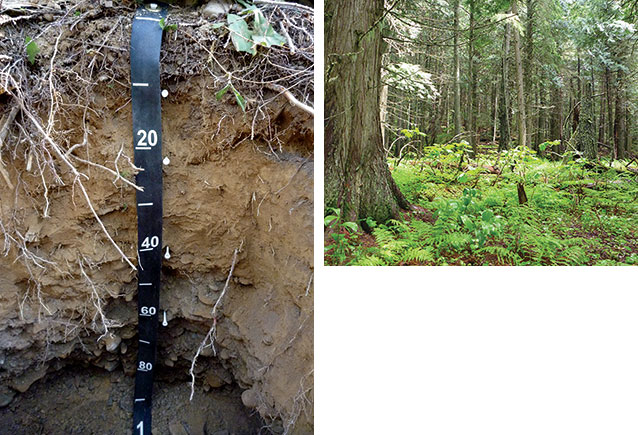
Photos courtesy of Patrick O’Connell and Jay Skovlin
However, hidden below the forest floor in some locations lies the Mazama ash. Where it occurs at lower elevations the soil with ash retains more water than non-ashy soil, which helps offset moisture stress during dry periods. The modeled moisture deficit during summer is about 15% lower in a soil that has a 36 cm (14.2 in)–thick volcanic ash layer (fig. 2). By making a few simplifying assumptions we determined that during this critical deficit period the relative difference is roughly 757 liters (200 gallons) of additional water per tree growing on soil with ash. While this relative difference in water availability is small compared with the annual water use of mature trees, it has been shown that the distribution of these two tree species is highly sensitive to variation in water-holding capacity of soil (Mathys et al. 2014). It also suggests that because red cedar and western hemlock are at the eastern extent of their range in Glacier they may persist longer in sites where climate and soil properties moderate the effects of increasing deficits that are likely with climate change (McKenzie 2012; Mathys et al. 2014). Although other factors like disturbance and competition matter, when we look broadly at vegetation distributions of many plant species the spatial patterns at biome, landscape, and site scales reflect variation in seasonal soil moisture availability (Stephenson 1998).
Management implications
If deficits increase over time as projected (Fagre 2007) and cedar and hemlock stands decrease in area in Glacier, then these stands may become increasingly important to other animal and plant species that inhabit these forests. Understanding where suitable sites for plant species exist, and for how long those sites may confer resilience or resistance to climate change, could be useful to managers as they strive to mitigate impacts of the changing climate. For example, if managers want to retain or restore red cedar or hemlock stands in Glacier, they could use soil maps and water balance models to help identify locations that are more likely to experience stress sooner and to find locally suitable conditions that may not exist presently but may exist in the future. As temperatures warm and summer deficit regimes change spatially, suitable potential habitat may be at higher elevations from current red cedar and hemlock forest stands or may be on different slopes or aspects with suitable soil, which could become target areas for active management. Soil survey data combined with a water balance model can help managers untangle the complicated interactions of these various factors that affect water availability and deficits.
Conclusion
Small differences in soil properties play roles in the historical and current distribution of vegetation types in Glacier and will continue to influence vegetation patterns in the future. The Natural Resources Conservation Service soil survey of Glacier National Park describes the variation of soil in this rugged and spectacular landscape, and here we describe climate interactions with terrain and soil that affect seasonal moisture availability. Soil moisture is a temporally and spatially dynamic landscape feature that affects drought, fire, and forest disease, which in turn influence vegetation patterns and subsequent use of the landscape by wildlife (Stephenson 1998; Kane et al. 2015; McCloskey et al. 2009; Singer 1979). Coupled with long-term vegetation monitoring like that being conducted by parks and the National Park Service Inventory and Monitoring Program, water balance models could help identify thresholds or tipping points of vegetation change and potential locations with optimum conditions for successful restoration efforts. Processes like fire and recovery are dramatic manifestations of climate and soil interactions, but some processes are more subtle, like the water balance character of a site that determines suitability for different species. These factors, bold and subtle, add character and nuance to the repeating theme in the architecture of vegetation pattern. In this International Year of Soils we call attention to these themes, especially those that are more subtle, and hope to raise awareness of soil and how considering interactions with climate could be used by park managers. Though often overlooked, these subtleties may be key to stewarding large landscapes in a rapidly changing world.
Acknowledgments
Work on the Soil Survey of Glacier National Park was conducted by the NRCS through the NPS Inventory and Monitoring Program, research permit GLAC-2011-SCI-0175. Conclusions expressed herein are those of the authors and may not reflect thoughts of subject-matter experts or park staff.
References
Fagre, D. B. 2007. Adapting to the reality of climate change at Glacier National Park, Montana, USA. Pages 221–235 in Instituto de Hidrologia, Meteorologia, y Estudios Ambientales (IDEAM). Proceedings of the First International Conference on the Impact of Climate Change on High-Mountain Systems, 21–23 November 2005, Bogotá, Colombia. https://www.nrmsc.usgs.gov/files/norock/products/GCC/Fagre_Bogota_Conference_paper_FINAL.pdf.
Food and Agriculture Organization of the United Nations. 2015. 2015 International Year of Soils: Healthy soils for a healthy life. Accessed 8 October from http://www.fao.org/soil-2015/en.
Kane, V. R., J. A. Lutz, A. Cansler, N. A. Povak, D. J. Churchill, D. F. Smith, J. T. Kane, and M. P. North. 2015. Water balance and topography predict fire and forest structure patterns. Forest Ecology and Management 338:1–13.
Lutz, J., J. W. van Wagtendonk, and J. W. Franklin. 2010. Climatic water deficit, tree species ranges, and climate change in Yosemite National Park. Journal of Biogeography 37(5):936–950.
Mathys, A., N. C. Coops, and R. H. Waring. 2014. Soil water availability effects on the distribution of 20 tree species in western North America. Forest Ecology and Management 313:144–152.
McCloskey, S. P. J., L. D. Daniels, and J. A. McLean. 2009. Potential impacts of climate change on western hemlock looper. Northwest Science 83(3):225–238.
McDaniel, P. A., M. A. Wilson, R. Burt, D. Lammers, T. D. Thorson, C. L. McGrath, N. Peterson. 2005. Andic soil inland Pacific Northwest, USA: Properties and ecological significance. Soil Science 170(4):300–310.
McKenzie, D. A. 2012. Postfire species shifts, and growth and regeneration of western red cedar (Thuja plicata), a range margin species, in Glacier National Park, Montana. Dissertation. University of Wyoming, Laramie, Wyoming, USA.
NRCS (Natural Resources Conservation Service). 2013. Web Soil Survey. U.S. Department of Agriculture, Natural Resources Conservation Service, Washington, D.C., USA. Singer, F. J. 1979. Habitat partitioning and wildfire relationships of cervids in Glacier National Park, Montana. The Journal of Wildlife Management 43(2):437–444.
Stephenson, N. L. 1998. Actual evapotranspiration and deficit: Biologically meaningful correlates of vegetation distribution across spatial scales. Journal of Biogeography 25(5):855–870.
Thornton, P. E., M. M. Thornton, B. W. Mayer, N. Wilhelmi, Y. Wei, R. Devarakonda, and R. B. Cook. 2014. Daymet: Daily surface weather data on a 1-km grid for North America, version 2. Data set. (Temporal range: 1980/01/01-2013/12/31.) Oak Ridge National Laboratory Distributed Active Archive Center, Oak Ridge, Tennessee, USA. Accessed 15 January 2015 from http://daac.ornl.gov.
Zdanowicz, C. M., G. A. Zielinksi, and M. S. Germani. 1999. Mount Mazama eruption: Calendrical age verified and atmospheric impact assessed. Geology 27:621–624.
About the authors
Jay Skovlin is a USDA Natural Resources Conservation Service soil scientist in Missoula, Montana. David Thoma is a hydrologist with the NPS Northern Colorado Plateau and Greater Yellowstone Inventory and Monitoring Networks.
Download: PDF of this article
This article published
Online: 6 May 2016; In print: 25 March 2016
URL
https://www.nps.gov/ParkScience/articles/parkscience32_2_60-63_skovlin_thoma_3837.htm
Suggested citation
Skovlin, J., and D. Thoma. 2016. Interactions underfoot: The subtle influence of soil moisture on vegetation pattern. Park Science 32(2):60–63.
This page updated
5 May 2016
Site navigation
• Back to Volume 32, Number 2
• Back to Park Science home page
Last updated: February 11, 2021