The degree of genetic, morphological, and physiological differentiation ... suggest[s] that newts residing within the steep-walled caldera have been physically isolated from outside newt populations and have adapted to the local lake environment.
Get PDF
Download PDF of this article
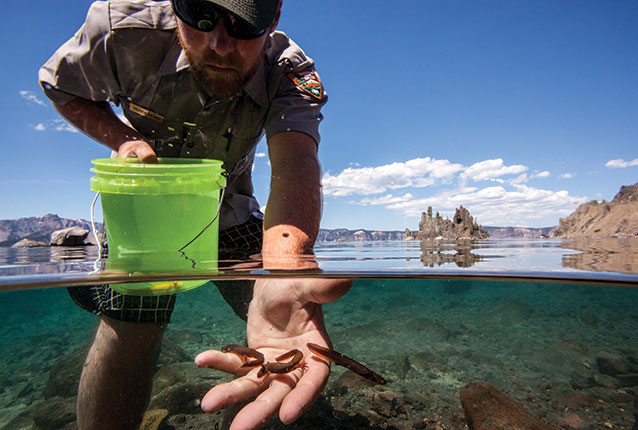
Copyright Jeremy Monroe, Freshwaters Illustrated
Abstract and key words
Abstract: The signal crayfish (Pacifastacus leniusculus) was introduced to Crater Lake in 1915 and is now displacing a native salamander. Before the introduction of this crayfish species, no crayfish existed in the lake. A proposed subspecies of the rough-skinned newt (Taricha granulosa), the Mazama newt (T. granulosa mazamae) is reportedly endemic to Crater Lake, Oregon. The Mazama newt is morphologically, genetically, and physiologically distinct from populations of newts outside of the lake. Observations by park biologists through the 1900s suggested a decline in Mazama newt distribution and an increase in signal crayfish abundance, which led us to investigate current distribution, relative abundance, and interactions between crayfish and newts. Results indicate that crayfish have expanded in distribution to occupy nearly 80% of the lakeshore. Newts remain in areas that crayfish have yet to invade but are almost entirely absent in areas occupied by crayfish. Isotopic signatures of carbon and nitrogen in newt and crayfish tissue indicate that the two species eat similar food items and occupy a similar position (primarily predacious) in the food web. Abundance of other aquatic invertebrates was dramatically reduced in locations with crayfish compared with areas of the lake without crayfish. Mesocosm experiments conducted with newts and crayfish revealed that crayfish prey on newts, displace newts from cover, and generally alter newt behavior when the two species co-occur. This evidence, taken together, suggests that further crayfish expansion likely will cause additional declines in newt abundance and distribution, and could lead to extinction of the unique Mazama newt. Conserving this irreplaceable component of Crater Lake’s native fauna will be a challenge for park resource managers.
Key Words: Crater Lake, invasive species, rough-skinned newt (Taricha granulosa), signal crayfish (Pacifastacus leniusculus)
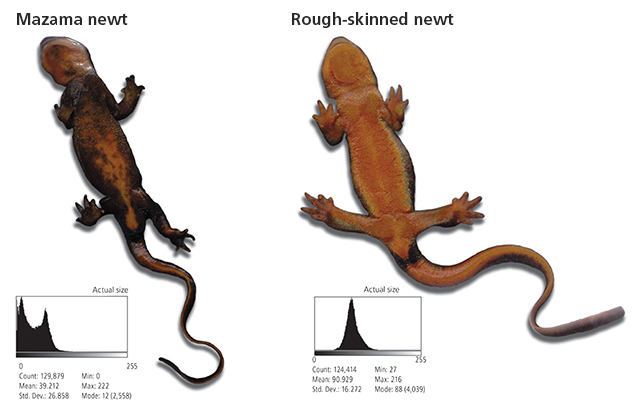
NPS photos and histograms
CRATER LAKE IS THE DEEPEST LAKE IN THE UNITED STATES, with a maximum depth of 592 m (1,943 ft), and is well-known for its blue color and extreme water clarity (Bacon et al. 2002; Larson et al. 2007). Nearly a half million visitors annually come to enjoy the stunning beauty of this special lake, which formed roughly 7,700 years ago in the collapsed caldera of Mount Mazama, a volcano in the southern Oregon Cascade Mountains (Bacon et al. 2002). The lake has been protected in Crater Lake National Park and managed in near-pristine condition since 1902, yet this remarkable ecosystem is not immune to the impacts of introduced species. A unique population of salamanders in Crater Lake is now threatened by introduced crayfish, and park managers face the challenge of controlling crayfish to conserve the native amphibian.
The Mazama newt (Taricha granulosa mazamae) is a distinct population of the more widely distributed rough-skinned newt (T. granulosa) and occurs only within Crater Lake. The Mazama newt was first formally described in the 1940s and proposed as a subspecies characterized by unusually dark ventral pigmentation (Myers 1942; Farner and Kezer 1953; fig. 1, above). Recent genetic analyses of rough-skinned newts by the University of Idaho and the U.S. Geological Survey (USGS) confirmed that newts in Crater Lake are genetically distinct from other Pacific Northwest newt populations (University of Idaho and the Orianne Society, S. Spear, conservation geneticist, personal communication, Moscow, Idaho, 31 December 2014). In addition, the Mazama newt differs from rough-skinned newts outside of Crater Lake in appearance, toxicity, and possibly life history expression.
The rough-skinned newt is the only terrestrial animal to contain tetrodotoxin (TTX), the same neurotoxin that is found in deadly saltwater puffer fish (Tetraodontidae) and blue-ringed octopus (Hapalochlaena spp.) Most rough-skinned newts outside the Crater Lake caldera contain measurable levels of TTX and are highly toxic (Brodie 1968). Rough-skinned newts have evolved bright coloring to warn predators that they are poisonous. In addition to their darker ventral coloration (fig. 1), Mazama newts have extremely low toxicity levels (average whole newt toxicity = 0.50 µg TTX) compared with newt populations sampled at other high-elevation sites outside the caldera (6.91 µg TTX) and newts at low-elevation sites along the Oregon coast (2,000 µg TTX; Stokes et al. in press). Our recent observations also suggest that the Mazama newt has a more predominantly aquatic life history than typical rough-skinned newts, although terrestrial Mazama newts were reported to be common historically “under the rocks and driftwood along the shore” of Crater Lake (Farner and Kezer 1953).
Newts in Crater Lake may be less toxic and darker in color than other populations for multiple reasons. Lower toxicity may be associated with the absence or reduction of a terrestrial life history stage and terrestrial predation, the lack of evolutionary history with native aquatic predators, or insufficient energy available to produce toxins in the ultra-oligotrophic (i.e., nutrient-poor) lake environment. Darkened coloration on ventral surfaces may also represent a darkening response to elevated ultraviolet radiation (Belden and Blaustein 2002; Garcia et al. 2009), which penetrates to great depth in the exceptionally clear lake (Hargreaves et al. 2007).
By analyzing scanned newt images, we have quantified color differences between Mazama newts and rough-skinned newts from nearby populations. Our analysis used average gray scale intensity of the images, ranging from 0, representing true black, to 255, representing complete white. Using this approach we were able to summarize the color distribution for representative samples of newts captured inside and outside of Crater Lake and express this variation in gray scale values. Preliminary results suggest that the ventral surfaces of Mazama newts are quantitatively darker. For example, in figure 1, the newt from Crater Lake (mean gray scale intensity = 39) contains more black color than the newt from nearby Spruce Lake (mean gray scale intensity = 91).
The degree of genetic, morphological, and physiological differentiation in the Mazama newt is particularly striking given the close proximity of more typical rough-skinned newt breeding populations within 12 km (7 mi) of Crater Lake. These traits suggest that newts residing within the steep-walled caldera have been physically isolated from outside newt populations and have adapted to the local lake environment.
Prior to the introduction of nonnative fish in 1888 and the subsequent introduction of crayfish, the Mazama newt presumably occupied a key ecological niche for thousands of years as the top aquatic predator in Crater Lake. Through the mid-20th century, park naturalists described newts as common along the shoreline, including Wizard Island (Farner and Kezer 1953). As recently as the 1980s, newts were frequently observed at Wizard Island and near the boat dock facility on the north shore of the lake.
Signal crayfish (Pacifastacus leniusculus) were introduced into Crater Lake as food for nonnative trout and salmon in 1915. Crayfish significantly and fundamentally modify shoreline aquatic plant and animal communities because of their aggressive behavior and consumption of a wide variety of plants and animals, including invertebrates, small fish, vascular plants, algae, and detritus (Momot 1995; Light 2003; Lodge and Hill 1994; Stenroth and Nyström 2003; Geiger et al. 2005).
Investigating crayfish impacts on newts and invertebrates
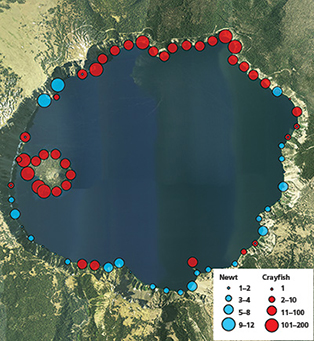
Prompted in part by the apparent decline in Mazama newt distribution at Crater Lake, we conducted several studies to investigate the effect of crayfish on newts and benthic aquatic invertebrates such as insects, snails, and worms that live on the lake bottom and are typical food sources for newts (Farner 1947). Our current studies describe the distribution and relative abundance of newts and crayfish, stable isotopic signatures of carbon and nitrogen for newts and crayfish, densities and relative abundance of invertebrates in areas of crayfish presence and absence, and the behavior of newts and crayfish in mesocosms (enclosed experiments in a pseudo-natural environment designed to control key variables).
In the century following their introduction, crayfish have colonized a large portion of the lake and may be expanding further in distribution and abundance. We documented spatial distribution and relative abundance of both crayfish and newts along the shoreline beginning in 2008 using snorkel surveys and baited traps at regularly spaced intervals around the lakeshore (fig. 2). We also documented vertical depth distribution of crayfish at five locations around the lake using baited traps set at 18 discrete depths ranging from 1 m to 300 m (3–984 ft) (fig. 3). Snorkel surveys indicated that crayfish occupied 47% of the 31 km (19 mi) of rocky shoreline in 2008 and nearly 80% in 2013 (fig. 4). In addition, we captured crayfish in traps to 250 m (820 ft) below the surface of the lake (fig. 3). Newts were locally abundant in areas where crayfish were not observed but rarely occurred in sites where crayfish were present (fig. 2). Notably, newts were consistently absent from Wizard Island and the north shore of the lake where they had been distributed historically (Farner and Kezer 1953).
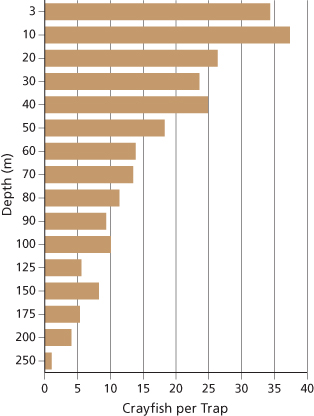
Evaluation of the stable isotope composition of carbon and nitrogen in plant and animal tissue is often used to understand animal diets and the potential for food web interactions in aquatic ecosystems (Fry 1991). The composition of these isotopes in the tissues of an organism is a direct reflection of the sources of energy the organism consumes. Carbon isotopes reflect the source of primary production (e.g., phytoplankton vs. vascular plants). Similar carbon isotopic signatures between organisms in the same ecosystem indicate a dependency or use of similar sources of carbon and, consequently, similar diets. Nitrogen isotopes indicate trophic level position in the food web, that is, primary producer (e.g., algae and plants), primary consumer or “grazer” (e.g., organisms that eat algae or plants), secondary consumer (e.g., zooplankton and many aquatic insects), or predator (e.g., fish, newts, and crayfish). Similar nitrogen isotopic signatures indicate similar positions in a food web. The average isotopic signatures of crayfish (muscle tissue) and newts (average of muscle, liver, and tail tissues) in Crater Lake (expressed as the ratios of heavy to light isotopes in the samples compared with accepted standard ratios) were d13C (‰) crayfish −8.11 ± 1.33 (mean ± standard deviation), newt −9.64 ± 2.16; and d15N (‰) crayfish 5.20 ± 0.51, newt 5.46 ± 0.66. These values are remarkably similar between species, indicating that crayfish and newts in Crater Lake eat similar food items and occupy the same trophic level (primarily predators).
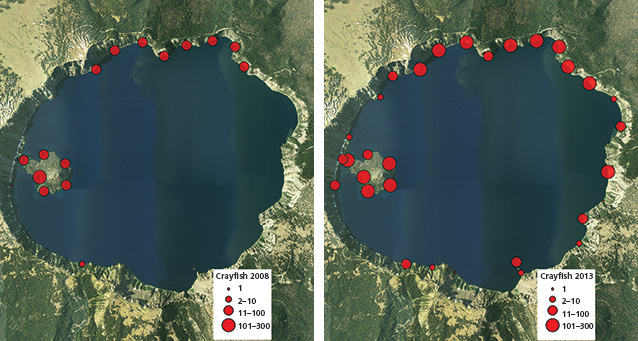
We sampled benthic aquatic invertebrates in areas of the lake with and without crayfish. Scuba divers collected samples from hard substrate at depths of 1, 3, and 10 m (3, 10, and 33 ft) using a battery-powered vacuum. After collection, invertebrate samples were preserved in ethanol and later sorted and identified to the lowest taxonomic resolution possible—genus for all taxonomic groups except oligochaete worms (identified to family) and midges (identified to species). Invertebrate abundance was dramatically reduced in locations with crayfish compared with areas of the lake without crayfish (Henery et al. 2012). For example, the average density of aquatic worms was 93% lower in crayfish areas than in areas not occupied by crayfish. Likewise, midge density was 84% lower in crayfish areas. On average, the total density of invertebrates was reduced by 78% in areas with crayfish. Given the substantial overlap in diet between crayfish and newts, such dramatic reductions in food availability in an already unproductive lake ecosystem may have profound negative effects on newt growth and survival.
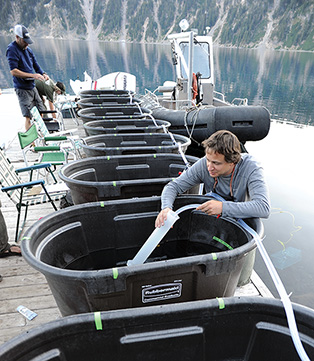
NPS Photo
We conducted mesocosm experiments at Wizard Island to evaluate newt behavior in the absence and presence of crayfish at multiple densities. The experiments used 380-liter (100 gal) tanks to simulate the rocky shoreline conditions of the lake (fig. 5). The bottoms of the tanks were covered with gravel, and one large protruding rock was located in the middle of each tank for cover. We circulated lake water through the tanks to replicate ambient water quality conditions. One newt was placed in each of the eight tanks and allowed 60 minutes to acclimate. Trials started with the addition of crayfish (zero, two, four, or eight crayfish per tank) and lasted 60 minutes, during which time newt and crayfish behavior were documented every 2 minutes. The highest crayfish density in the mesocosms (eight crayfish) was 17.6/m² (1.6/ft²). Crayfish density in areas of the lake with high abundance ranged from 13 to 24/m² (1.2 to 2.2/ft²). Behavior was quantified into four categories: swimming, resting on the bottom, occupying rock shelter, and actively avoiding crayfish. Fifteen 60-minute trials (each with eight mesocosms) were completed. We conducted experiments during daylight and after dark with both adult and larval newts to investigate whether behavioral patterns and responses to crayfish differed between life stages or under different lighting conditions or times of day. The day and night experiments were also initiated because in Crater Lake, newts are generally observed under cover during the day and in the open at night, presumably foraging on the rocky bottom.
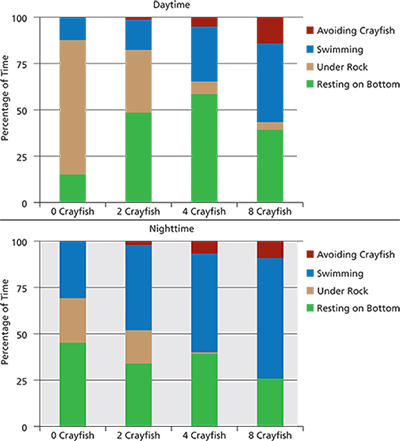
The mesocosm experiments showed that crayfish displaced newts from under rocks and also caused the newts to spend more time swimming (fig. 6). Newts in tanks with crayfish spent more than 200% more time in the open than did newts in tanks without crayfish. As the density of crayfish increased, newts spent progressively more time swimming. Following encounters with crayfish, newts in the tanks almost always swam to the water surface to breathe air. As the density of crayfish increased, the number of trips to the surface also increased. In the most crayfish-dense treatments at night, adult newts were swimming to the water surface more than once per minute on average during the 60-minute trials. By contrast, newts in crayfish-free control tanks rarely surfaced. Such a large increase in time spent swimming presumably increases newts’ energy expenditure, which may be particularly detrimental in such an extremely low-nutrient lake. Displacement into the open during the day and swimming to the water surface following a crayfish encounter leave newts prone to predation from introduced fish as well as increased exposure to ultraviolet-B radiation (UV-B). Ultraviolet radiation is harmful to many species of amphibians, including rough-skinned newts, and is thought to decrease newt growth and increase activity level (Blaustein et al. 2000). Impacts of UV-B may be especially severe in Crater Lake, where high elevation is combined with extremely transparent water, resulting in unusually high UV-B penetration deep into the lake (Hargreaves et al. 2007).
We also observed direct predation on newts by crayfish during mesocosm trials. In the 76 experimental replicates with crayfish present, 10 newts were captured by crayfish, killed, and usually eaten immediately. Nine of the 10 newts killed were larvae, even though tanks with larvae represented only 42% of the total number of experimental trials. Larvae may not recognize crayfish as a potential predator or they may be less able than adults to escape crayfish attacks. At night larvae spent more time resting on the bottom of the tank than adults (68% compared with 33% of the time) even when crayfish were present. There were occasions during the experiments when larval newts shared the area under the rock cover with crayfish, usually to the detriment of the larvae. Another possible direct effect of crayfish that we have not investigated is predation on newt eggs, which are laid under rocks and large debris along the shoreline.
Although Mazama newts are also vulnerable to predation by nonnative trout, our research and annual surveys suggest introduced crayfish have played a dominant role in the newt population’s decline. The risk of trout predation is present throughout the lake and along the entire shoreline, yet newts have persisted in areas without crayfish while disappearing from crayfish-occupied sites. Mazama newts are occasionally found in the stomachs of rainbow trout (Oncorhynchus mykiss) in Crater Lake but make up a very small proportion of the trout diet (Brode 1938; Buktenica et al. 2007). The behavioral interactions observed in mesocosms indicate that crayfish presence may increase exposure of newts to fish predators. Thus, two introduced species, rainbow trout and signal crayfish, likely have additive or synergistic negative effects on native newts.
Conclusions and management challenges
The native Mazama newt is morphologically, genetically, and physiologically distinct from populations of rough-skinned newts outside of Crater Lake. Based on multiple lines of evidence, we believe that introduced crayfish are expanding in distribution and displacing Mazama newts. The mechanisms of displacement identified here include direct predation by crayfish, newt avoidance of crayfish-occupied habitats, and competition for food. Indirect effects may include increased energy expenditure by newts during avoidance and surfacing behavior, increased exposure to ultraviolet radiation, and increased predation of newts by introduced fish as newts are supplanted from their preferred habitats during the day. Further expansion of signal crayfish in Crater Lake will lead to additional declines in Mazama newt abundance and distribution and perhaps to extinction of the proposed newt subspecies.
Park managers now face the difficult challenge of slowing or stopping the spread of crayfish to preserve the Mazama newt. The National Park Service is mandated to protect and preserve natural resources in parks, particularly taxa such as the Mazama newt that are rare, endemic, or unique to specific parks. In other, smaller lakes, crayfish removal or suppression has been used with varying success to reduce negative ecological effects of nonnative crayfish. Suppression strategies include mechanical (e.g., trapping), physical (e.g., habitat manipulation, draining), biological (e.g., use of predators), biocidal, and autocidal (e.g., X-ray irradiation) approaches (Gherardi et al. 2011). Hein et al. (2007) demonstrated that sustained trapping over five years combined with fish harvest restrictions resulted in a 95% reduction in rusty crayfish (Orconectes rusticus) from a 64-hectare (158 acre) lake in Wisconsin. Unfortunately, trapping alone is not always successful, in part because this approach is biased toward larger individuals. Recently, X-rays have been tested to sterilize male crayfish in a laboratory with the aim of developing the technology for invasive crayfish control (Aquiloni et al. 2009). Also, sex pheromones have been employed to increase crayfish trapping efficiency (Aquiloni and Gherardi 2010).
In a very large and deep water body such as Crater Lake, where crayfish have been found hundreds of meters deep, it is unclear whether any current approach will be feasible for reducing the distribution of crayfish. Park staff conducted crayfish control experiments at Phantom Ship, a small island on the southeastern side of the lake that has a relatively low density of crayfish. Continuous trapping during the summer months of 2012, 2013, and 2014 resulted in 1,840, 2,087, and 1,629 crayfish removed during the three successive years, without any apparent reduction in crayfish density. The number of crayfish observed during standardized snorkel surveys at Phantom Ship actually increased over this same period, from 30 in 2011 (before crayfish removal) to 177 in 2014. It was evident from this pilot removal study that any meaningful crayfish control efforts will require significantly more resources than are currently available at Crater Lake National Park. Crater Lake managers may need to focus attention on the current edges of crayfish distribution and attempt merely to slow further expansion, assuming that large crayfish-free areas still remain.
A workshop is proposed to bring together scientists and resource managers who have direct experience confronting and managing crayfish invasions. The workshop will draw upon the expertise of participants to determine objectives, management options, and a conceptual model of the expected outcome of alternative actions to control crayfish or otherwise conserve Mazama newts. Because introduced crayfish are an increasing threat to aquatic resources globally (Gherardi et al. 2011), recent work to assess the effect of crayfish on newts and benthic invertebrates in Crater Lake, together with results of any future suppression efforts, may have far-reaching implications both within the National Park System and for aquatic resource management more generally.
Acknowledgments
Research of crayfish and newts in Crater Lake was supported by the National Park Service, Crater Lake National Park, the U.S. Geological Survey Park Oriented Biological Support Program, and the University of Nevada, Reno (UNR). Genetic analyses were performed by Dr. Steve Spear, University of Idaho. Genetic and morphological summaries were conducted under Montana State University’s Institutional Animal Care and Use Committee (IACUC) protocol 2011-40 and NPS investigator permits CRLA 2010-SCI-0013 and CRLA 2011-SCI-0011. Toxicology was conducted in collaboration with Dr. Amber Stokes and Dr. Edmund Brodie, Jr., Utah State University (USU), and in accordance with USU’s IACUC protocol 1008. Stable isotope analyses were conducted by the laboratory of Dr. John Roden, Southern Oregon University, and invertebrate taxonomy was performed by the UNR Aquatic Ecosystems Analysis Laboratory.
Citations
Aquiloni, L., A. Becciolini, R. Berti, S. Porciani, C. Trunfio, and F. Gherardi. 2009. Managing invasive crayfish: Use of X-ray sterilization of males. Freshwater Biology 54:1510–1519.
Aquiloni, L., and F. Gherardi. 2010. The use of sex pheromones for the control of invasive populations of the crayfish Procambarus clarkia: A field study. Hydrobiologia 649:249–254.
Bacon, C. R., J. V. Gardner, L. A. Mayer, M. W. Buktenica, P. Darnell, D. W. Ramsey, and J. E. Robinson. 2002. Morphology, volcanism, and mass wasting in Crater Lake, Oregon. Geological Society of America Bulletin 114(6):675–692.
Belden, L. K., and A. R. Blaustein. 2002. UV radiation causes skin darkening in larval salamanders. Copeia 2002:748–754.
Beukema, W. 2011. Ontogenetic pattern change in amphibians: The case of Salamandra corsica. Acta Herpetologica 6(2):169–174.
Blaustein, A. R., D. P. Chivers, L. B. Kats, and J. M. Kiesecker. 2000. Effects of ultraviolet radiation on locomotion and orientation in roughskin newts (Taricha granulosa). Ethology 108:227–234.
Brode, J. S. 1938. The denizens of Crater Lake. Northwest Science 12:50–57.
Brodie, E. D., Jr. 1968. Investigations on the skin toxin of the adult rough-skinned newt, Taricha granulosa. Copeia 1968:307–313.
Buktenica, M. W., S. F. Girdner, G. L. Larson, and C. D. McIntire. 2007. Variability of kokanee and rainbow trout food habits, distribution, and population dynamics, in an ultraoligotrophic lake with no manipulative management. Hydrobiologia 574 (1) (January):235–264.
Farner, D. S. 1947. Notes on the food habits of the salamanders of Crater Lake, Oregon. Copeia 1947:259–261.
Farner, D. S., and J. Kezer. 1953. Notes on the amphibians and reptiles of Crater Lake National Park. The American Midland Naturalist 50:448–462.
Fry, B. 1991. Stable isotope diagrams of freshwater food webs. Ecology 72(6):2293–2297.
Garcia, T. S., D. J. Paoletti, and A. R. Blaustein. 2009. Correlated trait response: Comparing amphibian defense strategies across a stress gradient. Canadian Journal of Zoology 87:41–49.
Geiger W., P. Alcorlo, A. Baltanas, and C. Montes. 2005. Impact of an introduced crustacean on the trophic webs of Mediterranean wetlands. Biological Invasions 7:49–73.
Gherardi, F., L. Aquiloni, J. Diéguez-Uribeondo, and E. Tricarico. 2011. Managing invasive crayfish: Is there a hope? Aquatic Sciences 73:185–200.
Hargreaves, B. R., S. F. Gardiner, M. W. Buktenica, R. W. Collier, E. Urbach, and G. L. Larson. 2007. Ultraviolet radiation and bio-optics in Crater Lake, Oregon. Hydrobiologia 574:107–140.
Hein, C. L., M. J. Vander Zanden, and J. J. Magnuson. 2007. Intensive trapping and increased fish predation cause massive population decline of an invasive crayfish. Freshwater Biology 52:1134–1146.
Henery, R., J. Umek, S. Chandra, and L. Holan. 2012. Benthic composition, biomass, and habitats contributing to zoobenthic consumers in Crater Lake: Emphasizing crayfish populations. Research report submitted to Crater Lake National Park. University of Nevada, Reno, Nevada, USA. 63 pp.
Larson, G. L., R. L. Hoffman, C. D. McIntire, M. W. Buktenica, and S. F. Girdner. 2007. Water quality and optical properties of Crater Lake, Oregon. Hydrobiologia 574:69–84.
Light, T. 2003. Success and failure in a lotic crayfish invasion: The roles of hydrologic variability and habitat alteration. Freshwater Biology 48(0):1886–1897.
Lodge, D. M., and A. M. Hill. 1994. Factors governing species composition, population size, and productivity of coolwater crayfishes. Nordic Journal of Freshwater Research 69:111–136.
Momot, W. T. 1995. Redefining the role of crayfish in aquatic ecosystems. Reviews in Fisheries Science 3:33–63.
Myers, G. S. 1942. Notes on Pacific coast Triturus. Copeia 2:77–82.
Stenroth, P., and P. Nyström. 2003. Exotic crayfish in a brown water stream: Effects on juvenile trout, invertebrates and algae. Freshwater Biology 48(3):466–475.
Stokes, A. N., A. M. Ray, M. Buktenica, B. G. Gall, E. Paulson, D. Paulson, S. S. French, E. D. Brodie III, and E. D. Brodie Jr. 2015. Otter predation on Taricha granulosa and variation in tetrodotoxin levels with elevation. Northwestern Naturalist 96(1):13–21.
About the authors
M. W. Buktenica, S. F. Girdner, and D. K. Hering are with Crater Lake National Park, Oregon. A. M. Ray is with the NPS Greater Yellowstone Network, Bozeman, Montana. J. Umek is with the Aquatic Ecosystems Analysis Laboratory, Department of Biology, University of Nevada, Reno. Please direct correspondence to Mark Buktenica.
Documentation
Suggested citation for this article
Buktenica, M. W., S. F. Girdner, A. M. Ray, D. K. Hering, and J. Umek. 2015. The impact of introduced crayfish on a unique population of salamander in Crater Lake, Oregon. Park Science 32(1):5–12.
This article published
Online: 4 September 2015; In print: 14 September 2015
URL
https://www.nps.gov/ParkScience/articles/parkscience32_1_5-12_buktenica_et_al_3815.htm
This page updated
16 September 2015
Site navigation
Last updated: August 9, 2018