Stress hormone metabolites found in fecal samples are used increasingly to evaluate stress in sensitive populations of birds and mammals.… Glucocorticoid metabolites (GCMs) can be detected in the excrement of birds and mammals.
Get PDF
Download PDF of this article
Abstract and key words
Abstract: American pikas are widely considered a sentinel species for detecting ecological effects of climate change. They are declining within a large portion of their range, and the National Park Service (NPS) recently initiated a research program, Pikas in Peril (PIP), which is designed to assess pika vulnerability to predicted changes in climate. As part of the PIP program, we collected fresh fecal samples from eight western national parks. We also tested fecal sample storage techniques using feces collected from pikas that were live-trapped, thus validating storage protocols established by NPS surveyors. Finally we measured physiological stress (glucocorticoid metabolites, GCM) in pikas in parks using fecal samples collected non-invasively. Here we present baseline values of GCM concentration for pikas across the western United States, which can be used to identify changes in physiological stress levels for this climate-sensitive species. Our research contributes to the understanding of climate change effects on this sentinel mammal, and provides park managers with baseline information that can be incorporated into long-term monitoring studies.
Key Words: American pikas, climate change, Crater Lake National Park, Craters of the Moon National Mounument and Preserve, Grand Teton National Park, Lassen Volcanic National Park, Lava Beds National Monument, non-invasive sample collection, Pikas in Peril program, Rocky Mountain National Park, stress physiology, Yellowstone National Park
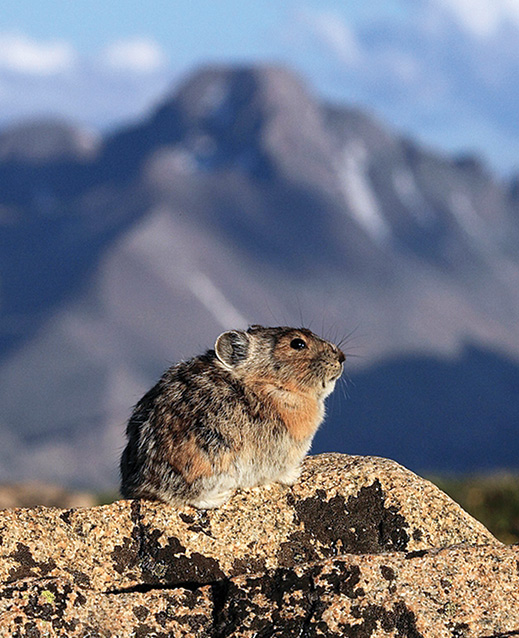
Copyright Dick Orleans
AMERICAN PIKAS (OCHOTONA PRINCEPS) ARE CHARISMATIC INHABITANTS of mountainous and rocky areas in many national parks of the western United States (fig. 1). These cold-adapted members of the rabbit order, commonly known as rock rabbits, are sensitive to warmer temperatures and rely on access to cooler microclimates located underneath rocky habitats, such as talus (Hafner 1993). As a result of recent climate-mediated population declines and extirpations, pikas have become widely considered a sentinel species for detecting ecological effects of climate change (Beever et al. 2011). Projections suggest that populations will continue to decline (Ray et al. 2012), and the species has been considered for listing as threatened or endangered at the state and federal levels. Interest in documenting changes in pika distribution is growing; in response the National Park Service (NPS) recently funded a research program, called Pikas in Peril (PIP), designed to assess vulnerability of pikas in national parks to future climate scenarios.
The PIP program is a collaborative research effort among multiple university researchers and park staff from eight western national parks (fig. 2, below). The main goals of the program are to document pika occurrence patterns and distribution, measure gene flow and connectivity of populations, and predict impacts of future climate change on pika populations (Garrett et al. 2011). Occupancy surveys were conducted in eight western parks in 2010 and 2011. Survey crews recorded evidence of pika presence or absence at dozens of plots within each park each year, and also evaluated pika habitat and collected fresh fecal pellets for DNA analysis. Pikas are ideal candidates for occupancy surveys because their presence is easy to detect. Data generated from these studies are being used to relate pika occupancy to habitat characteristics (Jeffress et al. 2013), which will allow park managers to identify prime habitat and predict the future distribution of the species under climate-change scenarios (Garrett et al. 2011).
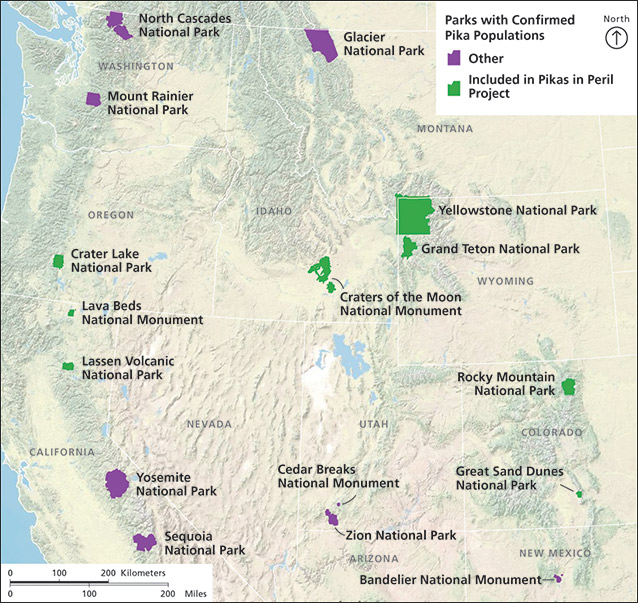
Meghan Lonneker and Gordon Dicus, NPS Upper Columbia Basin Network
However, patterns of pika presence and absence such as used in the PIP study may be confounded by transient processes and source-sink dynamics. For example, pika populations tend to be small because habitat patches are small and pika territories are largely nonoverlapping (Smith and Weston 1990). These small populations may experience temporary extirpation because of a sequence of random and unrelated deaths (demographic stochasticity) or a rare event (environmental stochasticity). These stochastic extinctions can occur in otherwise suitable habitats (Morrison and Hik 2007), obscuring the relationship between habitat quality and pika presence. Extinctions caused by poor habitat quality can also create a bias in occupancy data if the species easily recolonizes poor-quality “sink” patches through dispersal from high-quality “source” patches. Such source-sink dynamics can make a poor-quality habitat appear suitable, while stochastic extinctions make suitable habitats appear unsuitable. To supplement presence-absence data and to predict trends in occupancy that may not yet be apparent, it would be useful to have data on the physiological response of pikas to their local habitats.
One method for identifying potential stressors directly affecting individuals is to measure physiological stress. Stress hormone metabolites found in fecal samples are used increasingly to evaluate stress in sensitive populations of birds and mammals. In response to a stressor, animals release glucocorticoid hormones (such as cortisol or corticosterone) into the bloodstream. These hormones circulating in the body are metabolized by the liver and then excreted as metabolites into the gut. Glucocorticoid metabolites (GCMs) can be detected in the excrement of birds and mammals (Möstl and Palme 2002).
Fecal samples can be collected relatively easily and non-invasively, important considerations for a species in decline such as pikas. Of course, animals must be handled to validate the use of GCMs as a metric of stress, but non-invasive sampling is possible following validation. Validation should address not only the relationship between stress and GCM in samples collected non-invasively, but also the potential influence of environmental factors acting directly on GCM concentration in those samples after deposition. Environmental conditions such as temperature or humidity can alter microbial activity, which can result in increased decomposition of steroid metabolites and biased measurements of metabolite concentration (Millspaugh and Washburn 2004). Therefore, accurately comparing GCM concentrations from samples collected across the western United States may be difficult, since environmental conditions can vary considerably in different regions. Similarly, sample storage techniques have been found to alter GCM concentration in fecal samples (Khan et al. 2002), and must be tested to interpret results reliably.
Here we present a practical application of recently developed techniques for non-invasively measuring stress in pikas, using fresh fecal samples that were collected as part of the PIP program. In this study, our first objective was to examine the effects of storage methods on stress hormone metabolite concentration (GCM) in pika fecal pellets. By comparing GCM concentration measured in control samples with that in samples that were placed in storage envelopes for varying amounts of time, we tested whether storage methods directly alter GCM concentrations measured in samples. Our second objective was to non-invasively measure physiological stress (GCM concentration) in pikas living in national parks throughout the western United States. We present baseline values of GCM concentration for pikas in eight national parks, which can be used to identify future changes in physiological stress levels for this climate-sensitive species. Finally, we provide additional information regarding how our established techniques can contribute to long-term monitoring and management programs related to pikas and other NPS key vital signs.
Methods
Testing Effects of sample storage (objective 1)
To obtain fecal samples, we took advantage of an ongoing demographic study of pikas at the Niwot Ridge Long-Term Ecological Research Site in Colorado. Fresh fecal pellets were collected from live-trapped, anesthetized pikas as a by-product of determining each animal’s sex, because manipulations required to observe the genitals often result in fecal pellet excretion. Additional pellets were often collected from inside the trap or anesthetizing chamber, or from the rock that had supported the (wire-mesh) trap. Samples were collected from individuals that had been in traps for less than 3 hours, which ensured that measured GCM concentration represented a baseline level (GCM increase in response to stress does not occur until 10–15 hours after initiation of a stress-inducing event, such as trapping) (Wilkening et al. 2013). Trapping and sampling procedures were reviewed and authorized by Colorado Parks and Wildlife (license TR2014), and procedures followed those approved by the University of Colorado–Boulder Institutional Animal Care and Use Committee (protocol 1104.06). Fecal pellets used for this study were from adult female pikas, to control for observed differences in GCM concentration resulting from gender and age, and each female was released where captured within 15 minutes after sampling. Fecal samples were placed into storage tubes, kept on ice in the field, and transferred within 12 hours to a −20°C (−4°F) freezer. Pellets collected from six individuals were pooled and divided at random into 14 samples: two controls and 12 samples slated for experiment. Each sample consisted of approximately nine pellets, for an average of 21 pellets per pika, placed inside a small paper envelope (coin envelope). Envelopes were kept at room temperature in a dry location, and storage methods mimicked those already in use by NPS staff and trained project volunteers (Jeffress and Garrett 2011). Each envelope was stored for a different number of months (1–12), and envelopes were numbered according to storage time (for example, envelope 1 = one month, 2 = two months). After each specified time period, samples were removed from envelopes and placed in storage vials in a −20°C freezer. Control samples were maintained in a -20°C freezer during the entire period and GCM analysis was performed on all samples at the same time.
We extracted GCM using protocols previously validated for pikas (Wilkening et al. 2013). Comparative analysis of GCM concentration in samples was conducted using a commercially available Corticosterone Enzyme Immunoassay Kit (Arbor Assay Design, Inc., Ann Arbor, MI; catalog number K014-H1). Final concentrations of fecal GCM were expressed as ng GCM/g (or parts per billion, ppb) dry feces. GCMs were extracted using all pellets from each envelope, and extractions from each envelope were assayed in duplicate. We used duplicate extractions to calculate the mean GCM concentration for each sample, and related these means to sample storage times using a linear regression analysis. Linear regression assumes a straight-line relationship between the response variable (GCM concentration) and the predictor variable (storage time), and requires that the residuals (error or scatter of data points around the regression line) be normally distributed with no trend or autocorrelation related to the predictor variable. We examined residuals, residual autocorrelation, and a normal probability plot to confirm the validity of these assumptions. We assumed there was a significant linear relationship between GCM concentration and storage time if the probability of no relationship between these variables was less than 0.05. All statistical analyses were conducted using R 3.0.1 statistical software (R Core Team 2013).
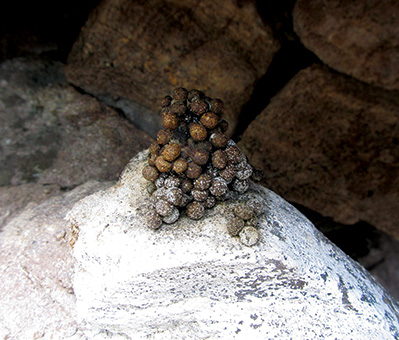
Copyright Johanna Varner
Comparing pika stress hormone levels among parks (objective 2)
Survey crews collected fresh scat samples from pika-occupied habitats in each of eight National Park System units included in the PIP research program: Crater Lake National Park, Oregon; Craters of the Moon National Monument and Preserve, Idaho; Grand Teton National Park, Wyoming; Great Sand Dunes National Park and Preserve and Rocky Mountain National Park in Colorado; Lassen Volcanic National Park and Lava Beds National Monument in California; and Yellowstone National Park in Montana, Idaho, and Wyoming (see fig. 2). Habitats within these parks represent a broad range of those preferred by American pikas, including high-elevation talus slopes and boulder fields and lower-elevation lava flows underlain by ice features. Permits for fecal pellet sampling were obtained for each park in each year of the study, as directed in the PIP protocol (Garrett et al. 2011). Surveyors collected samples within and en route to sites randomly selected for pika occupancy surveys during 2010 and 2011. Collection protocols followed those established by Jeffress and Garrett (2011), and fresh fecal pellets were collected only from currently occupied pika territories as evidenced by a fresh hay pile or active latrine. Pikas maintain established latrines within territories and fecal pellets can be identified as fresh by color, consistency, and relative positioning (fig. 3). Surveyors in all parks attended group trainings that described collection and storage protocols, and were also given detailed field manuals for reference. Fecal pellets were transferred into coin envelopes using twigs or similar devices to avoid human contamination. Each envelope contained 8–14 pellets, and GCM concentrations were determined from 8 to 35 envelopes per park. Envelopes were labeled with the collection date, time, and location (GPS coordinates) and sealed with tape or stickers. To ensure that each sample represented an individual pika, we did not analyze samples that were collected within 50 meters (164 ft) of each other. We took care to prevent pellets from being crushed, and kept collection envelopes in a cardboard box or similar receptacle. Samples were kept at room temperature in a dry environment and later mailed to the University of Colorado in Boulder, where GCM extraction and comparison procedures followed those detailed above. One-way ANOVA (analysis of variance) was used to test for differences between GCM concentrations (adjusted for storage time) among parks.
Results
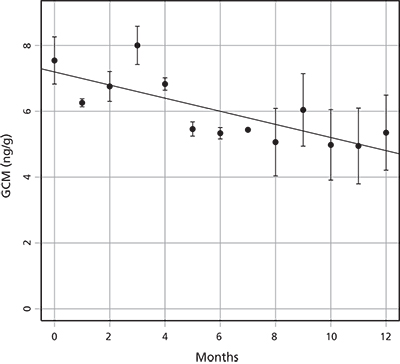
Testing effects of sample storage (objective 1)
Concentration of GCM was strongly affected by storage conditions. GCM concentration fell 4% per month and 33% per year on average in samples stored dry at ambient temperatures, relative to controls stored at −20°C (fig. 4). These results indicate that samples should be stored in the freezer immediately following collection; alternatively, the expected reduction in detectable GCM should be accounted for. In our experiment, there was a linear reduction in GCM concentration y, with the number of months, m, that a sample was stored unfrozen (y = 7.19 – 0.20m, p < 0.001, adjusted r² = 0.56). We used this relationship to estimate the pre-storage GCM concentration in each sample, given the number of months the sample was stored unfrozen.
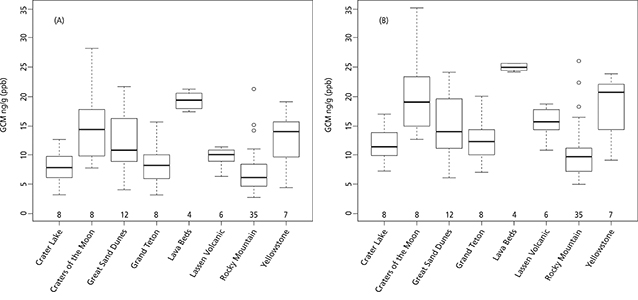
Comparing pike stress hormone levels among parks (objective 2)
Using either observed or adjusted estimates, we found considerable variation in GCM concentration among parks (fig. 5). In the observed data, mean GCM concentration was lowest at Rocky Mountain (7.13 ng/g = nanograms of GCM per gram of feces or 7.13 ppb) and highest at Lava Beds (19.3 ng/g or ppb). There was also less variation in GCM measurements at Lava Beds, with most values being in the higher range (17.4–21.2 ng/g or ppb, table 1). In contrast, the range of GCM values recorded at several other parks was large, such as at Craters of the Moon (7.7–28.8 ng/g or ppb) and Rocky Mountain (2.8–21.2 ng/g or ppb, table 1). The pattern of relative GCM concentration among parks was similar in data observed and adjusted for time in unfrozen storage, but adjusted concentrations averaged 4 units higher than observed (mean concentrations were 9.87 and 13.86 ng/g or ppb in observed and adjusted data, respectively). ANOVA results showed a highly significant effect of park on adjusted GCM (F [7, 80] = 9.28, p-value < 0.001), indicating that adjusted GCM concentration differed significantly among parks.
Table 1. Comparison of stress hormone metabolite concentration adjusted for storage time (adjusted GCM) and environmental characteristics at eight western national parks | |||||
---|---|---|---|---|---|
Park | Adjusted GCM ng/g (ppb) | Elevation | Precipitation | ||
m* | ft | cm* | in | ||
Crater Lake National Park | 11.8 (± 1.1) | 2,115 (1,701–2,530) |
6,939 (5,581–8,268) |
156.7 (104.5–183.1) |
61.7 (41.1–72.1) |
Craters of the Moon National Monument and Preserve | 20.3 (± 2.6) | 1,700 (1,511–1,833) |
5,577 (4,957–6,014) |
32.7 (23.1–37.4) |
12.9 (9.1–14.7) |
Grand Teton National Park | 12.5 (± 1.4) | 2,815 (2,090–3,635) |
9,236 (6,857–11,926) |
171.9 (95.9–235.5) |
67.7 (37.8–92.7) |
Great Sand Dunes National Park and Preserve | 15.1 (± 1.6) | 3,427 (2,647–3,987) |
11,243 (8,684–13,081) |
86.2 (38.9–104.1) |
33.9 (15.3–41.0) |
Lassen Volcanic National Park | 15.5 (± 1.1) | 2,282 (1,731–3,089) |
7,487 (5,679–10,135) |
218.0 (85.0–308.4) |
85.8 (33.5–121.4) |
Lava Beds National Monument | 25.0 (± 0.3) | 1,463 (1,249–1,717) |
4,800 (4,098–5,633) |
43.3 (31.2–58.0) |
17.0 (12.3–22.9) |
Rocky Mountain National Park | 10.4 (± 0.8) | 3,462 (2,572–3,795) |
11,358 (8,438–12,451) |
98.4 (45.2–130.8) |
38.7 (17.8–51.5) |
Yellowstone National Park | 18.1 (± 2.1) | 2,424 (1,651–3,088) |
7,953 (5,417–10,131) |
94.5 (33.2–140.4) |
37.2 (13.1–55.3) |
*Data from Jeffress et al. 2013. Notes: Means are given, followed by the standard error (for GCM concentration) or the range of values (for elevation and precipitation) measured from each park. Annual precipitation data were obtained from the PRISM Web site (www.prism.oregonstate.edu), which provides grid-based estimates at 800-meter resolution for years 1971–2000 using parameter-elevation regressions on independent slope models. |
Discussion and management implications
Fecal samples differed among parks in a common metric of stress, fecal GCM. Exposure to uncontrolled ambient conditions during storage affected observed GCM concentration, but there was a strong linear relationship between GCM concentration and storage time. This relationship was used here in a preliminary attempt to adjust for storage time and estimate GCM concentration prior to storage. In some cases these estimates involved extrapolation beyond the range of the observed relationship between GCM and storage time, requiring caution in the interpretation of results. Further experiments will be necessary to determine whether the linear relationship observed here extends to situations where initial GCM concentrations are higher and storage times longer than in the current experiment. Given this caveat, alternatives to estimation may be preferred. Exposure to uncontrolled ambient conditions could be avoided if samples were collected fresh and immediately stored frozen. This could be accomplished if pika latrines were located, cleaned of accumulated pellets, and revisited for sampling within a few days.
Observed and adjusted estimates of GCM concentration resulted in similar ranking of parks in terms of the degree and range of putative pika stress. We propose several hypotheses that could explain variation in GCM concentration observed both within and among parks. First, the range of GCM values measured in each park includes samples collected from adult male and female pikas. Concentration of GCM has been shown to vary considerably between the sexes, with measurements in samples collected from males being almost twice as high (Wilkening et al. 2013). If sample sizes are small and collection localities do not encompass large geographic areas, GCM values within an area could reflect only males or females, leading to skewed larger or smaller overall GCM concentrations. Second, temperature and precipitation or humidity regimes are not the same across different parks, and these factors can influence GCM concentration in samples collected non-invasively. Differing environmental factors (such as increased temperature or precipitation) can alter GCM concentration in feces, which can occur when samples are not collected in the same ecoregion and same season. For example, table 1 displays the large range of precipitation and elevation values measured in each park. Elevation is often considered a proxy variable for temperature, with lower-elevation areas correlating with higher temperatures. Average GCM concentration is highest in parks with lower-elevation habitat (Craters of the Moon and Lava Beds, table 1), indicating that high temperatures may be increasing GCM concentration in exposed samples, or pikas subject to higher temperatures may be experiencing higher stress. Diet can alter GCM concentration, since hormones pass through the digestive system (Touma and Palme 2005). Pikas are generalist herbivores and feed primarily on locally abundant vegetation, which varies by region and among parks. Other factors that may vary among parks and contribute to higher levels of pika stress include predation pressure and human disturbance impacts (such as proximity to roads or trails). Additional analyses investigating the influence of habitat characteristics specific to each park may help to explain the variation we observed.
This is the first study to present multiregional values of a physiological stress metric measured in pikas, an important indicator species occurring in a large number of western parks. Additionally, the spatial extent of our research is unique, since there are no other studies measuring physiological stress in a species across such a large and varied geographic area. Samples collected non-invasively should represent baseline values of GCM concentration in each park. Previous research has shown that exposure to environmental conditions does not influence GCM concentration if samples are collected within a single ecoregion during the same season (Wilkening et al. 2015). Pika fecal samples are typically collected during the summer months (because of weather constraints), and ecoregions do not vary within parks. Thus, park managers can use baseline values given here (based on our adjusted estimates) to document any changes in this stress metric over time measured in a particular park. Results from our study add a vital physiological component to methods of estimating population vulnerability, helping to improve our understanding of the potential for effects of climate change on this species.
Acknowledgments
Special thanks go to the numerous field assistants, NPS staff, and project volunteers who collected samples for this study as part of the PIP program. We also thank Jessica Castillo for organizing and sending fecal samples, Riley Stuckey for his help in the laboratory with sample analysis, and Rebecca Safran for the use of laboratory equipment. Funding for this research was provided by a National Park Service George Melendez Wright Climate Change Fellowship. Support for this project also came from the National Park Service Climate Change Response Program and the U.S. Geological Survey Park-Oriented Biological Support (POBS) Program (grant G11AC20397).
References
Beever, E. A., C. Ray, J. L. Wilkening, P. F. Brussard, and P. W. Mote. 2011. Contemporary climate change alters the pace and drivers of extinction. Global Change Biology 17:2054–2070.
Garrett, L., M. Jeffress, M. Britten, C. Epps, C. Ray, and S. Wolff. 2011. Pikas in Peril: Multiregional vulnerability assessment of a climate-sensitive sentinel species. Park Science 28(2):16–17, 95.
Hafner, D. J. 1993. North American pika (Ochotona princeps) as a Late Quaternary biogeographic indicator species. Quaternary Research 39:373–380.
Jeffress, M., and L. Garrett. 2011. Pika monitoring under way in four western parks: The development of a collaborative multipark protocol. Park Science 28(2):18–19, 95.
Jeffress, M. R., T. J. Rodhouse, C. Ray, S. Wolff, and C. W. Epps. 2013. The idiosyncrasies of place: Geographic variation in the climate-distribution relationships of the American pika. Ecological Applications 23:864–878.
Khan, M. Z., J. Altmann, S. S. Isani, and J. Yu. 2002. A matter of time: Evaluating the storage of fecal samples for steroid analysis. General and Comparative Endocrinology 128:57–64.
Millspaugh, J. J., and B. E. Washburn. 2004. Use of fecal glucocorticoid metabolite measures in conservation biology research: Considerations for application and interpretation. General and Comparative Endocrinology 138:189–199.
Morrison, S. F., and D. Hik. 2007. Demographic analysis of a declining pika Ochotona collaris population: Linking survival to broad-scale climate patterns via spring snowmelt patterns. Journal of Animal Ecology 76:899–907.
Möstl, E., and R. Palme. 2002. Hormones as indicators of stress. Domestic Animal Endocrinology 23:67–74.
R Core Team. 2013. R: A language and environment for statistical computing. R Foundation for Statistical Computing, Vienna, Austria. Available at http://www.R-project.org.
Ray, C., E. Beever, and S. Loarie. 2012. Retreat of the American pika: Up the mountain or into the void? Pages 245–270 inJ. F. Brodie, E. Post, and D. F. Doak, editors. Wildlife conservation in a changing climate. University of Chicago Press, Chicago, Illinois, USA.
Smith, A. T., and M. L. Weston. 1990. Ochotona princeps. Mammalian Species 352:1–8.
Touma, C., and R. Palme. 2005. Measuring fecal glucocorticoid metabolites in mammals and birds: The importance of validation. Annals of the New York Academy of Sciences 1046:54–74.
Wilkening, J. L., C. Ray, and K. L. Sweazea. 2013. Stress hormone concentration in Rocky Mountain populations of the American pika (Ochotona princeps). Conservation Physiology 1:1–13.
Wilkening, J. L., C. Ray, and J. Varner. 2015. Relating sub-surface ice features to physiological stress in a climate sensitive mammal, the American pika (Ochotona princeps). PLoS ONE 10(3):e0119327. doi:10.1371/journal.pone.0119327.
About the authors
Jennifer L. Wilkening is a former recipient of an NPS George Melendez Wright Climate Change Fellowship, and conducted this research while a PhD student at the University of Colorado, Boulder. Chris Ray is a research associate at the University of Colorado, Boulder.
Documentation
Suggested citation for this article
Wilkening, J. L., and C. Ray. 2015. Parks, pikas, and physiological stress: Implications for long-term monitoring of an NPS climate-sensitive sentinel species. Park Science 32(1):42–48.
This article published
Online: 4 September 2015; In print: 14 September 2015
URL
https://www.nps.gov/articles/parkscience32_1_42-48_wilkening_ray_3820.htm
This page updated
16 September 2015
Site navigation
Tags
- crater lake national park
- craters of the moon national monument & preserve
- grand teton national park
- lassen volcanic national park
- lava beds national monument
- rocky mountain national park
- yellowstone national park
- park science journal
- ps v32 n1
- research report
- american pika
- climate change
- crater lake national park
- craters of the moon national monument and preserve
- grand teton national park
- lassen volcanic national park
- lava beds national monument
- non-invasive sample collection
- pikas in peril program
- rocky mountain national park
- stress physiology
- yellowstone national park
- climate
- animals
- ecology
- zoology
- wildlife
Last updated: March 21, 2021