Last updated: August 25, 2017
Article
Measurement of Glacial Meltwater Outflow through Water Analysis for Geohazard Recognition
IMPORTANCE
Predicting the onset of jökulhlaups is difficult due to a lack of understanding of the signals of glacial meltwater stagnation and conditions that may lead to outburst flooding. Understanding the causes for glacial meltwater storage, and measuring the flow of jökulhlaups would provide researchers important information for determining conditions that may lead to events. Studying the englacial and subglacial channeling systems in various geological settings may afford useful information for areas currently experiencing jökulhlaups, potentially offering information useful for predicting the magnitude and relative time of future events. Measuring glacial meltwater output during periods of normal activity and event-causing activity would be useful for further understanding the mechanisms that cause jökulhlaups.
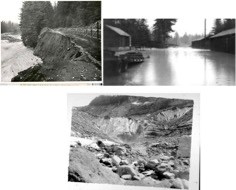
Photos attained from Archives in Longmire Library, Mount Rainier National Park.
STATUS AND TRENDS
The Nisqually Glacier in Mount Rainier National Park has a lengthy history of jökulhlaups, many of which occurred in the mid to late 1900’s (Figure 1). The goal of this project was to do a complete survey of water chemistry associated with glacial meltwater to find predictors of flow and thus potential signals of water retention. A geochemical analysis of the Nisqually Glacier was conducted by collecting snow, ice, water, and debris samples along the glacier. Surface ice, deep crevasse ice, Nisqually River water, surface stream water, water from a flooded crevasse, and surface debris were amongst these samples. Items from the summit (hoarfrost, fumarole residue, and ice cave snow) were also collected and analyzed as a geochemical baseline for Mount Rainier. Ice, snow, and water samples from the Emmons glacier were collected as a control variable against the Nisqually. Snow samples were also collected from the Paradise Glacier to compare with the Nisqually. All samples were collected following NPS sampling protocol. Sampling sites were strategically chosen to observe how the measured chemical signals diluted downstream from the Nisqually terminus by non-glacially fed tributaries. Herein, a signal is defined as a solute that directly emanates from the glacier and could be used to monitor glacial output. A non-signal is a solute dissolved in water that is common in all locations on the mountain, or ubiquitous. Water samples collected near the terminus contain
non-diluted signals, as there are no tributaries feeding into the river between the sampling location and the terminus. Three major snowpack tributaries were sampled downstream to determine which signals were representatives, or non-representatives, of snowpack melt when observing the dilution of glacier signals along the Nisqually River.
Results
There are detectable chemical differences between glacial meltwater and mountain snowpack inlet streams that can be used to monitor glacial outflow. It is hypothesized that iron concentration, turbidity, temperature and pH levels may be used to monitor hydrology of the Nisqually Glacier. A series of field tests (Fe, NO3, hardness, pH/conductivity/ORP/temperature, turbidity) provided preliminary data to compare with further analytical testing in the lab, including inductively coupled plasma-atomic emission spectroscopy (ICP-AES), a bathophenanthroline colorimetric test, and total organic carbon measurements. Analysis showed dissolved iron concentration to be statistically higher in glacial meltwater than in snowpack inlets (t=9.26, p<0.001). Fe levels decreased downstream, presumably due to dilution from snowpack inlet streams. Turbidity followed an equivalent trend, decreasing as the zero-turbidity snowpack melt diluted the river at increasing distances from the glacial terminus. Temperature measurements followed suit by increasing in value along the Nisqually River, presumably due to contributions from warmer snowmelt inlet streams. pH levels were detected at similar values for each of the sampling sites, but according to previous research, a drastic change in pH would indicate a hydrogeological shift in the glacier. Thus, Fe, turbidity, temperature, and pH appear to be effective signals for monitoring glacial output that may be quickly and easily tested using field kits. Utilization of a real-time method to test for stagnation would afford researchers the opportunity to predict jökulhlaups.
Discussion
Based on this work, it is also postulated that glacial meltwater may be an unappreciated source of nutrient Fe in river and ocean ecosystems. Future work recommended includes continued measurements of Fe concentration, temperature, pH, and turbidity along with river flow to understand how these signals fluctuate as river flow changes. Daily measurements should be taken as close to the terminus as practical (i.e. Nisqually Glacier Bridge) to avoid increased dilution of glacial outflow signals. Continued measurements are important for chemical characterization of the Nisqually’s glacial outflow. Correlating these results with ice velocity and/or ice elevation measurements would allot a geochemical profile of the Nisqually Glacier and River.