Last updated: August 28, 2023
Article
Glacier and Perennial Snowfield Research in Rocky Mountain National Park
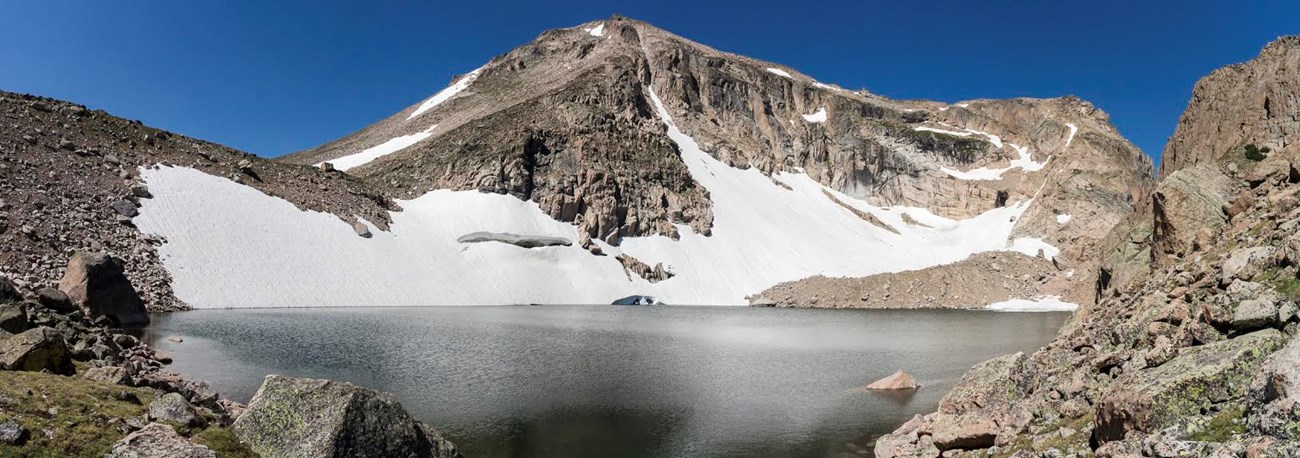
NPS Photo/ J. Westfall
Each year, millions of visitors come to Rocky Mountain National Park (RMNP) to experience its scenic mountainous landscapes, abundant wildlife, and high elevation alpine tundra. Glaciers, perennial snowfields, and ice patches are an important part of these human experiences. Glaciers shaped this landscape, carving the dramatic alpine cirques and aretes and filling valley floors (e.g., Moraine Park) with glacial deposits. Today, meltwater from snow and perennial ice fields/glaciers support the park’s plants and wildlife, as well as human populations on both sides of the Continental Divide (Clow et al. 2016; Fassnacht et al. 2018; Ingersoll et al. 2007; Patterson 2016). The vast blanket of seasonal snow draws many visitors and supports a robust winter recreation economy (Fassnacht et al. 2018; Patterson 2016).
RMNP has been shaped by two glacial periods: the Bull Lake Glaciation (200,000-130,000 years ago) and the Pinedale Glaciation (30,000-11,700 years ago). On U.S. Geological Survey topographic maps, eight masses of snow and ice in RMNP were historically identified as glaciers: Andrews, Dove, Mills, Moomaw, Rowe, Sprague, Taylor, and Tyndall. Under a strict definition, however, Andrews may be the only “true” glacier because the others do not appear to flow. The park contains another 20+ perennial ice/snow patches. Glaciers, perennial snowfields, and ice patches are strongly influenced by climate, particularly air temperature and frozen precipitation. Past and current research has increased our understanding of past climate and air quality. Research is also shedding light on the long-term sustainability of our glaciers, threats to their continued existence, and how we can mitigate those threats.
NPS Photo
Research
Early research on glaciers in and around RMNP focused on historic glacier extent and the timing of glacial retreat. During the Last Glacial Maximum, glaciers filled many major valleys in RMNP and along the Front Range but did not reach Estes Park and Tahosa Valley (Jones and Quam 1944). Research on the history of glaciers also includes the timing of glacial advances and retreats, and climate variations in the Holocene (Benedict 1973; Benedict 1968; Madole 1980; Madole 1976). Benedict et al. (2008) used radiocarbon dates on timber emerging from a melting ice patch to suggest treeline was ~175 m higher during the mid-Holocene and that ice patches are at a minimum extent today relative to the past 4,200 years. Previous work documented at least four periods of Holocene glacier growth, with the most recent during the Arapaho Peak advance (300-100 BP); regional climate change was the primary mechanism behind Holocene glacial change (Benedict 1973). Given their unique topographic setting and outsized role of wind redistribution of snow, the mass balance of glaciers in RMNP today is relatively insensitive to winter accumulation but does respond to spring precipitation and summer temperatures (Hoffman et al. 2007; Outcalt 1965; Olyphant 1985).
Recent research has focused on both modern and long-term changes in snowpack, ice patches, and glaciers. Hoffman et al. (2007) found that glacier extent fluctuated throughout the 20th century, with significant recession between 1999 and ~2005. More recent work by McGrath (2019) found the glaciers exhibited marked interannual variability, but limited net change in area between 2001 and 2018. McGrath used historical topographic maps and modern digital elevation models to calculate glacier volume change over 50-year intervals and found that Andrews and Tyndall were in near balance, whereas Moomaw Glacier had thinned by 3.2 m between 1964 and 2016. Elsewhere along the Front Range, Arapaho Glacier thinned by approximately 23 m over this same interval, suggesting that these glaciers do not respond equally to climate forcings. Topography, aspect, and wind redistribution clearly play an important role in the observed variability. McGrath used ground-penetrating radar to measure the ice thickness of Andrews Glacier along transects and found one section to be over 45 meters thick, making it most likely the thickest glacier in Colorado.
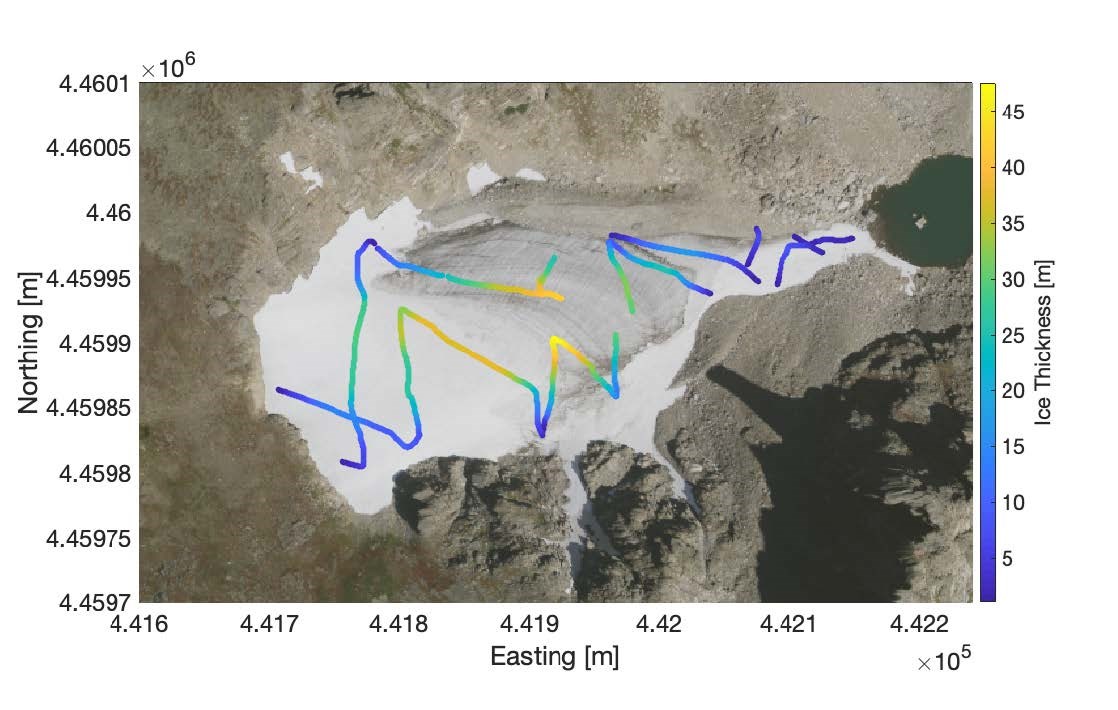
Figure courtesy of D. McGrath.
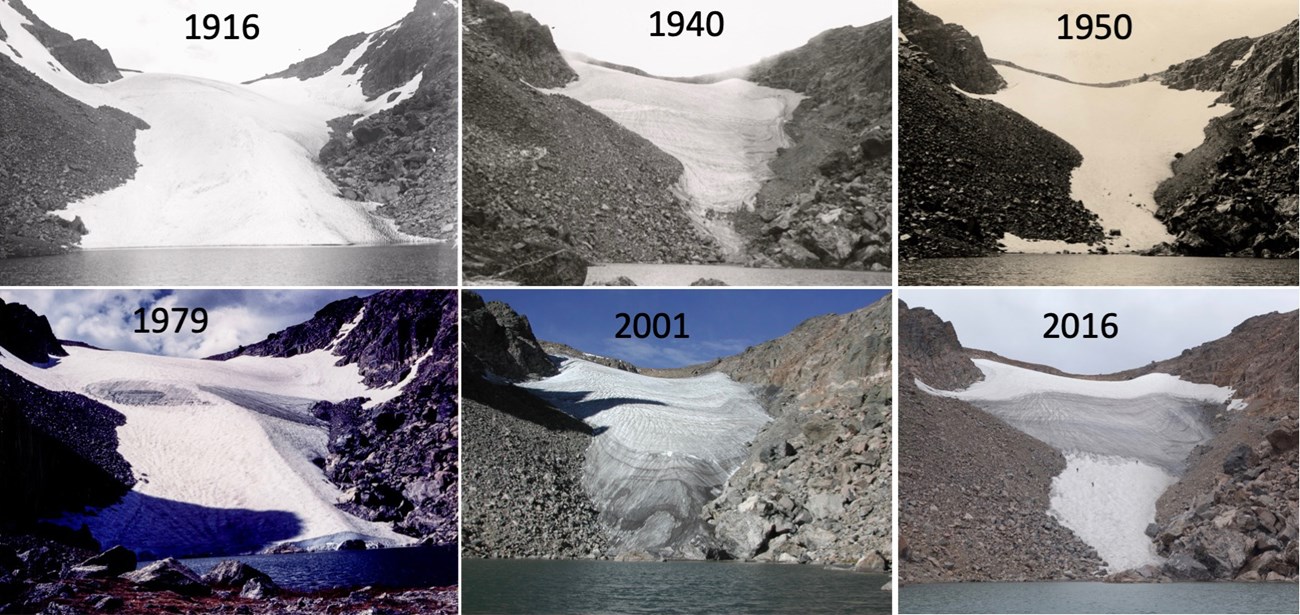
Series courtesy of D. McGrath.
Management
Research at RMNP has increased the understanding of why snowpack, ice patches, and glaciers are important, what is threatening their existence, and what can be done to mitigate those threats. Better agricultural practices (i.e. less grazing, tilling, and chlorinated pesticides) and significant global actions to reduce carbon emissions would mitigate glacier mass loss. Both RMNP and the National Park Service are working to reduce their own carbon footprints, largely by increasing their energy efficiency and encouraging visitors to do the same. The NPS Air Resources Division has also been leading the inter-agency Rocky Mountain National Park Nitrogen Deposition Reduction Plan (2007) and works closely with the park, partner agencies, and the Agricultural Subcommittee to address nitrogen emission from farms and ranches through the development of agricultural best management practices. RMNP encourages climate, glacier, and air quality research; supports long-term monitoring, including the SNOTEL sites within the park; and works with community scientists on a glacier repeat photography project.
References
*Benedict, J.B.; R.J. Benedict; C.M. Lee; and D.M. Staley. 2008. Spruce Trees from a Melting Ice Patch: Evidence for Holocene Climatic Change in the Colorado Rocky Mountains, USA. The Holocene 18(7):1067-1076.*Benedict, J.B. 1973. Chronology of Cirque Glaciation, Colorado Front Range. Journal of Quaternary Research 3(4):584-599.
*Benedict, J.B. 1968. Recent Glacial History of an Alpine Area in the Colorado Front Range, U.S.A. Journal of Glaciology 7(49):77-87.
*Clow, D.W. 2010. Changes in the Timing of Snowmelt and Streamflow in Colorado: A Response to Recent Warming. Journal of Climate 23(9):2293-2306.
*Clow, D.W.; M.W. Williams; and P.F. Schuster. 2016. Increasing Aeolian Dust Deposition to Snowpacks in the Rocky Mountains Inferred from Snowpack, Wet Deposition, and Aerosol Chemistry. Atmospheric Environment 146:183-194.
*Fassnacht, S.R.; N.B. Venable; D. McGrath; and G.G. Patterson. 2018. Sub-Seasonal Snowpack Trends in the Rocky Mountain National Park Area, Colorado, USA. Water 10(5):562.
*Hoffman, M.J.; A.G. Fountain; and J.M. Achuff. 2007. 20th-Century Variations in Area of Cirque Glaciers and Glacierets, Rocky Mountain National Park, Rocky Mountains, Colorado, USA. Annals of Glaciology 46:349-354.Huss M. and R. Hock. 2015. A New Model for Global Glacier Change and Sea-level Rise. Frontiers in Earth Science 3:54.
*Ingersoll, G.P.; M.A. Mast; L. Nanus; H.H. Handran; D.J. Manthorne; and D.M. Hultstrand. 2007. Rocky Mountain Snowpack Chemistry at Selected Sites, 2004. U.S. Geological Survey Open-File Report 2007—1045, 15p.
*Ingersoll, G.P.; J.T. Turk; M.A. Mast; D.W. Clow; D.H. Campbell; and Z.C. Bailey. 2002. Rocky Mountain Snowpack Chemistry Network: History, Methods, and the Importance of Monitoring Mountain Ecosystems. U.S. Geological Survey Open-File Report 01—466, 14p.
*Jones, W.D. and L.O. Quam. 1944. Glacial Land Forms in Rocky Mountain National Park, Colorado. The Journal of Geology LII(4):217-234.
*Madole, R.F. 1980. Time of Pinedale Deglaciation in North-central Colorado: Further Considerations. Geology 8:118-122.
*Madole, R.F. 1976. Bog Stratigraphy, Radiocarbon Dates, and Pinedale to Holocene Glacial History in the Front Range, Colorado. Journal of Research of the U.S. Geological Survey 4(2):163-169.
*Mast, M.A.; W.T. Foreman; and S.V. Skaates. 2006. Organochlorine Compounds and Current- Use Pesticides in Snow and Lake Sediment in Rocky Mountain National Park, Colorado, and Glacier National Park, Montana, 2002–03. U.S. Geological Survey Scientific Investigations Report 2006-5119, 54p.
McGrath, D. 2019. Glacier and Perennial Snowfield Mass Balance of Rocky Mountain National Park (ROMO): Past, Present, and Future. Final Report to Rocky Mountain National Park for Task Agreement P16AC00826. Colorado State University.
National Park Service. 2019. Rocky Mountain Park—Open Data Resources. http://irma.nps.gov/DataStore/Reference/Profile/2259557.
*Olyphant, G.A. 1985. Topoclimate and the Distribution of Neoglacial Facies in the Indian Peaks Section of the Front Range, Colorado, U.S.A. Arctic and Alpine Research 17(1):69-78.
*Outcalt, S.I. 1965. The Regimen of the Andrews Glacier in Rocky Mountain National Park, Colorado, 1957-1963. Water Resources Research 1(2):277-282.
*Patterson, G.G. 2016. Trends in Snow Water Equivalent in Rocky Mountain National Park and the Northern Front Range of Colorado, USA. Dissertation. Colorado State University.Rhoades, A.M.; P.A. Ulrich; and C.M. Zarzycki. 2018. Projecting 21st Century Snowpack Trends in Western USA Mountains using Variable-resolution CESM. Climate Dynamics 50:261-288.
*These and other reports and publications can be found in a collection created through the NPS Integrated Resource Management Applications (IRMA) data store: https://irma.nps.gov/DataStore/Collection/Profile/7665