Last updated: April 24, 2020
Article
Monitoring Paleontological Resources
Monitoring in situ paleontological resources
Jason P. Kenworthy
National Park Service, Geologic Resources Division, Denver, Colorado 80225, USA
Alison L. Mims
National Park Service, Curecanti National Recreation Area, Gunnison, Colorado 81230, USA
Santucci, V.L., Kenworthy, J.P., and Mims, A.L., 2009, Monitoring in situ paleontological resources in Young, R., and Norby, L., Geological Monitoring: Boulder, Colorado, Geological Society of America, p. 189–204, doi: 10.1130/2009.monitoring(08). For permission to copy, contact editing@geosociety.org. ©2009 The Geological Society of America. All rights reserved.
INTRODUCTION
Paleontological resources (fossils) are the remains of life preserved in a geologic context. Fossils may consist of the physical remains of a biological organism such as a tooth, bone, shell, leaf, cone, seed, or wood. Evidence of biological activity may sometimes be preserved in rocks, hence tracks, traces, burrows, coprolites (fossil dung and feces), and nests represent other valuable types of paleontological resources.
The fossil record reveals an extremely rich diversity of ancient organisms preserved in the Earth’s strata (layers of rock). The fossil record of life on Earth spans nearly every period of geologic history from Precambrian bacteria, billions of years old, to more recent Pleistocene or Holocene cave fossils dating back only a few thousand years. Together, fossils from around the world yield significant scientific and educational information regarding the history of life on Earth.
From the moment of death for an organism, a wide array of agents and forces work toward the breakdown and destruction of its physical remains. Raup and Stanley (1978) identify and describe biological, chemical, and mechanical variables associated with the destruction of biological remains. Only a very small percentage of all living organisms become part of the fossil record, and of those, an even smaller percentage have been discovered, collected and studied. The preservation of fossils and the associated biases in the fossil record are directly attributable to the organism’s morphologic composition, rate of post-mortem burial, environment of deposition, diagenetic variables, taphonomic (after death) factors, and the post-depositional geologic history of the area.
Despite how common or rare a particular species of fossil may be, all paleontological resources are nonrenewable. Whether fossils occur in situ beneath the surface, exposed at the surface, or removed from strata through collection, these resources are finite, a fact which should be reflected in paleontological resource management strategies. Additionally, the distribution of paleontological resources within a given area is generally not uniform. Fossils frequently occur in local concentrations within lithologic units (Shipman, 1981). This non-uniform distribution of fossils presents the fundamental challenge in their in situ management and protection.
The documentation of baseline paleontological resource data is essential in order to develop effective strategies for monitoring the stability and condition of fossil localities. A resource inventory should be designed in order to compile data regarding the scope, significance, and distribution of fossils at each locality. The spatial and surficial distribution of fossiliferous exposures and strata should always be determined and mapped during paleontological resource inventories. A documented paleontological locality is a spatially defined area that may include either in situ fossils or a site from which fossils have been previously collected and curated into a museum repository. Field documentation for each paleontological locality should include a site description, photography, and some level of mapping (Fremd, 1992). It is important to recognize that new localities may be discovered, and previously documented sites may be lost due to natural or human impacts.
Additionally, during the inventory phase, an assessment of the existing or emerging threats (stressors) to the stability of in situ fossils should be undertaken and documented. This is essential baseline information in order to develop and initiate the appropriate design for paleontological resource monitoring. Some examples of important measurable baseline paleontological locality information include: (1) lithology (physical/chemical properties) of fossiliferous strata (hardness, solubility, bedding, jointing, etc.); (2) relationship of overlying and underlying strata (rock falls, undercutting, sinkholes); (3) degree of slope of the fossiliferous strata; and (4) type and percentage of vegetation cover.
Some land management agencies have developed strategies to document baseline paleontological resource data. In 2006 the National Park Service Inventory and Monitoring Program funded a multi-year project to undertake baseline paleontological resource inventories for parks throughout the agency. Other valuable sources for paleontological resources data include the U.S. Geological Survey (USGS), state geological surveys, local museums, and universities.
STRESSORS AND THREATS
The scientific and educational values of in situ fossils are directly dependent upon their stability. Various benchmarks can be established to determine or define a stable paleontological resource. Some land managing agencies generate a benchmark referred to as a “desired future condition” to defi ne resource management goals. These goals are often expressed as a qualitative description of the resource condition. Below is a proposed benchmark to define a paleontological resource in good condition.
Paleontological resources and their associated geologic context are maintained in situ in a stable environment and are in good condition with little or no potential for degradation or impairment by artificially accelerated natural processes or direct human impacts. Similarly, the scientific and educational values are unimpaired and are also preserved in good condition.
A wide range of natural physical, chemical, and biological processes, along with human access and activities, may threaten the stability of in situ paleontological resources. Santucci and Koch (2003) present an overview of these natural and human related stressors to paleontological resources. The following section identifies some of the natural processes and human factors that influence the stability of in situ paleontological resources. Figure 1 presents a conceptual diagram of these stressors.
Environmental Factors Affecting Stability of In Situ Paleontological Resources
The stability of in situ paleontological resources will always be a function of the natural processes, environmental conditions, and other natural factors at the fossil locality. These natural variables are typically beyond the ability of humans to manage or control, and as natural conditions or processes, should be sustained. For example, natural erosion should not be impeded in order to preserve fossils, except under special circumstances to stabilize a significant locality such as Douglass Quarry at Dinosaur National Monument.
Climatic factors including temperature, precipitation, humidity, and wind can influence rates of weathering and erosion of fossiliferous strata. Both diurnal and annual changes in temperature, particularly when temperatures fluctuate above and below freezing, will cause expansion and contraction of water in rock fractures, contributing to the weathering and erosion of soil, rocks, and fossils. The freeze-thaw index is the number of 24-hour periods per year when the temperature fluctuates above and below freezing. Areas with a high freeze-thaw index would theoretically experience higher rates of weathering and erosion than areas with a low freeze-thaw index. The freeze-thaw index can be a useful variable in determining how often fossil sites should be monitored.
Another climatic factor infl uencing the stability of in situ paleontological resources is precipitation, in the form of rain, snow, hail, sleet, or mist. Rates of erosion are directly proportional to levels and intensity of precipitation. Sites with greater levels of precipitation, or areas with brief (seasonal) periods of intense precipitation, would generally exhibit higher rates of erosion. Therefore, precipitation rates and intensity should also be considered in determining the frequency of monitoring.
Relative humidity or aridity may also influence the stability of in situ paleontological resources. The remains of biological organisms typically experience higher rates of decomposition in areas with high relative humidity. Conversely, rates of decomposition of biological remains may not be as high in arid environments. This relationship is evident in the differential preservation of fossils in arid and humid caves. In arid caves of the southwestern United States, the preservation of soft tissues, hair and dung dating back tens of thousands of years is not uncommon (Santucci et al., 2001b). Similar soft-tissue preservation is generally not found in humid climates.
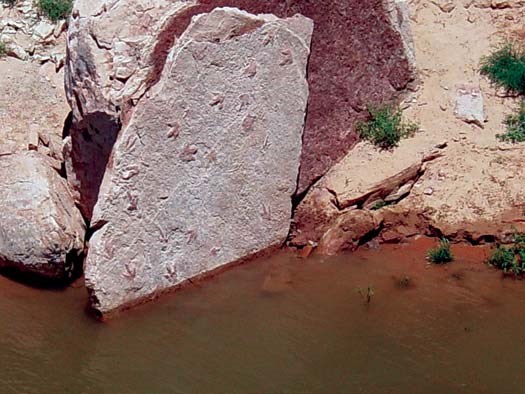
Hydrologic factors such as water depth and changes in water depth of oceans, lakes, ponds, rivers, streams, and other hydrologic features, as well as hydrologic processes such as flooding, drought, tides, waves, currents, and stream velocity, can all significantly influence the stability of in situ fossils (Fig. 2). Increases in water depth may impact paleontological resources during periods of flooding along shorelines and riverbanks. Fossiliferous strata may be subjected to higher than normal rates of erosion due to flooding. Decreases in water depth due to drought or other factors may result in the emergence of previously submerged fossils.
A variety of geologic processes and events may catastrophically influence the stability of in situ paleontological resources. Local or regional volcanism, tectonics, seismicity, glacial activity, landslides, and other geologic factors may present significant threats to fossil localities. These may be direct threats, such as lava burying preserved tree molds, or indirect threats that accelerate local rates of weathering and erosion, such as a loss of vegetative cover due to landslides.
A number of biological factors may influence the stability of in situ paleontological resources. Bioturbation of fossiliferous strata can occur at or beneath the surface. Trampling by ungulates (hoofed animals), burrowing by vertebrates and invertebrates, and root development by trees and other plants can all disturb in situ fossils, either directly or by accelerating local rates of weathering and erosion (Fig. 3). Root development from smaller plants can stabilize soil and other surfi cial deposits, potentially reducing their susceptibility to erosion. Conversely, larger root development can break apart rocks, increasing their susceptibility to erosion. Areas with minimal vegetation cover are generally more susceptible to higher rates of erosion. Numerous factors can affect vegetation cover, and may serve as proxies for potential damage to nearby fossils, including climate/weather, animal grazing/trampling, fi re, visitor use, vegetation management activities, and construction.
Human Factors Influencing Stability of In Situ Paleontological Resources
Within publicly administered lands, the disturbance or removal of fossils by humans is often prohibited by laws, regulations, and policies. In certain cases, fossils may be disturbed or removed by individuals or institutions authorized through a permit, subject to an environmental compliance review, or while performing management actions to preserve or stabilize in situ paleontological resources. Nevertheless, human disturbances may threaten the stability of fossils and can be contrary to theirmanagement goals. Therefore, any monitoring program or prescription for paleontological resources should consider strategies for identifying, understanding, and evaluating the impacts of human factors on fossils.
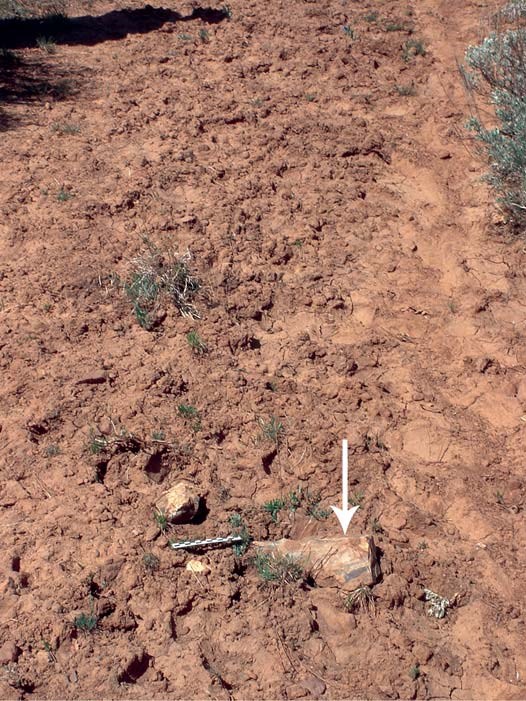
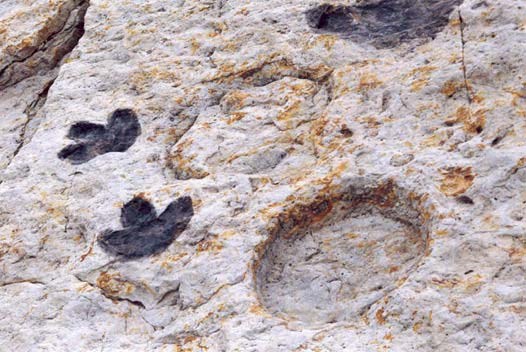
VITAL SIGNS FOR MONITORING IN SITU PALEONTOLOGICAL RESOURCES
Five vital signs are presented here for monitoring of in situ paleontological resources (Table 1). These vital signs exhibit the broadest applicability for the wide diversity of conditions and variables associated with fossil sites. In the future, additional vital signs may be developed for more specific resource conditions. These individual vital signs are not mutually exclusive; rather, there are inherent relationships between vital signs that contribute to an understanding of the stability or instability of in situ fossils.
The vital signs are based on measurable and relevant parameters. Vital signs do not directly measure the “health” of paleontological resources per se, but actually measure variables reflecting their stability in situ. The vital signs discussed in this chapter should help land and resource managers identify thresholds that would trigger the need for some management action or intervention. Monitoring strategies are developed for each vital sign at three levels. Level 1 identifies the simplest and most cost efficient monitoring approach. Level 3 represents a technically sophisticated, but generally most expensive approach. Level 2 is intermediate in cost and complexity. It is important to recognize that the elements of each level are sometimes cumulative through levels 2 and 3.
The use of high resolution photogrammetry is perhaps the most universally valuable approach for monitoring in situ paleontological resource localities. When possible, the establishment of photo points for long-term photography is the best method for measuring both long- and short-term changes.
Vital Sign 5 relates specifi cally to monitoring human-related threats to in situ paleontological resources. Both intentional and unintentional human activities can threaten the stability of fossils. Many of the discussions related to visitor use and resource protection are social science issues; the inclusion of this discussion here enables a more comprehensive approach to monitoring the widest range of threats to in situ fossils.
While not all of the other vital signs may be applicable or practical for every fossil site, collectively the vital signs offer some widely adaptable methods for monitoring in situ fossil sites. Land and resource managers can select the preferred vital signs in order to develop monitoring prescriptions tailored to specific locality or management needs. In most cases, the monitoring of one or more vital signs should provide land and resource managers
with valuable data for planning and decision-making.
Finally, these vital signs have been designed to be implemented by land and resource managers who do not necessarily have a background in geology or paleontology. For managers interested in obtaining more specific geological or paleontological information or expertise, there are a number of resources, including the National Park Service (NPS) Geologic Resources Division, the Geological Society of America (GSA), the U.S. Geological Survey (USGS), state geological surveys, and local universities, museums, and other institutions.
Vital Sign 1: Rates of Natural Erosion I (Geologic Variables)
Description
Rates of natural erosion for fossiliferous strata (rock layers) are influenced by the physical geologic characteristics and properties of the bedrock. Variables such as lithology (physical characteristics of a rock), bedding, degree of slope and geochemistry contribute directly to the resistance or susceptibility to weathering and erosion upon fossiliferous strata exposed at the surface.
The stability of in situ fossils present at or near the surface is proportional to the erosion rates of the exposed strata in which they are preserved. High rates of erosion result in more rapid destruction of fossils exposed at the surface, and low rates of erosion often mean slower destruction. Monitoring rates of erosion at fossil sites will enable land and resource managers to better determine how frequently to schedule site assessments or cyclic prospecting (periodic field investigation of a fossil locality).
Methods
Level 1: Repeat Photography. Differential rates of erosion for fossiliferous strata and the stability of in situ paleontological resources can be assessed through the use of repeat photography. Each fossil site identified through field inventories should be spatially defined, mapped, and photographed. Photo points must be established for both large-scale and small-scale repeat photography so that consistent points of reference and perspectives are available over time. The schedule or frequency of the repeat photography should be determined based upon site-specific variables and management objectives.
All photographs should be maintained in a secure archival storage facility. Each photograph should be labeled with the site name or number, photo point identifier, date of photograph, and name of photographer. Additional site data, such as stratigraphic, taxonomic, or historical information, should be maintained in an associated file or database.
Repeat photography is a low-cost and low-technology approach to monitoring paleontological sites over time. This photography could be undertaken by a trained volunteer. Photos enable the documentation of observable changes in stability at each site,
and the fossils exposed at the surface. This level of information may also enhance resource management and scientific decision making relative to a fossil site, such as the frequency of cyclic prospecting or stabilization of the site.
Level 2: Erosion Monitoring Stakes. Differential rates of erosion for fossiliferous strata and stability of in situ fossils can be assessed through the establishment of erosion monitoring stakes. The stakes are placed into a rock unit perpendicular to the surface. The baseline level is marked directly on the monitoring stake at the point where the stake enters the ground surface. During subsequent site visits, any changes in the ground surface level relative to the monitoring stake should be recorded. Monitoring stakes should be measured at least annually for the first five years after placement (Fig. 5).
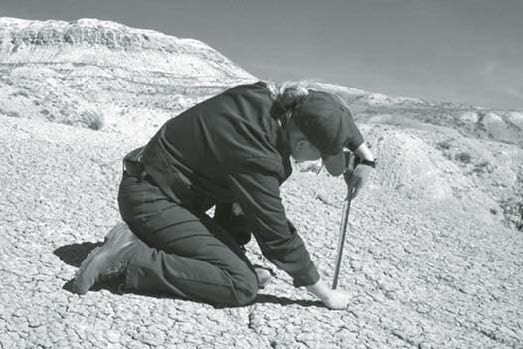
The most durable and environmentally sensitive material to use for the stakes is galvanized steel. The stakes should be at least one meter in length and approximately 0.75 cm in diameter. Initially, the stake should be driven into the substrate until ~15 cm are exposed above the ground surface. A marker flag may be attached to the top portion of the stake to facilitate relocation in the future (and for public safety). If available, a Global Positioning System (GPS) device can be used to record the location of the stakes.
The establishment of erosion monitoring stakes to measure stability of fossiliferous strata is another low-cost and low-technology approach to monitoring fossil sites. These periodic measurements could be undertaken by a trained volunteer. However, the initial placement of erosion monitoring stakes would benefit from the experience of a professional geologist or paleontologist. The acquisition of data from the erosion monitoring stakes will, over time, enable documentation of measurable changes in stability at each site and the fossils exposed at the surface. This level of information may also enhance resource management and scientific decisions, such as the frequency of cyclic prospecting or stabilization of a site.
Level 3: Technology-Enhanced Erosion Monitoring. Differential rates of erosion for fossiliferous strata and stability of in situ paleontological resources can also be assessed through the use of technology-enhanced monitoring equipment. Digital elevation data (topography) is obtained for each site. Geospatial data for the area is also needed in each locality. Locality and elevation data are then used to produce maps differentiating areas of low, medium, and high slopes. Erosion monitoring stakes are installed in sensitive areas and measured annually. A landslide assessment using aerial photographs and geospatial data can also be initiated. This information can be used to determine which areas would be susceptible to higher rates of erosion and would in turn benefit from more frequent monitoring.
The use of technology-enhanced monitoring to measure the stability of fossiliferous strata is a relatively expensive approach to monitoring paleontological sites. The periodic measurements and evaluation of data require a professional geologist and someone trained in the use of the specific technology employed in the monitoring. The acquisition of this data, over time, will enable documentation of measurable changes in the stability at each site and the fossils exposed at the surface. This level of information may also enhance resource management and scientific decisions, such as the frequency of cyclic prospecting or stabilization of the site.
Discussion
The rates of natural erosion and the stability of associated in situ paleontological resources will be the most important factors in determining the frequency of monitoring for this vital sign. Repeat photography and monitoring of erosion stakes should be scheduled at least annually for the first few years. Once monitoring data becomes available, the land or resource manager will be better able to determine if the timing of site monitoring is appropriate or needs adjustment.
A few additional recommendations are worth considering regarding rates of erosion influenced by the geology of the locality:
• Assess surface morphology for each fossiliferous rock exposure. Rock units which are more resistant to weathering and erosion generally develop into more prominent and vertical cliffs, while units more susceptible to weathering and erosion generally develop into more gentle slopes. Rock units more resistant to weathering and erosion require less frequent monitoring.
• Assess differential erosion, as exhibited in surface morphology, between fossiliferous units and the adjacent overlying and underlying strata. Differential erosion contributes to undercutting, rock falls, landslides, slumps, and other forms of geologic hazards which threaten the stability of in situ fossils.
• Assess differential rates of erosion through the use of aerial and ground photographs in order to identify the areas of greatest instability, and areas to target for more frequent cyclic monitoring.
• Estimate the degree of slope or range of slopes for each fossil site using topographic maps or measure slopes in the field with a Brunton compass. Greater slopes are susceptible to higher rates of erosion. Hence, these areas of greater slope should be monitored on a more frequent schedule. Conversely, areas of low or no slope are less susceptible to erosion. Sites occurring at a low slope require less frequent monitoring.
Vital Sign 2: Rates of Natural Erosion II (Climatic Variables)
Description
Rates of natural erosion for fossil strata are infl uenced by climatic conditions and variables. Precipitation and temperature contribute directly to the resistance or susceptibility to weathering and erosion upon fossiliferous strata exposed at the surface. High precipitation promotes higher rates of erosion. Another climatic variable influencing rates of erosion is a measurement referred to as the freeze-thaw index. The freeze-thaw index, as previously explained, is the number of 24-hour periods per year when the temperature fluctuates above and below 32 °F (0 °C).
The stability of in situ fossils present at or near the surface is proportional to the erosion rates of the exposed strata in which they are preserved. Therefore, high rates of erosion will generally result in more rapid destruction of fossils exposed at the surface. Conversely, low rates of erosion often enable slower destruction of exposed fossils. Monitoring rates of erosion at fossil sites will enable land and resource managers to better determine how frequently to schedule site condition assessments or cyclic prospecting.
Level 1: Climatic Records. Local climatic data should be compiled and evaluated, including temperature and precipitation records, for at least a five-year period. Fossil sites in areas of high annual precipitation should be assessed more frequently than areas with low annual precipitation. Local temperature records are evaluated in order to calculate the average number of 24-hour periods per year when the temperature fluctuates above and below 32 °F (0 °C). From this average the freeze-thaw index for the park is determined and incorporated into an appropriate schedule for periodic fossil site assessments. Fossil sites in areas with a low freeze-thaw index require less frequent monitoring than those in areas with a high freeze-thaw index.
Once historic climatic data is compiled, temperature and precipitation data should continue to be recorded for areas with fossil sites. During periods of increased precipitation, and/or increases in the freeze-thaw index, more frequent site assessments may be warranted. This method is a low-cost and low-technology approach to monitoring paleontological sites over time.
Level 2: Repeat Photography. Local climatic data should be compiled and evaluated, including temperature and precipitation records, for at least a five-year period, as outlined in level 1. The freeze-thaw index for the fossil site should also be calculated.
During monitoring, differential rates of erosion for fossiliferous strata and the stability of in situ paleontological resources are assessed using repeat photography. Each fossil site identified through field inventories should be spatially defined, mapped, and photographed. Photo points must be established for both large-scale and small-scale repeat photography so that consistent points of reference and perspectives are available. The schedule or frequency of the repeat photography should be determined based upon climatic variables and management objectives.
All photographs should be maintained in a secure archival storage facility. Each photograph should be labeled with the site name or number, photo point identifier, date of photograph, and name of photographer. Additional site data, such as stratigraphic, taxonomic, or historical information, should be maintained in an associated file or database.
Repeat photography is a low-cost and low-technology approach to monitoring paleontological sites over time. This photography could be undertaken by a trained volunteer. Photos enable the documentation of observable changes in stability at each site,
and the fossils exposed at the surface. This level of information may also enhance resource management and scientific decision making relative to a fossil site, such as the frequency of cyclic prospecting or stabilization of the site.
Level 3: Technology-Enhanced Erosion Monitoring. Local climatic data should be compiled and evaluated, including temperature and precipitation records, for at least a five-year period, as outlined in level 1. The freeze-thaw index for the fossil site should also be calculated.
Differential rates of erosion for fossiliferous strata and stability of in situ paleontological resources can also be assessed through the use of technology-enhanced monitoring equipment. Digital elevation data (topography) is obtained for each site. Geospatial data for the area is also needed in each locality. Locality and elevation data are then used to produce maps differentiating areas of low, medium, and high slopes. Erosion monitoring stakes are installed in sensitive areas and measured annually. A landslide assessment using aerial photographs and geospatial data can also be initiated. This information, combined with local climatic data, can be used to determine which climatic conditions would result in higher rates of erosion, and thus which sites would in turn benefit from more frequent monitoring.
The use of technology-enhanced monitoring to measure the stability of fossiliferous strata is a relatively expensive approach to monitoring paleontological sites. The periodic measurements and evaluation of data require a professional geologist and someone trained in the use of the specific technology employed in the monitoring. The acquisition of this data will, over time, enable documentation of measurable changes in the stability at each site and the fossils exposed at the surface. This level of information may also enhance resource management and scientific decisions, such as the frequency of cyclic prospecting or stabilization of the site.
Discussion
Climatic variables influencing the rates of natural erosion and stability of associated in situ paleontological resources will be the most important factors in determining the frequency of monitoring activities for Vital Sign 2. In areas with high precipitation the rates of erosion would typically be greater. Therefore, monitoring should be more frequent in areas of high precipitation.
Various systems have been established for classifying climatic zones. The Köppen Climate Classification System is the most widely recognized system for classifying world climate. The Köppen system recognizes fi ve major climate types based on annual and monthly averages of temperature and precipitation. These include:
• Moist tropical climates: Climates characterized by year round high temperatures and high levels of precipitation throughout the year.
• Dry climates: Climates characterized by a significant daily temperature range and low levels of precipitation. There are two subgroups: arid/desert and semi-arid/steppe.
• Humid middle latitude climates: Climates characterized by warm/dry summers and cool/wet winters.
• Continental climates: Climates characterized by widely varying seasonal temperatures and moderate to low annual precipitation. This type of climate is typically found in the interior portions of large land masses.
• Cold climates: Climates where the majority of the year experiences below-freezing temperatures. This climate is typical of areas where permanent ice and tundra dominate
the landscape.
Fluctuations in temperature, in particular at temperatures near freezing, may have a significant influence upon rates of erosion and the stability of in situ paleontological resources. The amount of surface and subsurface water varies from arid to humid climates. Fossiliferous rock units saturated with surface water or groundwater will experience more intense freeze-thaw and frost action than those in arid areas.
The concept of a freeze-thaw index is based upon the perspective that areas subjected to increased frost action and freeze-thaw cycles are likely to exhibit higher rates of erosion. Some geographic areas rarely experience temperatures that drop below the freezing point of water. Southern Florida or the Sonoran Desert are examples of areas where the freeze-thaw index would be very low. In other areas, such as the Rocky Mountains or Great Plains, freeze-thaw cycles may be experienced during more than two-thirds of the year, so the freeze-thaw index is much higher.
In areas with a low freeze-thaw index, the rates of erosion based upon temperature fluctuations are probably low. Therefore, fossil sites in these areas would require less frequent monitoring. Conversely, more frequent monitoring would be needed for areas with a high freeze-thaw index.
Vital Sign 3: “Catastrophic” Geologic Processes or Geohazards
Description
A variety of geologic processes and hazards (also known as geohazards) may contribute to the instability of in situ paleontological resources and adversely impact the scientific or educational values of fossils. Geohazards include, but are not limited to, local/regional volcanism, geothermal activity, earthquakes, glacial activity, and mass-wasting events (landslides, slumps, rockfalls, etc). This vital sign would only apply to paleontological resources located in areas at risk for geohazards.
Methods
Level 1: Geohazard Assessment. Potentially catastrophic geohazards which could affect in situ paleontological resources should be identified. Initially, this should be accomplished by researching local geologic resources and processes, review relevant geologic maps and scientific literature, and consulting with professional geologists.
A geohazard assessment is a relatively low-cost and low-technology approach to monitoring paleontological localities. The assessment should be undertaken by a professional geologist. Monitoring of local geohazards which may potentially threaten fossil sites may enhance resource management and scientific decision making.
Level 2: Digital Mapping. Once a geohazard which threatens in situ fossil sites has been identified, more sophisticated monitoring may be warranted. At this level, site monitoring would benefit from the development of digital maps (GIS) integrating spatial fossil distribution with geohazards. The monitoring design would be directly tied to the type of geologic hazard identified.
Digital mapping requires moderate levels of funding for support in addition to a professional geologist who specializes in geohazards.
Level 3: Technology-Enhanced Geohazard Monitoring. Specialized equipment (e.g., seismometers, cameras, GPS stations, ground motion sensors, high-resolution photogrammetric monitor, etc.) are installed to actively and/or cyclically monitor fossil sites, provide quantitative data on Earth processes, or to provide early warning of imminent impacts. A predictive model and maps through GIS are then developed to identify paleontological areas which would be impacted by the identified hazards, at various degrees of severity (e.g., different earthquake magnitudes, extent of mass wasting events, extent of glacial advance or retreat, etc.).
Because this vital sign requires higher costs and professional support to monitor, this level of monitoring would only be justified for in situ paleontological resources of substantial significance. The level of technical support would be determined by the type of geohazard.
Discussion
This vital sign is limited to very specific circumstances where a geologic hazard is identified as a potential threat to in situ paleontological resources. Depending on the type of geohazard, the alternative may be the salvage of any surficial fossils which might otherwise be destroyed if the geohazard event occurs.
Land and resource managers must also consider the public safety aspects of local geologic hazards. Any planning should evaluate whether site monitoring would place individuals in an unsafe environment.
Vital Sign 4: Hydrology and Bathymetry
Description
Fossiliferous strata and paleontological resources may occur in areas submerged by or adjacent to either marine or fresh-water resources. These hydrologic resources include oceans, lakes, ponds, reservoirs, hot springs, rivers, and streams. Water levels of these features, and any associated hydrologic processes, may change over time due to fluctuations in precipitation, temperature, winds, tidal patterns, and catastrophic events. Tsunamis, hurricanes, rapid melting of ice due to volcanism, landslides, and other factors may result in tidal surges, abrupt changes in wave patterns and intensity, flooding, and other significant hydrologic events.
The stability of in situ fossils located in areas submerged by or adjacent to hydrologic resources is influenced by changes in water levels and forces associated with the movement of water. Therefore, fossils which occur along marine coastlines, lakeshores, riverbanks, and similar areas are threatened by hydrologic processes and events. Human activities may also influence water levels and forces, especially the construction of dams. Changes in water levels may result in the emergence or submergence of fossils. Hydrologic processes contribute to changes in the rates of sedimentation and erosion, therefore leading to the exposure of fossils and influencing their stability at the surface. Monitoring changes in water level, bathymetry, or other hydrologic variables at fossil sites will enable land and resource managers to better determine how frequently to schedule site condition assessments or cyclic prospecting.
Methods
Level 1: Repeat Photography. The stability of in situ paleontological resources in areas submerged by or adjacent to marine and fresh-water resources can be assessed using repeat photography. Each fossil site identified through field inventories should be spatially defined, mapped, and photographed. Photo points must be established for both large-scale and small-scale repeat photography so that consistent points of reference and perspectives are available.
Whenever possible, records of high and low water levels should be maintained for water resources which are associated with in situ fossil sites. The schedule or frequency of the repeat photography should be determined based upon site specific variables including short and long term changes in water levels and hydrologic processes. Any observable impacts to the fossils should be documented and photographed during periods of above- or below-normal water levels.
All photographs should be maintained in a secure archival storage facility. Each photograph should be labeled with the site name or number, photo point identifier, date of photograph, and name of photographer. Additional site data, such as stratigraphic, taxonomic, or historical information, should be maintained in an associated file or database.
Repeat photography is a low-cost and low-technology approach to monitoring paleontological sites over time. This photography could be undertaken by a trained volunteer. Photos enable the documentation of observable changes in stability at each site, and the fossils exposed at the surface. This level of information may also enhance resource management and scientific decisions, such as the frequency of cyclic prospecting or stabilization of the site.
Level 2: Digital Mapping. Fossiliferous strata and stability of in situ paleontological resources are monitored through the development of a digital map (GIS) integrating spatial fossil and shoreline data. High and low water events are recorded and areas where fossils are submerged or emerged are documented. Emergent areas should be assessed and documented during low water periods for exposure of previously submerged paleontological resources. Areas should also be assessed after high water periods for accelerated erosion, landslides, or sediment burial. This data can be used to determine which fossil sites are more susceptible to the impacts of changes in water levels and hydrologic processes.
This level of monitoring requires moderate levels of funding and support, and a trained technician who specializes in geographic information systems.
Level 3: Technology-Enhanced Monitoring. Fossiliferous strata and stability of in situ paleontological resources are monitored with technology-enhanced monitoring equipment. Hydrologic monitors (tidal gauges or flow meters) are established to record changes in water depth and velocity. A predictive model and maps through GIS are then developed to identify paleontological areas which would become submerged or emerged at different water levels. Data from the hydrologic monitoring equipment and the predictive model are used to establish management prescriptions for paleontological sites adjacent to bodies of water. Monitoring data can be used to determine which sites are more susceptible to the impacts of changes in water levels and hydrologic processes.
This method is a relatively high-cost approach to monitoring paleontological localities. The periodic measurements and evaluation of data require a professional geologist and someone trained in the use of the specific technology employed in the monitoring. The data from the technology-enhanced monitoring equipment will enable documentation of measurable changes in stability at each site, and fossils exposed at the surface or submerged beneath water. This level of information may also enhance resource management and scientific decisions, such as the frequency of cyclic prospecting or stabilization of the site.
Discussion
This vital sign is specifically designed for paleontological resources directly associated with water resources. The types of monitoring equipment employed will depend upon whether the objective is to measure changes in water levels or changes in the energy or velocity of moving water. Sometimes watercraft may be needed to conduct site monitoring. Land and resource managers must also consider the public safety aspects of any work in or near water. All planning should evaluate whether site monitoring would place individuals in an unsafe environment. Appropriate water safety training and equipment should be utilized.
Water levels in dam-controlled reservoirs can vary daily, sometimes on a set schedule. The Army Corps of Engineers, U.S. Bureau of Reclamation, and other offices record daily water level for major reservoirs in the United States. This information is generally available online.
Vital Sign 5: Human Impacts
Description
The stability of in situ paleontological resources may be directly associated with human related variables. Public access to fossil sites may increase the potential for either intentional or unintentional impacts. Further, the types of activities and behaviors engaged in by humans may pose significant threats to fossils. Human activities can have both large-scale and small-scale effects on continental erosion and sedimentation (Wilkinson and McElroy, 2007).
The types of human activities permitted on public lands are a function of the laws, regulations, and mission of the respective land managing agency. Certain federal or state land managing agencies may support human activities such as agriculture, forestry, oil and gas development, surface/subsurface mining, and the use of off-road all-terrain vehicles (ATVs). These types of human activities can have direct and significant impacts on the stability of in situ fossils.
In addition to human activities that may be legally permitted, unauthorized activities may also threaten and contribute to the loss of fossils. Theft and vandalism of paleontological resources has been documented at greater frequency on public lands. The unauthorized collecting of fossils ranges from casual souvenir gathering to intensive, systematic collection. An escalating commercial market for fossils may be responsible for the increasing number of incidents of fossil theft documented on public lands.
Given the potential for human impact, monitoring public use would enhance land and resource manager’s ability to make informed decisions related to management of fossil sites. Monitoring public use may help determine the scheduling of site condition assessments or cyclic prospecting.
Methods
Level 1: Repeat Photography. The stability of in situ fossils present in developed visitor use areas (e.g., trails, buildings, roads, parking areas, etc.) or areas where future development or construction is planned is monitored using repeat photography. The types of visitor behaviors and activities are documented. Evidence of adverse impacts to paleontological resources through each type of visitor behavior or activity (increased erosion, damage to fossils, theft, vandalism), should also be observed and documented. Each site should be spatially defined, mapped, and photographed. Photo points must be established for both largescale and small-scale repeat photography so that consistent points of reference and perspectives are available.
Whenever possible, changes in public access and activities should be measured and monitored relative to potential impacts to associated in situ fossils. The schedule or frequency of the repeat photography should be determined based upon site-specific variables, including short- and long-term changes in public use patterns. Any observable impacts on paleontological resources should be documented and photographed in areas where human activities increase or change.
All photographs should be maintained in a secure archival storage facility. Each photograph should be labeled with the site name or number, photo point identifier, date of photograph, and name of photographer. Additional site data, such as stratigraphic, taxonomic, or historical information, should be maintained in an associated file or database.
Repeat photography is a low-cost and low-technology approach to monitoring paleontological sites over time. This photography could be undertaken by a trained volunteer. Photos enable the documentation of observable changes in stability at each site and the fossils exposed at the surface. This level of information may also enhance resource management and scientifi c decisions, such as the frequency of cyclic prospecting or stabilization of the site.
Level 2: Digital Mapping. Fossiliferous strata and the stability of in situ fossils should be monitored through the development of digital maps (GIS) which integrate public use data. Areas with the highest public use should be identified, and monitoring prescriptions developed accordingly. Further, digital maps (GIS) should be developed which integrate spatial fossil site data and visitor activity areas. Evidence of adverse impacts from each type
of visitor behavior or activity should be observed, documented, and recorded as metadata. Areas where visitor behaviors and activities directly impact in situ paleontological resources should be determined and monitored appropriately.
Impacts of existing or future land use on paleontological resources should be assessed, and recommendations to mitigate possible threats provided. Monitoring data can be used to determine which sites are more susceptible to changes in public use patterns or human activity. This level of monitoring requires moderate levels of funding and a trained technician who specializes in geographic information systems.
Level 3: Technology-Enhanced Monitoring of Public Use. At this level, photographic or video surveillance equipment is installed, with increased frequency at sites experiencing high levels of visitor use. Impacts of future public use patterns on paleontological resources can be assessed and alternatives presented which enhance the protection of susceptible fossils.
A predictive model and maps can be developed through GIS to identify paleontological areas which would be most heavily impacted by increases or changes in public use. Data from the public use monitoring equipment and the predictive model can be used to establish management prescriptions for paleontological sites most impacted by human activities. Monitoring data can be used to determine which fossil sites are most susceptible to the
impacts of changes in public use. Technology-enhanced monitoring is a relatively high-cost approach to monitoring fossil sites. The measurements and evaluation of data require a professional geologist and someone trained in the use of the specific technology employed in the monitoring. The data from the technology-enhanced monitoring equipment will, over time, enable documentation of measurable changes in the stability at each site. This information may also enhance resource management and scientific decision making about a fossil site, such as the frequency of cyclic prospecting or stabilization.
Discussion
The management of public use and activities undertaken by humans is often accomplished at the regulatory level for respective land managing agencies. Some agencies operate under more resource preservation mandates, while other agencies support a broader range of multiple-use activities. Balancing resource stewardship and public use is often the subject of healthy philosophical and legal debate.
Federal and state land managing agencies have evolved over recent decades to consider and recognize the importance of the stewardship of nonrenewable paleontological resources. Federal agencies engaged in ground-disturbing activities which may impact in situ fossils are required to undergo an environmental review process under the guidance of the National Environmental Policy Act (NEPA, 1969) and Section 106 of the National Historic Preservation Act (NHPA, 1966).
Land managing agencies involved in some type of developmental planning must initiate environmental compliance review of the proposed project. A few examples of projects requiring environmental compliance review include: construction of new roads or trails; oil and gas development (Santucci, 2000; Santucci, et al., 2001a); surface/subsurface mining; timber harvesting; and agricultural development. During the compliance review, comments are solicited from professional scientists, engineers, historians, and other stakeholders, as well as the public at large, so that all interests may be considered.
Land managing agencies also evaluate the effects of public recreational use on resources. The use of ATVs, snowmobiles, and other recreational vehicles may significantly impact cultural and natural resources, including in situ fossils. Land managing agencies consider legislation, regulations, and public interests in the development of policies that relate to recreational use on public lands.
In areas designated as “wilderness,” public use limits are generally established by law and careful planning by land and resource managers. Wilderness areas prohibit the development of roads and structures, restrict the use of motorized vehicles, and often manage the numbers of visitors through a permit system. Therefore, in situ paleontological resources within wilderness areas typically do not face the same level of human-related
threats as those on other public lands.
Depending on the levels of public access and the types of human activities in paleontological resource areas, the impacts to in situ fossils may range from negligible to significant. The types of monitoring equipment employed for measuring any human impact
will be dependent on site-specific variables and conditions.
It is also important to consider the loss of fossils through theft and vandalism. Santucci (2002, 2006) provides an assessment of illegal activities which have resulted in either the loss or destruction of in situ fossils on public lands. Surface exposures of petrified wood, dinosaur trackways, and other paleontological resources are often managed in situ to support scientific and public educational goals. Informal monitoring has consistently shown evidence of unauthorized collection of these fossils.
At Petrified Forest National Park, Arizona, the illegal collection of souvenir petrified wood by the public has been recognized for over one hundred years. Photographs of the petrified wood deposits taken during the 1930s can be compared to similar photographs today (Fig. 6). This repeat photography shows areas of the park which have been essentially depleted of petrified wood.
In some areas, such as Petrified Forest National Park, where intensive illegal collecting of in situ fossils has been documented over long periods of time, social science studies have been undertaken to assess this behavior. In these social science studies, researchers evaluate visitor attitudes and perceptions and their relationship to behaviors which may affect the stability of in situ fossils (Williams et al., 1993; Hockett and Roggenbuck, 2002, 2003). Land and resource managers may utilize data from these social science studies to establish targeted monitoring prescriptions for areas where visitor behavior and activity adversely impact paleontological resources.
Photo on the left is from 1932, photo on the right is from 2002. National Park Service photos courtesy of T. Scott Williams.
Paleontological resource monitoring is distinctly different from other types of geological resource monitoring. As nonrenewable resources which preserve the remains of life in a geologic context, there are scientific, intellectual, and legal reasons for monitoring fossils. As discussed above, there are both natural and human related variables which threaten the stability of in situ fossils.
Once the destructive agents act upon in situ fossils, observable and sometimes measurable outcomes occur. The changes in fossilized remains may be gradual or abrupt, resulting in breakage, fragmentation, crumbling, shatter, scatter, dislodge, transport, disintegration, and even complete loss. These changes diminish the scientific and educational values of paleontological resources. Further, the concept of “limits of acceptable change” is not applicable to the management of nonrenewable resources such as fossils.
The five vital signs proposed for monitoring in situ fossils are designed to focus on those variables which most directly influence the stability (or health) of fossils within a geologic context (Table 1). Monitoring paleontological resources through the use of one or more of the vital signs will enable land and resource managers to identify those stressors, threats, and variables which have the greatest impacts upon the stability of in situ fossils. This data will enhance management’s ability to target the most appropriate mitigation strategies and to establish the frequency of periodic condition assessments based upon site-specific variables.
Photodocumentation of the stumps and debris trails in 2002 allowed creation of a baseline condition for the assessed stumps. National Park Service photos.
During the process of determining which vital signs might be appropriate for monitoring, land and resource managers should review any and all existing paleontological resource inventory data available. The inventory data should provide baseline information which may contribute to successfully determining the existing variables, conditions, and locations where vital signs monitoring may be most valuable. The selection of vital signs should consider such variables as erosion rates, climatic conditions, proximity to active geohazards, changes in water levels or hydrologic patters, or types of human activities which could adversely impact in situ fossils. This evaluation process can be referred to as the development of a “management prescription for vital signs monitoring of in situ paleontological resources.” When developing monitoring prescriptions and strategies, they should be consistent with natural resource management policies, such as those outlined for the National Park Service as resource management guidelines, director orders, and management policies (National Park Service, 2001, 2004, 2006).
During the selection and prioritization of sites to include in vital signs monitoring, sites which are the most sensitive or experience the greatest threats would likely be assigned a higher priority. The number of sites to begin monitoring should initially be limited to one or two of highest priority. This will enable staff to learn and become efficient with the methods, and assess funding and equipment needs. Land and resource managers can develop their monitoring skills during the first few years, and then refine them.
Finally, it is worth mentioning that vital signs monitoring is designed to provide land and resource managers with data relative to variables which threaten in situ fossils. Therefore, data may on occasion indicate resource threats at levels higher or lower than anticipated. In these cases, some management intervention or action may be warranted. These determinations are beyond the scope and intent of this chapter.
CASE HISTORIES
The science of paleontology has focused very little attention on the monitoring of in situ fossil sites, as evidenced by the general lack of scientific literature focusing on this issue. Similarly, monitoring strategies and management prescriptions for the long-term assessment of fossil sites are rarely employed by land and resources managers. Despite the paucity of rigorous efforts in long-term monitoring of in situ paleontological resources, a few case studies present lessons learned which contribute to this discussion: Fremd, (1992); Kunzman, et al. (1993); Fremd (1995); Nyborg and Santucci (1999); Koch, et al. (2002); DeBlieux, et al. (2003); Santucci and Koch (2003); and Vissagi, et al. (2005).
Yellowstone National Park: Rates of Erosion—Photo Monitoring
The petrified forests of Yellowstone National Park are frequently located on very steep slopes in poorly consolidated volcaniclastic deposits (Fig. 7A). These conditions create a highly erosive environment, evidenced by the down-slope “trail” of fossil wood fragments associated with many of the in situ stumps (Fig. 7B). The park has historical references and photographs regarding the condition of a few individual stumps. To assess rates of erosion and impacts, the stumps in historical records and photographs were located, captured by GPS, and photographed during field work in 2002. Additional stumps were also identified and inventoried. This photo record created a baseline condition for comparison with subsequent site visits and photographs, allowing resource managers to see changes over time and undertake appropriate management prescriptions.
Fossil Butte National Monument: Rates of Erosion—Monitoring Stakes
The highly erosive Wasatch Formation exposures within Fossil Butte National Monument contain a wide variety of fragmented terrestrial fossils. Because the rates of erosion and subsequent exposure and loss of fossil material are not well understood, the park initiated a multifaceted approach to study erosion rates. The park established a monitoring program incorporating many different vital signs, including the freeze-thaw index and the installation, photography, and GPS positions of erosion monitoring stakes (Fig. 5). Fossil Butte also adopted a procedure in which all fossil material exposed at the surface was located by GPS and collected. These sites are revisited as time and personnel permit. All new material continues to be located by GPS and collected. By comparing initial fossil densities to subsequent collections, a proper collecting cycle can be adopted. The abundance of new material in some areas suggests that a 2–3-year cycle is required, while the paucity of material at most sites suggests that their reexamination is only needed once every 5 to 10 years.
Hagerman Fossil Beds National Monument: Geohazards—Landslides
Landslides in the fossil-rich, but very unstable, Glenns Ferry Formation are a significant threat to paleontological resources within Hagerman Fossil Beds National Monument. More than a dozen landslides have occurred within the park over the past 25 years, some of which have led to the destruction of paleontological sites and in situ fossil material. Leakage from extensive irrigation in the area has fed perched artificial aquifers, believed by hydrologists to be triggers of slope failure. Park staff and an international team of hydrologists, geologists, and landslide specialists continue to intensively study and monitor the ongoing landslide and groundwater activity. Because of the slope instability, the need for increased documentation and monitoring of known paleontological sites is warranted. The park’s current paleontological monitoring plan addresses these issues along with the safety of personnel working in such unstable sediments.
Headings and distance (dashed arrows) from reference stakes to fossils provide location control and are repeated periodically. Distance from each stake to cutbank is also measured and monitored to assess local erosion rates.
An assessment of in situ paleontological resources at Curecanti National Recreation Area began in the summer of 2005. Wave action, temperature fluctuations (freeze-thaw index) and human access were identified as significant threats to paleontological resources at the reservoir. In response, the park began to collect as much identifiable surface material as possible and to implement a cyclic monitoring program to determine the rates at which new fossil material is being exposed. As of October 2005, material from at least one threatened site has been collected and identified. In 2006, in response to a technical assistance request to the National Park Service Geologic Resources Division, the park initiated photo monitoring at a number of sites to better document the exposure of new fossil remains, or the impacts on previously exposed material. At one beach site, a series of nine reference stakes were installed at regular intervals above the reservoir’s high water cutbank. These GPS-referenced stakes were numbered and photo-documented (Fig. 8). Park staff then measured the distance and bearing from the nearest stake to five significant fossils or landmarks at the site (Fig. 9). The location of the reference stakes also provides a datum for documentation of new specimens. When the stakes were installed, the distance between the stake and the cutbank was also measured. Changes in this distance over time may serve as an indicator of local erosion rates. This cost-effective approach is easily duplicated in similar fossil sites and is highly adaptable to local conditions.
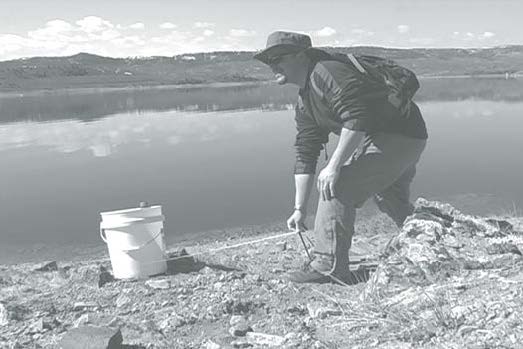
These reference stakes, located above the eroded cutbank, provide a datum for photodocumentation and location of paleontological resources periodically inundated by the Blue Mesa Reservoir. National Park Service photo.
The scientifically significant and easily accessible petrified wood deposits at Petrified Forest National Park are considered among the most endangered by resource theft. Unauthorized souvenir collecting of petrified wood is undertaken by many park visitors. The park utilizes a number of different management strategies to combat this loss of petrified wood, including photographic monitoring. A pilot project initiated in the early 1990s established monitoring plots in areas of varying public accessibility. The plots were photographed and the amount of fossil wood was determined within each plot. Subsequent monitoring of the plots documented significant levels of petrified wood loss, which was calculated to be consistently greater in areas more accessible to the public. Historical photographs showing some of the more famous petrified wood sites in the park extend back to the 1930s, providing the opportunity to measure and monitor long-term loss of petrified wood (Fig. 6).
The discussions associated with the loss of petrified wood at Petrified Forest National Park often include a reference to the story of Fossil Cycad National Monument. Despite the fact that this important fossil site was established as a national monument in 1922, the lack of resource monitoring at the site and years of unauthorized collecting led to a nearly complete loss of the fossil cycads (Santucci and Hughes, 1998). The mismanagement and loss of these resources at Fossil Cycad National Monument led Congress to officially abolish the monument in 1957.
The management of in situ fossils presents many interesting and complex challenges to land and resource managers. The variables associated with the stewardship of nonrenewable paleontological resources, as briefly outlined above, do not generally allow for simple textbook solutions. The vital signs presented here are intended as recommendations to be adopted in part or whole based upon the paleontological site variables, conditions, and the resource management goals. At minimum, this information may generate new thoughts and discussions that could ultimately enhance our ability to manage and protect in situ fossils.
It is essential to recognize that resource management practices should not be designed to impede natural processes. Monitoring strategies should be based upon natural processes and their potential impacts on the stability of in situ paleontological resources. Understanding the natural processes acting upon a fossil site or area will enable the development of more precise, locally focused monitoring prescriptions.
Therefore, monitoring schedules should incorporate the rates at which natural processes and/or human-related activities potentially impact each paleontological site. In turn, site assessments (cyclic monitoring) will be conducted at regular intervals, defined within management prescriptions, to minimize the loss of scientifically significant specimens or information due to natural processes or human factors.
Most of the history of life on Earth has yet to be discovered. Our ability to assess and monitor these nonrenewable and scientifically valuable resources will result in a better understanding of the story of life on our planet.
REFERENCES CITED
Colbert, E.H., 1966, Rates of erosion in the Chinle Formation—ten years later: Museum of Northern Arizona Plateau, v. 38, no. 3, p. 68–74.
DeBlieux, D.D., Smith, J.A., McGuire, J.L., Santucci, V.L., Kirkland, J.I., and Butler, M., 2003, A paleontological inventory of Zion National Park, Utah, and the use of GIS technology to create paleontological sensitivity maps for use in resource management: Journal of Vertebrate Paleontology, v. 23, Supplement to No. 3, Abstracts of Papers, p. 45A.
Fremd, T., 1992, Paleontological resource management, NPS-77, and its practical applications: Proceedings of the Third Conference on Fossil Resources, Programs with Abstracts, National park Service Technical Report NPS/NRFOBU/NRR-94-14, p. 18.
Fremd, T., 1995, Cyclic prospecting to preserve vertebrate paleontological resources: San Bernardino County Museum Association Quarterly, v. 4, no. 2, p. 12–25.
Hockett, K.S., and Roggenbuck, J.W., 2002, Characteristics of visitors to Fossil Butte, New Mexico, and the influence of the visitor center on fossil knowledge and ethics. A final report submitted to Fossil Butte National Monument, National Park Service, Kemmerer, Wyoming: Department of Forestry, Virginia Polytechnic Institute and State University, Blacksburg, Virginia, USA.
Hockett, K.S., and Roggenbuck, J.W., 2003, Infl uence of interpretation along a nature trail on visitor attitudes and behavior toward fossils. A final report submitted to Fossil Butte National Monument, National Park Service,Kemmerer, Wyoming: Department of Forestry, Virginia Polytechnic Institute and State University, Blacksburg, Virginia.
Kunzman, M.R., Guertin, D.P., and Santucci, V.L., 1993, Cost effective paleontological field data acquisition techniques, strategies, and database management applications at Petrified Forest National Park (abstract): 53rd Society of Vertebrate Paleontology Meeting, Albuquerque, New Mexico, October 1993.
Koch, A.L., Santucci, V.L., and McDonald, H.G., 2002, Developing paleontological resource monitoring strategies for the National Park Service: Geological Society of America Abstracts with Programs, v. 34, no. 6, p. 422.
National Park Service, 2001, Natural Resource Management Reference Manual 77: Department of the Interior, National Park Service, Washington, D.C., http://www.nature.nps.gov/rm77/ (accessed 23 January 2007).
National Park Service, 2004, Director’s Order 2-1 (Resource Stewardship Planning): Department of the Interior, National Park Service, Washington, D.C., http://www.nps.gov/policy/DOrders/draftDO2-1.html. (accessed 23 January 2007).
National Park Service, 2006, Management Policies: U.S. Department of the Interior, National Park Service, Washington, D.C., http://www.nps.gov/ policy/mp/policies.html (accessed 23 January 2007).
Nyborg, T.G., and Santucci, V.L., 1999, In situ management and track site conservation of Late Miocene vertebrate tracks in Death Valley National Park, California (abstract): George Wright Society 1999 Annual Meeting, Asheville, North Carolina.
Raup, D.M., and Stanley, S.M., 1978, Principles of Paleontology: San Francisco, W.H. Freeman, 481 p.
Santucci, V.L., 2000, Standard Operating Procedures for locating and protecting paleontological resources. Lake Meredith National Recreation Area and Alibates Flint Quarries National Monument Oil and Gas Management Plant/Environmental Impact Statement: National Park Service, Denver, Appendix F, p. F1–2.
Santucci, V.L., 2002, Theft and vandalism of vertebrate tracksites: Challenges of in situ management and protection: Journal of Vertebrate Paleontology, v. 22, Supplement to No. 3, p. 103A.
Santucci, V.L., 2006, Theft and vandalism of in situ fossil vertebrate tracksites (abstract), in Lucas, S.G., et al., eds., American Antiquities: 100 Years of Managing Fossils on Federal Lands: Proceedings of the 7th Federal Fossil Conference, New Mexico Museum of Natural History and Science Bulletin No. 34, p. 34.
Santucci, V.L., and Hughes, M., 1998, Fossil Cycad National Monument: a case of paleontological resource mismanagement. National Park Service Technical Report NPS/NRGRD/GRDTR-98-01, p. 84–90.
Santucci, V.L., and Koch, A.L., 2003, Paleontological resource monitoring strategies for the National Park Service: Park Science, v. 22, no. 1, p. 22–25.
Santucci, V.L., Hunt, A.P., and Norby, L., 2001a, Oil and Gas Management Planning and the protection of paleontological resources: Park Science, v. 21, p. 36–38.
Santucci, V.L., Kenworthy, J., and Kerbo, R., 2001b, An inventory of paleontological resources associated with National Park Service caves: National Park Service Technical Report NPS/NRGRD/GRDTR-01/02, 50 p.
Shipman, P., 1981, Spatial distribution of fossils in sediments, in Shipman, P., Life History of a Fossil, an introduction to taphonomy and paleoecology: Cambridge, Harvard University Press, p. 65–98.
Vissagi, C.C., Santucci, V.L., Kenworthy, J.P., and Koch, A., 2005, Compiling servicewide paleontological resource data for effective vital signs monitoring in National Parks: Geological Society of America Abstracts with Programs, v. 37, no. 7, p. 331.
Wilkinson, B.H., and McElroy, B.J., 2007, The impacts of humans on continental erosion and sedimentation: Geological Society of America Bulletin, v. 119, p. 140–156, doi: 10.1130/B25899.1.
Williams, D.R., Roggenbuck, J.W., Santucci, V.S., Stratton, D.W., and Borrie, W.T., 1993, Social science methods for studying illegal fossil collecting: a case study from Petrified Forest National Park (abstract): 53rd Society of Vertebrate Paleontology Meeting, Albuquerque, New Mexico, October 1993.