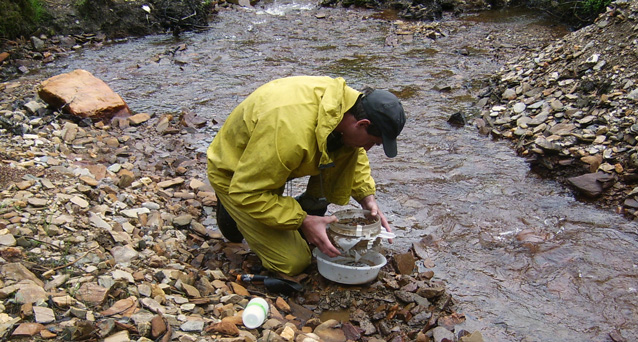
Photo by Vanessa Ritchie
Gold may have drawn miners to “them, thar” Kantishna Hills in the early 1900s, but the notoriety of the Kantishna Hills Mining District also extended to antimony lode deposits. Over half of Alaska’s antimony production from the 1940s and 1950s came from this region, and the Stampede Mine (now within Denali National Park and Preserve) is Alaska’s historically largest antimony producer.
What is antimony?
Antimony is an element with the symbol Sb (stibium is Latin for “mark” and antimony has been used in products such as paint that leave a mark). Antimony can take on a variety of oxidation states (chemical forms), most commonly Sb(III) and Sb(V). Each form has different reactive properties that directly affect how Sb combines with other elements, as well as its likelihood to be transported downstream via water or stream sediment.
At Stampede Mine, the orebody containing antimony is composed primarily of massive stibnite (antimony combined with sulfur (Sb2S3)) and other sulfur compounds containing iron or arsenic (pyrite (FeS2) and arsenopyrite (FeAsS)). The orebody is “hosted” in Precambrian to Paleozoic metamorphic rocks.
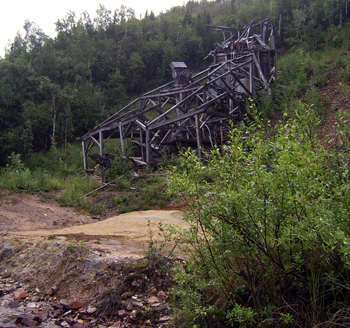
Photo by Tom Trainor
Weathering effects on exposed ore
The chemistry of antimony and its weathering products is complex. When the exposure of antimony orebodies to oxidizing surface environments (air) is accelerated through mining, the weathering of the sulfide-rich ores results in the release of oxidized metals (e.g. iron, copper, lead), metalloids (e.g. arsenic, antimony), and sulfuric acid to the local surface and near-surface waters.
Antimony and arsenic are highly toxic, and stibnite and arsenopyrite compounds containing these elements are very common in Kantishna. The potential risk for human and wildlife exposure to antimony depends on how far antimony moves downstream from the orebodies. The movement of antimony depends on whether oxidation produces soluble or insoluble products, which is directly related to the form of antimony involved. Sb(III) is believed to be more toxic but less mobile than the Sb(V) form.
Thus, water quality within the Kantishna Hills region of Denali is of concern due to the presence of antimony-rich mineral deposits and the legacy mining operations that exposed them to oxidation.
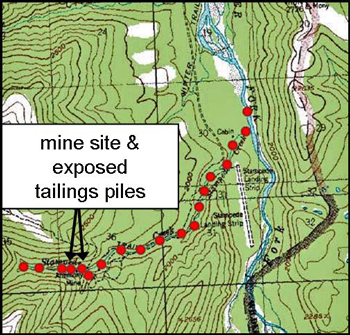
NPS photo
Research study of Stampede Mine area
To learn how antimony moves from its lode sources, and to what extent it affects stream water and sediments, researcher Tom Trainor, of the University of Alaska Fairbanks, and graduate student Vanessa Ritchie, have been investigating antimony in the Stampede Mine area. Prior to this study, most research has focused on understanding the geochemical behavior of arsenic, rather than antimony, in the surface environment.
Field sampling and laboratory methods
In 2006, to document the transport of antimony downstream from the Stampede Mine, the researchers sampled intensively (see high density of points on map at right) the stream water and stream sediments in the Stampede Creek drainage from upstream of the Stampede Mine to downstream of the confluence with the Clearwater Fork.
To determine which of the important forms of antimony—Sb(III) vs. Sb(V)—was most common in Stampede Creek, Trainor and Ritchie used a technique involving liquid chromatography linked to a mass spectrometer. Each water sample was passed through a column to separate the Sb forms, and then each form was identified by its mass. To analyze which forms of antimony and arsenic were found in the stream sediment and tailings samples, they used an X-ray absorption spectroscopy (XAS) technique.
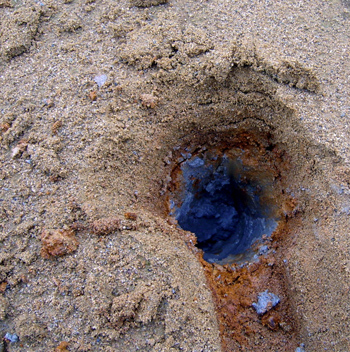
Photo by Tom Trainor
Research findings
In general, the concentrations of most priority trace metals in Stampede Creek drainage met EPA drinking water standards, or at least rapidly declined with distance from the source environment (the mine and tailings pile). However, elevated antimony (and arsenic) concentrations were observed near the tailings pile (up to 260 parts per billion) as well as several miles downstream from the mine source (see graph below). For comparison, safe drinking water standards for antimony, as determined by the EPA, are 6 parts per billion. This spatial distribution of antimony indicates that it remains highly mobile in the Stampede drainage.
The results of the chemical analysis indicate that the majority of antimony is in the more mobile Sb(V) form, not surprising given its considerable downstream transport. Even in the vicinity of the tailings, this form predominates, suggesting that oxidation of antimony is very rapid in the Stampede Creek area.
Concentrations of antimony and arsenic in the stream sediment are also elevated downstream of the mine tailings. The concentrations of sediment antimony are correlated with those of sediment arsenic, suggesting the two elements have similar mobility. The XAS analyses show that the Sb(V) form predominates in sediments and accumulates onto the surfaces of iron-oxide minerals. In contrast, arsenic was observed as both As(III) and As(V) in the stream sediments, suggesting it has a different geochemical behavior than antimony in Stampede Creek.
From the XAS analyses, they confirmed that antimony is typically moved downstream in association with iron-oxides, either suspended in the water or as part of the streambed load transport of the iron-oxides. The re-distribution of Sb back into the water from the iron-oxide surface is likely a slow process, and only makes a small contribution to the downstream water concentrations.
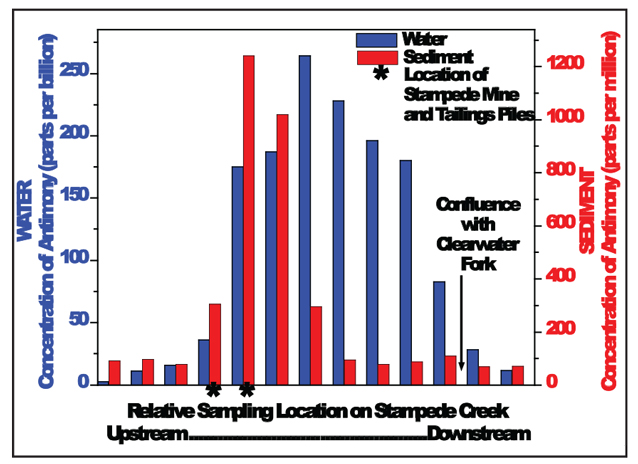
Development of geoenvironmental models
By compiling data about the characteristics of antimony (and other trace elements) in specific drainages such as Stampede Creek, where the characteristics of the ore-bearing rocks and the ore-deposit minerals are known, Trainor and Ritchie are adding to the data sets being gathered by other researchers. These data sets can be synthesized to develop geoenvironmental models that predict the concentrations and “behavior” of potentially toxic trace elements in other legacy mining locations that have similar geological properties and ore-deposit mineralogy.
Learning about the key physical and chemical processes controlling the trace element forms is critical to predicting the downstream mobility of trace elements, such as antimony. Understanding the chemical form not only helps to predict the element’s mobility, but also determines the potential bioavailability and, along with concentration, the toxicity of the element.
When the biogeochemical models are completed, they will provide a tool for public land managers and environmental quality agencies to develop effective remediation strategies and treatments for legacy mining contamination.
Acknowledgements
Funding was provided by U. S. Geological Survey Grant 06HQGR0177 and NSF Grant CHE-0431425. V. Ritchie also received a Discover Denali Research Fellowship from the Denali Education Center through the Murie Science and Learning Center.
Last updated: July 28, 2016