Last updated: October 23, 2021
Article
Fire in the Range of Western Arctic Caribou Herd
By Kyle Joly, T. Scott Rupp, Randi R. Jandt, and F. Stuart Chapin, III
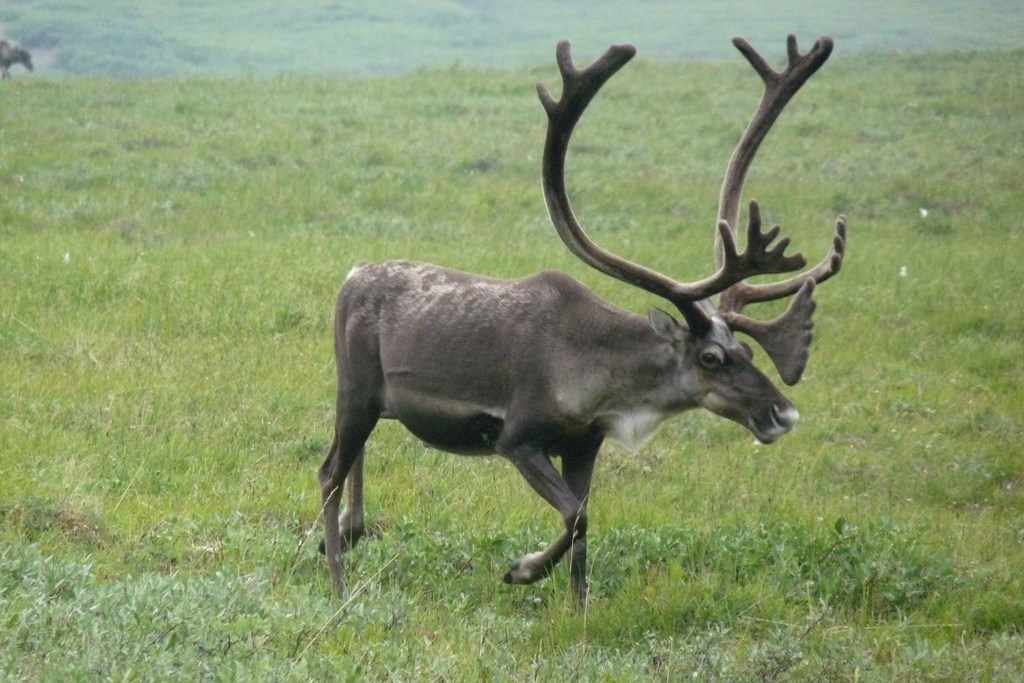
National Park Service photograph
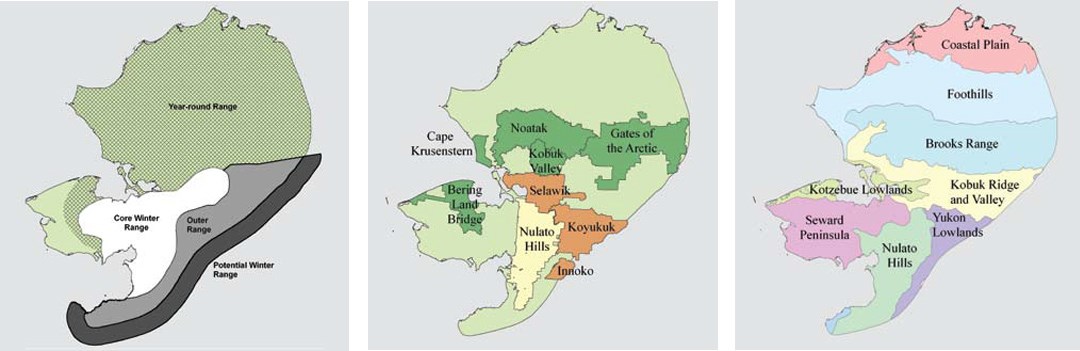
Abstract
Wildfire is the dominant ecological driver in boreal forest ecosystems. Although much less is known, it also affects tundra ecosystems. Fires effectively consume fruticose lichens, the primary winter forage for caribou, in both boreal and tundra ecosystems. We summarize 1950-2007 fire regime data for northwestern Alaska and sub-regions. We also identified meteorological factors that help explain the variability in fire extent across this landscape. We review information and inferences from recent studies on tundra fire regimes for managing caribou winter range. Climate warming may increase fire size and frequency in this region, which may substantially impact the vegetation, wildlife, and people of this region.Introduction
Although much attention has been focused on the role of wildfire in boreal forest ecosystems, its role in tundra ecosystems has largely been overlooked (but see Miller 1985, Racine et al. 1985). Wildfires effectively consume ground-dwelling lichens that are the primary winter forage for Western Arctic Herd (WAH) caribou. These lichens can take decades to recover to pre-fire levels in northwestern Alaska (Jandt et al. 2008). Wildfires potentially limit caribou winter range by destroying preferred forage (Rupp et al. 2006). The WAH has undergone a population expansion starting in 1976 until reaching a high of 490,000 caribou in 2003 (Dau 2005). The herd has expanded and shifted its primary winter range to the Nulato Hills and the Seward Peninsula from its historic winter range in the Buckland Valley and Selawik National Wildlife Refuge (Figure 2). Both density dependent (i.e., increasing herd size leading to reduced lichen biomass) and density-independent (i.e., increasing area burned by wildfires leading to less mature habitat) may factor into the changes in winter range distribution exhibited by the WAH.Temperatures, in Alaska, including northwestern Alaska, have been rising and the rate of climate warming is predicted to increase (Stafford et al. 2000, ACIA 2005). Summer precipitation has decreased at many locations throughout Alaska, and Barrow has seen declines in annual precipitation as well (Stafford et al. 2000). Warmer and drier summers are associated with greater area burned in Alaska (Duffy et al. 2005). In the tundra ecosystem, wildfires are also predicted to increase (Higuera et al. 2008). Regional temperature and precipitation are correlated with large scale climatic regimes, such as the Pacific Decadal Oscillation (PDO) (Hartmann and Wendler 2005). Our goals were to 1) elucidate the fire regime in northwestern Alaska and in tundra ecosystems, 2) test whether wildfires are increasing in extent and frequency in the range of the WAH and 3) identify meteorological variables that correlate with annual area burned.
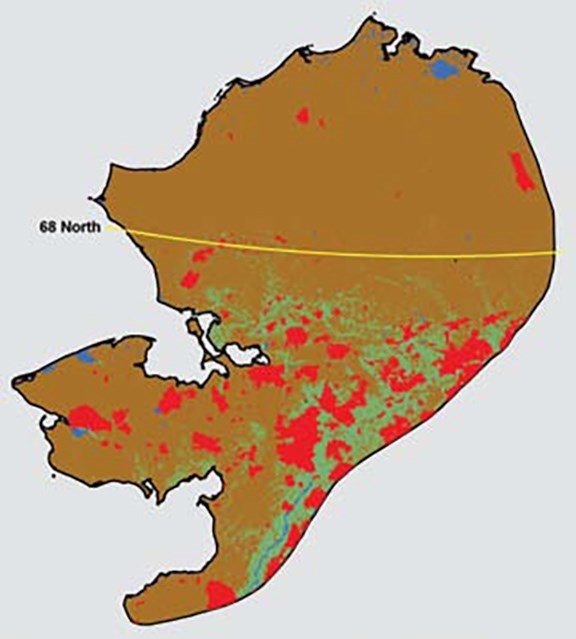
Methods
We summarized the data contained in the Alaska Fire Service’s geodatabase, which catalogs the extent, num-ber and location of large fires mapped from 1950-2007 (Figure 3; data). Kasischke et al. (2002) performed similar analyses for Interior Alaska, but we calculated them for northwestern Alaska and various subsets including the WAH core winter range, outer range, and potential future winter range - defined as a 30-mile (50-km) wide buffer around the outer range (Figure 2 Left). We also summarized burn area by conservation unit, ecoregion, tundra ecosystem, boreal forest ecosystem and also the area north of the 68th latitude (Figures 2 and 3). Tundra ecosystems were differentiated from forested areas using the 33-yard (30-m) National Land Cover Database – Alaska 2001 coverage.For all of these areas, we also calculated the percentage of burned area that burned two or more times during the 58-year study period. Similarly, we calculated the fire cycle (i.e., the number of years required to burn over an area) (Kasischke et al. 2002) for these areas. We calculated this by dividing 1 by the proportion of area burned and multi-increase over time (F1,42 = 9.95, P = 0.003). There were 15 years where the annual burn area was greater than 200,000 acres (81,000 hectares) and they were clustered into 4 groups – each group contained three to five years of high burn area, spanned four to nine years and were temporally separated by an average of 16.3 years (sd = 0.72). All but 1 (i.e., 14 of 15) of these high fire years were associated with average August temperatures exceeding 53°F (11.7°C). The exception occurred in 1969, which had the lowest June precipitation on record – well less than half the normal for the month. Although burned area was more than double in years with average August temperatures > 53°F (11.7°C), 226,000 versus 96,000 acres (91,000 versus 39,000 hectares), the difference was not statistically significant (P > 0.1).
The number of wildfires in northwestern Alaska and in the tundra ecosystem significantly increased from 1950 to 2007 (F1,57 = 11.50, P = 0.001, F1,57 = 11.40, P = 0.001, respectively). These trends disappeared when the analysis was limited to 1988-2007 (F1,18 = 0.73, P = 0.404, F1,18 = 0.72, P = 0.406, respectively). Dry weather in August was signifi-cantly associated with high August temperatures (F1,55 = 7.42, P = 0.009). A 6-factor (June-September precipitation and July-August temperature) model explained the most variation, approximately 31%, in annual burn area in northwestern Alaska. Explanatory power was increased when non-linear factors were added; a 5-factor model (June and August precipitation, exponential of June precipitation, exponential of August temperature and PDO) explained 55% of the variance in annual burned area. This model plus the exponential of June temperature explained 67% of the variance of average annual burned area within tundra ecosystems. The single factor of the exponential of August temperature explained 28% of the variance in burned area for northwestern Alaska and 47% for tundra fires in this region. For more on models, see Figure 6.
Region | % Area Burned | % Area Re-burned | Fire Cycle (years) |
---|---|---|---|
Caribou | |||
Core winter range | 19.6 | 8.7 | 296 |
Potential winter range | 34.9 | 6.8 | 166 |
Conservation Units | |||
Cape Krusenstern (NPS) | 0.1 | 0.0 | 53349 |
Gates of the Arctic (NPS) | 2.7 | 5.0 | 2173 |
Noatak (NPS) | 4.7 | 5.2 | 1237 |
Bering Land Bridge (NPS) | 4.9 | 0.0 | 1188 |
Kobuk Valley (NPS) | 6.9 | 3.5 | 844 |
Nulato Hills (BLM | 19.9 | 1.3 | 292 |
Selawik (FWS) | 28.0 | 15.7 | 207 |
Koyukuk (FWS) | 45.1 | 11.0 | 129 |
Innoko (FWS) | 57.6 | 12.7 | 101 |
Ecoregions | |||
Coastal Plain | 0.0 | 0.0 | n/a |
Brooks Range | 1.0 | 3.3 | 5917 |
Foothills | 1.7 | 0.0 | 3467 |
Kotzebue Lowlands | 6.8 | 2.9 | 859 |
Seward Peninsula | 13.9 | 15.1 | 418 |
Nulato Hills | 20.5 | 2.0 | 283 |
Kobuk Ridge and Valley | 30.0 | 8.6 | 193 |
Yukon Lowlands | 42.2 | 10.0 | 137 |
Discussion and Conclusions
We found that wildfire is a common occurrence in northwestern Alaska, in the range of the WAH and in tundra ecosystems. Burn acreage tended to decrease with latitude and longitude as, historically, fires have been rare events north of the Brooks Range and in maritime climates. Nearly 20% of the WAH core winter range has burned during the past 58 years. With current high population densities and declining lichen cover, the herd may seek out additional winter ranges (Joly et al. 2007b). We found that the WAH’s outer range has burned even more extensively than its core winter range. Potential future winter range, further to the east, was one of the most extensively burned areas in the region and also appeared to have one of the highest incidences of re-burning - likely because it is in the warmer and drier continental Interior climate zone. We believe this level of burning may prove to be an impediment for the herd to expand its winter range possibilities as extensive, mature lichen mats are unlikely to be found in these areas.The extent of burned area within Selawik National Wildlife Refuge (28%) came as a surprise, as well as the fact that this area had the highest percentage of re-burned area of any sub-region within northwestern Alaska. These facts may help explain why the herd has largely abandoned its historic winter range in the refuge, though density- dependant factors are likely to have also played an important role. The Seward Peninsula ecoregion had the second highest re-burn percentage, but is still utilized by the herd (Joly et al. 2007a). One possible reason for the continued use of this region as winter range is that it contains > 4 times more area that has not burned in the past 58 years than the Selawik. Re-burn estimates need to be cautiously interpreted, however, as fire perimeters in the AFS database do not account for unburned inclusions and in earlier years were often based on rough maps produced by firefighting crews.
As we expected, wildfire affected a greater percentage (~ 25%) of forested areas than tundra areas (< 10%) in north-western Alaska during the past 58 years. We found that burned tundra was 4.5 times more likely to re-burn than burned forest during our 58-year study period. This finding is intuitive because grasses and sedges that dominate tundra ecosystems recover very quickly (Jandt et al. 2008), and produce an important surface fuel (dead leaf litter) to carry new fires. Conversely, surface fuel loads (dominated by feather mosses) in the boreal forest can take decades to return (Kasischke and Stocks 2000, Camp et al. 2007). The fire cycle for tundra areas was more than 2.5 times longer than for forested areas. We did not find any examples of forested areas being re-burned more than once, while we found 11 cases in the tundra where a patch had re-burned more than once and one location on the central Seward Peninsula was mapped as burned in 1971, 1990, 1997 and 2002.
Using the large fire database, we were unable to detect a trend of increased annual burn area over time. This may be because climate warming is not yet strong enough to impact northwestern Alaska’s fire regime or is intertwined with other factors that may suppress wildfires. However, when we omitted large fire seasons, we found a strong increasing trend (Figure 7). This may be explained in at least two ways. First, changes in the accuracy of fire maps and in fire suppression capability and management over the period of record may affect apparent burn acreage trends. Alternatively, climate warming may indeed be increasing annual area burned, but some other factor may induce pulses of large fire seasons that mask this overall trend when included in the regression analysis. The number of wild and tundra fires in northwestern Alaska appear to have significantly increased during the past 58 years. This, however, may also be an artifact of the fact that fires less than 1,000 acres (405 hectares) were not regularly mapped prior to 1988. We did not find evidence that the number of wild and tundra fires have increased since 1988 – a time period when all of these factors should be equivalent in the database. Increases in both the area burned and number of fires in the boreal forest have been identified (Kasischke and Turetsky 2006).
Our addition of non-linear and Pacific Decadal Oscillation factors greatly improved our model’s ability to predict annual burned area. The model was even stronger at predicting the amount of burned tundra. The effects of climate change, potentially warmer and drier summer weather, may have non-linear effects on the fire regime of northwestern Alaska. For northwestern Alaska and tundra, the exponential of August temperature had the greatest explanatory power. For Interior boreal forests, June temperature was the single most important factor explaining variance in burned area (Duffy et al. 2005). Part of this difference may be explained by phenology differences between the ecosystems – in other words, summer simply comes later to northwestern Alaska than it does to the Interior, therefore temperatures later in the year are more important in determining annual burn area. Additionally, August is on average the coolest of the summer months but has the greatest variability. Warm temperatures in August were correlated with dry weather and thus it is not surprising that they are associated with increased annual burned area in northwestern Alaska (Miller 1985).
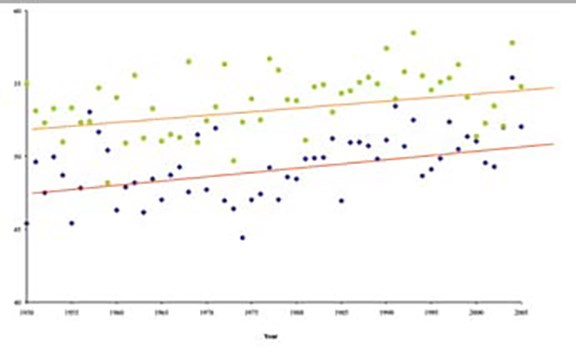
Model Variables | ▲ | Adj. |
---|---|---|
June and August precipitation, exp August temperature, exp June precipitation, PDO | 0.00 | 0.547 |
June precipitation, exp August temperature, exp June precipitation, PDO | 0.40 | 0.532 |
exp August temperature | 20.12 | 0.284 |
Model Variables | ▲ | Adj. |
---|---|---|
June and August precipitation, exp June and August temperature, exp June precipitation PDO | 0.00 | 0.667 |
June precipitation, exp June and August temperature, exp June precipitation, PDO | 0.16 | 0.656 |
June precipitation, exp August temperature, exp June precipitation, PDO | 0.47 | 0.645 |
exp August temperature | 19.10 | 0.467 |
Figure 6. Models for explaining the annual amount of area burned for (A) all of northwestern Alaska and (B) just for tundra (non-forested) ecosystems in northwestern Alaska, 1950-2005. The term “exp” means the exponential of that variable was used. The term “PDO” is the average of the January and February values of the strength of the Pacific Decadal Oscillation.
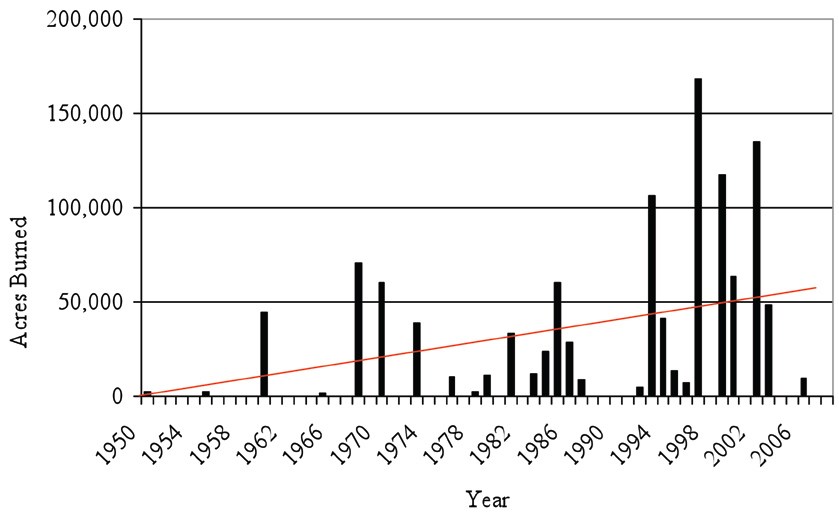
Management Implications
The management of wildfires is a contentious issue, not least of all because of its implications for caribou winter range. Our findings are based on the large fire database maintained by the Alaska Fire Service and thus should be viewed carefully. We believe our preliminary findings provide a starting point for understanding the importance of wildfire in northwestern Alaska and tundra ecosystems in general. While fires are less common in tundra ecosystems than in boreal forests, tundra ecosystems are capable of burning much more frequently. Understanding the fire regime of this region and its impacts on the WAH will be critical information utilized in the development of a fire management plan for the winter range of the herd.Acknowledgements
Paul Duffy, Mark Olson and Dave Verbyla provided constructive advice that improved our analyses and manuscript.
REFERENCES
Arctic Climate Impact Assessment (ACIA). 2005.
Cambridge University Press.
Camp, A.E., P.N. Omi, M. Huffman, and J.B. Cronan. 2007.
Refinement and development of fire management decision support models through field assessment of relationships among stand characteristics, fire behavior and burn severity. Joint Fire Science Program. Final Report 04-2-1-96.
Dau, J. 2005.
Western Arctic Herd. In Caribou management report of survey and inventory activities, 1 July 2002-30 June 2004, edited by C. Brown. Alaska Department of Fish and Game. Juneau, Alaska. 177-218.
Duffy, P.A., J.E. Walsh, J.M. Graham, D.H. Mann, and T.S. Rupp. 2005.
Impacts of large-scale atmospheric-ocean variability on Alaskan fire season severity. Ecological Applications 15:1317-1330.
Hartmann, B., and G. Wendler. 2005.
The significance of the 1976 Pacific climate shift in the climatology of Alaska. Journal of Climate 18: 4824-4839.
Higuera, P.E., L.B. Brubaker, P.M. Anderson, T.A. Brown, A.T. Kennedy, and F.S. Hu. 2008.
Frequent fires in ancient shrub tundra: implications of paleorecords for Arctic environmental change. PLoS ONE, 3(3): e0001744. doi:10.1371/journal. pone.0001744.
Jandt, R.R., K. Joly, C.R. Meyers, and C. Racine. 2008.
Slow recovery of lichen on burned caribou winter range in Alaska tundra: potential influences of climate warming and other disturbances. Arctic, Antarctic and Alpine Research 40: 89-95.
Joly, K., P. Bente, and J. Dau. 2007a.
Response of overwintering caribou to burned habitat in northwest Alaska. Arctic 60: 401-410.
Joly, K., R.R. Jandt, C.R. Meyers, and M.J. Cole. 2007b.
Changes in vegetative cover on Western Arctic Herd winter range from 1981-2005: potential effects of grazing and climate change. Rangifer Special Issue 17: 199-207.
Kasischke, E.S., and B.J. Stocks (editors). 2000.
Fire, climate change, and carbon cycling in the boreal forest. Springer-Verlag, New York.
Recent changes in the fire regime across the North American boreal region—Spatial and temporal patterns of burning across Canada and Alaska. Geophysical Research Letters 33, L09703, doi:10.1029/2006GL025677.
Kasischke, E.S., D. Williams, and D. Barry. 2002.
Analysis of the patterns of large fires in the boreal forest region of Alaska. International Journal of Wildland Fire 11: 131-144.
Miller, M. 1985.
Fire occurrence in the Northwest Planning Area, 1956-1982. Open File Report 15, Bureau of Land Management, Anchorage, Alaska.
Racine, C.H., W.A. Patterson III, and J.G. Dennis. 1985.
Tundra fire regimes in the Noatak River watershed, Alaska: 1956-1983. Arctic 38: 194-200.
Rupp, T.S., M. Olson, L.G. Adams, B.W. Dale, K. Joly, J. Henkelman, W.B. Collins, and A.M. Starfield. 2006.
Simulating the influences of various fire regimes on caribou winter habitat. Ecological Applications 16: 1730-1743.
Stafford, J.M., G. Wendler, and J. Curtis. 2000.
Temperature and precipitation of Alaska: 50 year trend analysis. Theoretical and Applied Climatology 67: 33-44.
Arctic Climate Impact Assessment (ACIA). 2005.
Cambridge University Press.
Camp, A.E., P.N. Omi, M. Huffman, and J.B. Cronan. 2007.
Refinement and development of fire management decision support models through field assessment of relationships among stand characteristics, fire behavior and burn severity. Joint Fire Science Program. Final Report 04-2-1-96.
Dau, J. 2005.
Western Arctic Herd. In Caribou management report of survey and inventory activities, 1 July 2002-30 June 2004, edited by C. Brown. Alaska Department of Fish and Game. Juneau, Alaska. 177-218.
Duffy, P.A., J.E. Walsh, J.M. Graham, D.H. Mann, and T.S. Rupp. 2005.
Impacts of large-scale atmospheric-ocean variability on Alaskan fire season severity. Ecological Applications 15:1317-1330.
Hartmann, B., and G. Wendler. 2005.
The significance of the 1976 Pacific climate shift in the climatology of Alaska. Journal of Climate 18: 4824-4839.
Higuera, P.E., L.B. Brubaker, P.M. Anderson, T.A. Brown, A.T. Kennedy, and F.S. Hu. 2008.
Frequent fires in ancient shrub tundra: implications of paleorecords for Arctic environmental change. PLoS ONE, 3(3): e0001744. doi:10.1371/journal. pone.0001744.
Jandt, R.R., K. Joly, C.R. Meyers, and C. Racine. 2008.
Slow recovery of lichen on burned caribou winter range in Alaska tundra: potential influences of climate warming and other disturbances. Arctic, Antarctic and Alpine Research 40: 89-95.
Joly, K., P. Bente, and J. Dau. 2007a.
Response of overwintering caribou to burned habitat in northwest Alaska. Arctic 60: 401-410.
Joly, K., R.R. Jandt, C.R. Meyers, and M.J. Cole. 2007b.
Changes in vegetative cover on Western Arctic Herd winter range from 1981-2005: potential effects of grazing and climate change. Rangifer Special Issue 17: 199-207.
Kasischke, E.S., and B.J. Stocks (editors). 2000.
Fire, climate change, and carbon cycling in the boreal forest. Springer-Verlag, New York.
Recent changes in the fire regime across the North American boreal region—Spatial and temporal patterns of burning across Canada and Alaska. Geophysical Research Letters 33, L09703, doi:10.1029/2006GL025677.
Kasischke, E.S., D. Williams, and D. Barry. 2002.
Analysis of the patterns of large fires in the boreal forest region of Alaska. International Journal of Wildland Fire 11: 131-144.
Miller, M. 1985.
Fire occurrence in the Northwest Planning Area, 1956-1982. Open File Report 15, Bureau of Land Management, Anchorage, Alaska.
Racine, C.H., W.A. Patterson III, and J.G. Dennis. 1985.
Tundra fire regimes in the Noatak River watershed, Alaska: 1956-1983. Arctic 38: 194-200.
Rupp, T.S., M. Olson, L.G. Adams, B.W. Dale, K. Joly, J. Henkelman, W.B. Collins, and A.M. Starfield. 2006.
Simulating the influences of various fire regimes on caribou winter habitat. Ecological Applications 16: 1730-1743.
Stafford, J.M., G. Wendler, and J. Curtis. 2000.
Temperature and precipitation of Alaska: 50 year trend analysis. Theoretical and Applied Climatology 67: 33-44.