Most of the land area in the five National Park Service units in the Arctic Inventory and Monitoring Network (ARCN) is underlain by permafrost. Permafrost is ground so cold that it stays frozen for multiple years. Certain landforms develop when permafrost thaws, and they provide a way to recognize and monitor permafrost thaw by remote sensing.
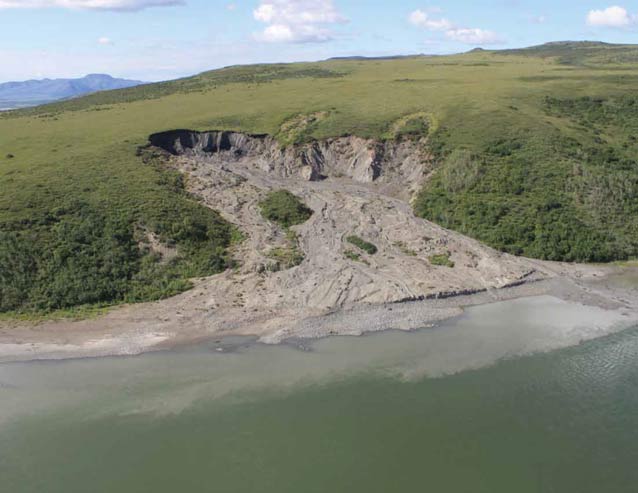
At ARCN we are monitoring four types of permafrost landforms as a window into the effect of climate change on our permafrost. These landforms are active-layer detachments, retrogressive thaw slumps, degraded ice-wedge polygons, and thermokarst lakes.
Active-layer Detachments
Active-layer detachments (ALD) are small landslides that occur on vegetated slopes. A surface layer roughly 3’ (1 m) thick slides as a unit and accumulates as a winkled mass below. ALD are typically 30’-90’ (10 to 30 m) wide and from tens to several hundred yards/meters long. The slide leaves an elongated region of bare soil exposed on a slope, which can lead to erosion of sediment into streams (Lamoureux and Lafrenière 2009). ALD usually occur after unusually warm summer weather (Carter and Galloway 1981; Lewkowicz and Harris 2005). Thaw of the ice-rich layer that is often present in the upper permafrost produces a mud slurry that lubricates the slide (Lewkowicz 2007).
My survey of ARCN using satellite images taken between 2006 and 2008 identified over 2200 active-layer detachments, mostly in the Noatak National Preserve and Gates of the Arctic National Park and Preserve (Swanson 2012 and unpublished data), many of these ALD have re-vegetated and few new ones have formed. Climate data suggest that the unusually warm summer of 2004 triggered a large number of ALD (Swanson 2012). Many of our most slide-prone locations may have released in this event and be more stable now. But we will continue to watch for summer warm spells that could trigger ALD formation in new places, as thaw reaches depths where layers of ground ice persist.
Retrogressive Thaw Slumps
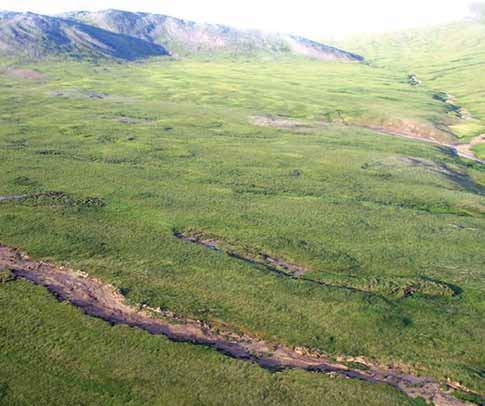
Retrogressive thaw slumps (RTS) occur where a steep cut-bank in ice-rich permafrost advances into undisturbed ground as material thaws (Burn and Lewkowicz 1990. While ALD impact only the top 1 m or so of soil, the eroding cut-bank of a RTS is typically 2 to 10 m high. RTS often begin as an escarpment on a lakeshore or riverbank. As they advance by thawing and slumping, they can shed large amounts of sediment into the adjacent water body (Kokelj et al. 2005; Bowden et al. 2008).
My recent survey of RTS in the Noatak National Preserve and Gates of the Arctic National Park and Preserve using high-resolution satellite images from 2006-2008 detected about 700 slumps (Swanson 2012 and unpublished data).
RTS in these two parks are found mainly on glacial deposits, and they develop by thaw of glacial ice that has persisted underground for tens of thousands of years. The largest of these slumps cover over 12 acres (5 ha) and have escarpments up to 20 m high. We have monitored the growth of 22 RTS in NOAT and GAAR for years beginning in 2010, by creating 3-dimensional models from aerial photographs shot at close range from the window of a helicopter or airplane (Swanson 2013). The 3-D models are used to create detailed maps of the slumps and to measure slump growth.
Is the current level of RTS activity in ARCN an unprecedented result of recent climate warming, or simply the continuation of a process that has gone on for centuries? In a 73 mi2 (190 km2) study area in the eastern part of NOAT, I compared the number and area of RTS visible on 2008 high-resolution satellite images with aerial photographs of the same area taken in 1977 (Swanson 2012). I identified 23 RTS covering a total of 33 acres (13.5 ha) on the 1977 photos, and 35 RTS covering 52 acres (21.2 ha) on the 2008 satellite image. The slumps visible on the 1977 photos were already up to 1,300’ (400 m) long, which according to our measured rates of growth shows that they must have started growing before the beginning of the current warm climatic phase that began in the mid 1970s (Hartman and Wendler, 2005). Thus RTS were active before the post-1970s warming began, but they may be more prevalent now than before. We expect any future climate change that causes summer thaw depths to penetrate deeper will trigger new RTS and faster growth of existing RTS.
Ice-wedge Polygons
Ice wedge polygons form by contraction cracking of frozen ground in the winter. The cracks fill with meltwater the following spring; the meltwater freezes and is preserved in permafrost. After many cycles of cracking, filling, and freezing, the ice wedges can grow to a meter or more wide at the top, and create a distinctive polygonal pattern visible from the air. As the wedges grow, the ground adjacent to the wedge buckles upward, creating what is known as a “low-centered polygon”, because the center is lower and typically wetter than the margin near the wedge.
Ice wedges are quite vulnerable to thaw because pure ice is present with little overlying insulation. When ice wedges degrade, the polygon margins subside and the polygons become “high-centered ice-wedge polygons&rdquo.
With a warming climate, we expect to see widespread ice wedge degradation. There is good evidence that this process began in the late 1900s around Alaska (Jorgenson et al, 2006). At the present time most ice-wedge polygons in ARCN are of the low-centered type, but we see some areas where ice-wedge degradation has produced high-centered polygons. We are collecting high-resolution imagery of polygon-rich terrain in the lowlands of ARCN to monitor future changes in ice wedges.
Thermokarst Lakes
Thaw of ground ice can cause subsidence, a process known as thermokarst. The depressions resulting from thermokarst are often occupied by lakes and ponds. Thaw of permafrost can cause thermokarst basins to enlarge, but it can also cause lake drainage by breaching the bank. In addition, like any other water body, thermokarst lakes can gain or lose water by climate-driven changes in the balance between precipitation and evaporation. At ARCN we are monitoring changes in the surface area of water bodies using Landsat satellite images. Several areas of thermokarst lakes in ARCN have shown marked declines in water area since the year 2000 (Figure 8; Jones et al. 2011; Swanson 2013). Much of the decline in thermokarst lake area is due to abrupt lake drainage events. Images from future years will show if this trend is continuing.
Conclusions
There is widespread but as yet subtle evidence for landscape changes due to permafrost degradation in Alaska’s national parks. If permafrost thaw accelerates in the arctic, we expect a variety of significant changes to occur, including delivery of more sediment and solutes to water bodies from ALD and RTS, continued declining area of lakes, and overall drying of the lowland landscape as ice wedges degrade and summer thaw depths increase. At ARCN we will continue our monitoring of landforms produced by thaw of permafrost to keep abreast of these potential changes.
Part of a series of articles titled Alaska Park Science - Volume 12 Issue 2: Climate Change in Alaska's National Parks.
Last updated: August 11, 2015