Abstract
We used a modeling framework and a recent ecological land classification and land cover map to predict how ecosystems and wildlife habitat in northwest Alaska might change in response to increasing temperature. Our results suggest modest increases in forest and tall shrub ecotypes in Northwest Alaska by the end of this century thereby increasing habitat for forest-dwelling and shrub-using birds and mammals. Conversely, we predict declines in several more open low shrub, tussock, and meadow ecotypes favored by many waterbird, shorebird, and small mammal species.
Introduction
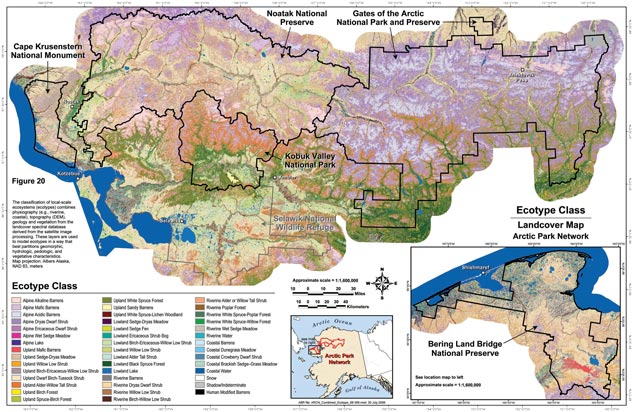
The Arctic is changing faster in response to climate warming than other places on earth. But what will this change mean to the ecosystems and wildlife populations that are found in the far north of Alaska? By studying the changes that have already occurred there, we can anticipate how future climate change could affect the plants and animals that make up this unique part of the world.
To address this issue, the WILDlife Potential Habitat ForeCASTing Framework, or WildCast, was begun as a collaboration between the National Park Service and the U.S. Geological Survey to develop a predictive framework for ecosystems and wildlife habitat in Northwest Alaska. The study area includes the five national park units that make up the Arctic Inventory and Monitoring Network: Gates of the Arctic National Park and Preserve, Noatak National Preserve, Kobuk Valley National Park, Cape Krusenstern National Monument, and Bering Land Bridge National Monument, as well as the adjacent Selawik National Wildlife Refuge of the U.S. Fish and Wildlife Service (Figure 1). The basic premise of the project is to develop methods and tools that, in the face of limited data, can be used to better understand how climate change might influence ecosystems and the habitats of birds and mammals that inhabit this arctic landscape. The products from WildCast will enable land managers in Northwest Alaska to visualize potential future changes to lands and resources under their jurisdiction, and to help identify and prioritize management, inventory, monitoring, and research needs. WildCast also complements the cooperative scenario planning efforts in Alaska by the National Park Service and Scenarios Network for Alaska Planning (SNAP) (see Winfree et al. in this issue).
Previously, Marcot (2009) summarized some of the challenges in undertaking a project such as this and discussed modeling approaches that can be used to accommodate the uncertainties that inevitably arise in forecasting future ecological scenarios in a data-poor world. In this contribution, we provide a brief overview of progress on this complex and challenging project.
WildCast Vision and Objectives
WildCast is intended to help anticipate how climate change could affect species, communities, wildlife habitats, and ecosystems in Northwest Alaska over the next century. We have three principal objectives:
- model probable changes in the areal extent of ecosystem types based on historical changes relative to time and regional air temperature;
- identify likely changes in percent and total area of wildlife habitats;
- and facilitate identification of critical research priorities to improve model outcomes.
Methods
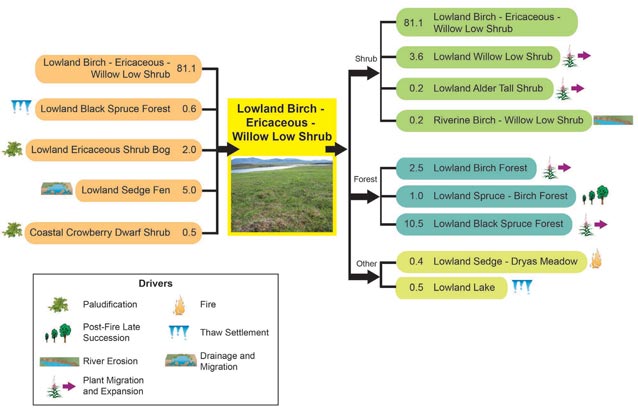
At the outset, we planned to base our analyses for WildCast on a limited number of land cover types generated from LandSat imagery. However, the availability of a new ecological land classification and land cover map for our study area (Jorgenson et al. 2009; Figure 1) allowed us to expand our analysis to include 60 vegetation land cover types (hereafter, “ecotypes”). To predict future changes in ecosystem abundance, we used a five-step modeling process. First, we analyzed historic trends in mean annual air temperature for selected weather stations located within or near the study area. Second, we compiled data on historical rates of ecosystem change during the last 30–50 years from previous studies in the region, with particular emphasis on the recent comprehensive analyses for the Arctic National Wildlife Refuge (Jorgenson et al. 2011) and the Arctic Network of National Parks (Swanson 2012). Third, data from the individual studies were averaged and adjusted to develop transition probabilities that encompass all the potential transitions from one ecotype into other ecotypes that could result from differing ecological drivers (e.g., fire, thermokarst, and primary succession). Fourth, the transition probabilities were extrapolated into the future for three time periods 2010–2040, 2040–2070, and 2070–2100 where transition probabilities were held constant for all three periods, and temperature, where past transition probabilities for a 1.8°F (1°C) temperature change found in our temperature analyses were linearly extrapolated to predicted temperature changes of 3.6, 7.2, and 10.8°F (2, 4, and 6°C) for the three future periods, respectively. The predicted future changes in mean annual air temperatures for the region were based on the regional projections for Northwest Alaska from the Scenarios Network for Alaska Planning (www.snap.uaf.edu). Fifth, the changes in ecotypes were calculated using the transition probabilities for each time period and the areas at the end of the previous period as the input for the next period. This produced changes as functions of time (fixed rate), temperature (rapidly increasing rate), and an average of time and temperature.
Comprehensive quantitative information on wildlife-habitat associations is unavailable for most mammal and bird species that reside in the study area. We recognized this critical data gap, but still needed some way to link species to the ecotypes they live in. Thus, we expanded on an approach used by The Nature Conservancy (TNC) for the North Slope of Alaska. We denoted habitat use of each ecotype by each species on an ordinal scale of 0 (none/negligible), 1 (low), 2 (medium), and 3 (high use) based on a qualitative synthesis of available data. To ensure that TNC’s approach was transferable to our study area, we cross walked and embellished their list of ecotypes to those from Jorgensen et al. (2009), and added species that occur in Northwest Alaska. Because our study projects future points in time, we added species that currently occur near, but not within, the study area, but that might move in if suitable habitat exists there sometime in the future. Species experts provided and reviewed our bird and mammal habitat use assignments. Next, total habitat area for each species was determined by tallying associated ecotypes, for each time period. In this way, we identified individual ecotypes, and species-specific habitats with significant gains and losses in extent within the study area, under historic temperature change rates extrapolated into the future. Our model provides a framework for easily updating any of the parameters for conducting sensitivity analyses or for incorporating improvements or variants in any of the parameter values such as different future temperature scenarios.
Results
Changes in Ecotypes
Future ecological transitions are based on 60 ecotypes found within the study area. We identified 243 potential ecological transitions that involve changes from one ecotype into another due to geomorphic and ecological processes that are likely to be influenced by climate change. A summary of key findings follows below, with detail on additional ecotypes and species provided in forthcoming publications. A few ecotypes show only one reasonable transition possibility (staying the same, e.g. from Alpine Lake to Alpine Lake), while others showed more. For example, Upland Dwarf Birch-Tussock Shrub showed the maximum number, with 11 potential transitions, due to the numerous drivers that can affect change. Figure 2 illustrates how multiple ecotypes can potentially transition into a single ecotype (in this example Lowland Birch-Ericaceous-Willow Low Shrub), which then can transition into other ecotypes depending on differing ecological drivers. Over a century-long period, an area can be affected by multiple drivers; for example Upland Dwarf Birch-Tussock Shrub can be replaced by Upland Barrens-Thermokarst, then by Upland Alder-Willow Tall Shrub (early succession), and finally by Upland White Spruce Forest (late succession).
Transitions from one ecotype into another have been documented for many ecotypes in Northwest Alaska and other parts of the Arctic (Figures 3 and 4). Based on a comprehensive compilation of data on historical rates of change, our work shows that nearly all ecotypes (56 of 60) will undergo some change in area during the next century across the study area. Ecotypes that currently occupy large areas (>657.37 sq mi or 1,700 km2) that are likely to experience major losses in area include Upland Birch-Ericaceous-Willow Low Shrub (due to thermokarst, fires, and shrub and forest expansion), Lowland Birch-Ericaceous-Willow Low Shrub (post-fire succession and forest expansion), Upland Dwarf Birch-Tussock Shrub (thermokarst, fires, and shrub and forest expansion), Upland Sedge-Dryas Meadow (thermokarst, shrub expansion, and acidification), and Lowland Alder Tall Shrub (forest expansion)(Figure 5a; also see Figures 6a-c). Conversely, ecotypes that are likely to show major increases include Lowland Black Spruce Forest (forest expansion and post-fire succession), Upland Alder-Willow Tall Shrub (shrub expansion), Lowland Willow Low Shrub (shrub expansion and soil drainage), Upland White Spruce Forest (forest expansion), and Upland Willow Low Shrub (primary succession after thermokarst)(Figure 5a; also see Figures 6d-f).
Several ecotypes that now cover relatively small areas show potential for large future increases when calculated as a percentage of their current areas, including: Upland Bluejoint-Herb Meadow (due to fires), Lowland Birch Forest (thermokarst, fires), Upland Aspen Forest (warming south-facing slopes), Upland Barrens-Thermokarst, Lacustrine Willow Shrub (lake drainage), and Lacustrine Barrens (lake drainage). Conversely, other Ecotypes show potential for large percentage reductions, including: Riverine Dryas Dwarf Shrub (shrub expansion), Upland Birch Forest (post-fire late succession), Upland Barrens-Landslides (early succession), and Alpine Snowfields and Glaciers (melting).
Changes in Wildlife Habitat
We assessed potential future changes in the habitat of 36 mammal species and 162 bird species based on medium and high use levels.
The largest percentage habitat gains for mammals through this century are for species that live in forests or use shrubs, including red squirrel, northern flying squirrel, porcupine, American marten, and moose, with gains in overall habitat area for these species exceeding 20%, and with lesser gains for black bear and northern bog lemming. Nearly all other mammals show various levels of decline in habitat ranging up to about 12% loss by the end of the century (Figure 5b), largely due to expected decline in Lowland Alder Tall Shrub, Lowland Birch-Ericaceous-Willow Low Shrub, Riverine Dryas Dwarf Shrub, Upland Birch Forest, Upland Birch-Ericaceous-Willow Low Shrub, and Upland Sedge-Dryas Meadow. Of note is the potential decline in habitats of Alaska hares, ground squirrels, lemmings, voles, and shrews, comprising the set of small mammal prey species important to mesocarnivores of the region, habitat for which is also projected to decline. Among all 36 mammal species analyzed, seven show an increase in habitat area, 28 a decrease, and one with no change.
Many waterbird species show various percentage increases in habitat, with shorebirds being about equally divided among those showing increases and decreases, and many forest- or shrub-dwelling raptors, passerines, and others showing large percentage increases exceeding 30% (Figure 5c). Among the greatest losers is a mix of waterbirds, shorebirds, and raptors, mostly because of expected declines in Coastal Brackish Sedge–Grass Meadow, Lowland Birch-Ericaceous-Willow Low Shrub, Lowland Lake, Riverine Dryas Dwarf Shrub, and Upland Sedge-Dryas Meadow. Among all 162 bird species analyzed, 99 show an increase in habitat area, 59 a decrease, and 4 show no change.
Also considered are wildlife species not currently present but that might move northward into and expand within the study area under future increases in some ecotypes. These include meadow jumping mouse, hairy woodpecker, red-breasted nuthatch, and ruffed grouse.
Key Assumptions and Uncertainties
Our projections of ecotypes are based on the linear extrapolation of historical rates to future time periods based on time (rates stay constant for each 30-yr period) or temperature (using predicted increases relative to historical temperature increase), using published studies of past changes and expert knowledge to forecast rates of future transitions. While the predictions are based on substantial observational records of past changes, there are numerous factors that affect the accuracy of the predictions. First, errors in the classification of ecotypes can occur with both the change detection interpretation conducted from these published studies and with the ecotype map for the study area (Jorgenson et al. 2009) that serve as the basis for quantifying the initial extent of ecotypes. The classification accuracy typically is 80% for photo-interpreted studies and the classification accuracy of the ecotype map was estimated to be between 65-80% for 41 ecotypes, indicating there is substantial error associated with detecting and mapping change. Second, while transition probabilities for the common ecotypes are supported by previous research, the transitions for uncommon types frequently relied on expert opinion. These transition probabilities are derived, in part, from other regions of Alaska and may vary in their applicability to our study area. Third, we recognize that other facets of climate change, such as changes in annual and seasonal precipitation, are also expected to force ecosystem changes. Finally, it is important to note that ecotypes, in themselves, do not respond to the environment, but are comprised of assemblages of species that respond individually to stressors and environmental change. The ecotype classification system is directed at identifying change in the dominant species that are used to characterize the ecotypes. Changes in dominant species during forest succession differentiated by the classification system can also capture some of the changes in other species, because understory species often are associated with dominant species in the canopy. Furthermore, large changes in the environment, such as from lake to barren drained-lake basin, can cause wholesale shifts closely associated plant assemblages.
The wildlife habitat projections are based on the assumption that use of individual ecotypes by a species is independent and equivalent; that is, we do not denote type of use (e.g., for breeding, feeding, resting, or migration) nor how the quality and spatial patterns of habitats contribute to population persistence, mostly because such data do not yet exist. The wildlife species-habitat relationships for Northwest Alaska were based on a combination of expert knowledge and limited field surveys. Our wildlife habitat projections should not be interpreted as expected changes in population size or trend of each species, which would require as-yet unavailable demographic data. We also recognize that availability of potentially suitable habitat does not ensure that it will be occupied, as human-caused disturbances and other factors also influence wildlife distributions.
Conclusions
This is the first evaluation of its type for boreal and tundra ecosystems that provides a comprehensive assessment involving the full diversity of ecosystems across a broad region. Overall, we view the results as a valuable tool for posing testable hypotheses of changes in ecotypes and species’ habitats; as a means of identifying potential priorities for management, inventory, monitoring, and research activities; and as basis for improvement over time as new data become available.
Acknowledgments
This project was the outcome of discussions among Leslie Holland-Bartels, Carl Markon, and Robert Winfree on how climate change might affect the natural resources of Alaska’s pational parks. Janet Jorgenson, U.S. Fish and Wildlife Service, and Dave Swanson, National Park Service, generously shared their change detection data for the Arctic National Wildlife Refuge and the Arctic Network of National Parks, respectively. We thank Colleen Handel, Andrew Hope, Dave Gustine, Link Olson, and Dan Ruthrauff for reviewing our bird and mammal ecotype use associations. Carl Roland and John Pearce provided helpful reviews of this manuscript. This project was funded through the Natural Resources Preservation Program (NRPP) of the USGS’s Ecosystems Mission Area.
Part of a series of articles titled Alaska Park Science - Volume 12 Issue 2: Climate Change in Alaska's National Parks.
Tags
Last updated: April 27, 2016