Abstract
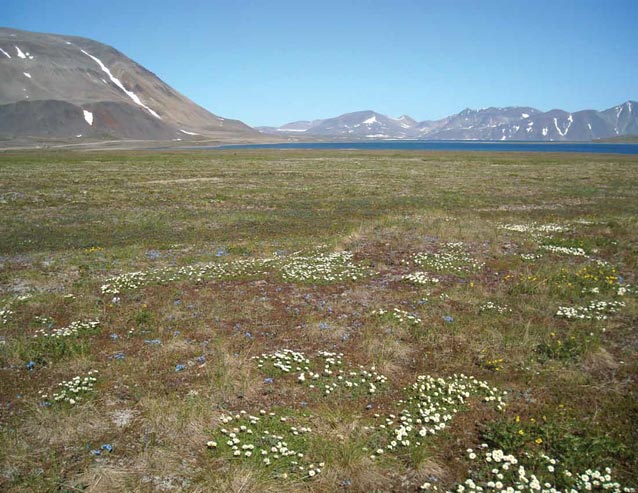
Photograph courtesy of Nadya Sekretareva
The history and geography of the arctic flora in Beringia has been complex—influenced by glacial retreats during the Quaternary, exchange via the Bering Land Bridge, in situ survival in refugia, and differing climatic regimes. Much of the details of these diversifications in Beringia are still lacking and to begin to address this issue we provide results from stochastic character mapping reconstruction to recover historical signals from occurrence data at the Herbarium, University of Alaska Fairbanks. A taxon matrix of 13 selected ecoregions in Beringia and 1549 extant vascular plant species was constructed and analyzed with RAxML and Mesquite software. The flora of Western Beringia appears younger than that of Eastern Beringia, with the ecoregions in Western Beringia derived from within those of Eastern Beringia. The Seward Peninsula ecoregion shares the most taxa with the ecoregions from Chukotka that form a clade. The Seward Peninsula is also the richest ecoregion, with 777 taxa recorded, a sharp contrast to the impoverished Bering Sea Islands ecoregions, where only 276 taxa are recorded. Overall, when examining stochastic mapping reconstructions, current species distributions in Beringia have been independently shaped by dispersal, extinction, and in some cases vicariance events due to ecological or physical barriers (e.g., Bering Strait). Mid-July temperature and precipitation differ across Beringia and at same latitudes, presenting a driver or “climatic barrier” for the overall ecosystem setup. Our study shows that distributional museum data can be informative in generating testable hypotheses on the history and evolution of the flora in an area such as Beringia.
Introduction
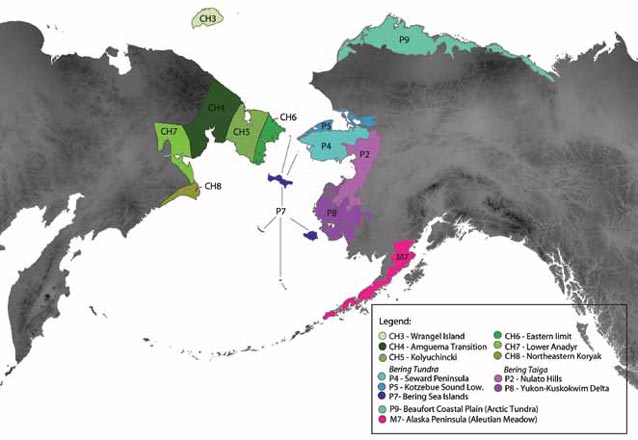
It should be stressed that the flora on both sides of the Bering Strait is identical with few exceptions. This is what can be expected as the present northern Bering Sea more than once during its Pleistocene history has been dry permitting dispersal of plants from one continent to the other. (Eric Hultén 1937).
Alaska and the Yukon immediately present the picture of a floristic appendage to Asia… However, a closer scrutiny of the flora… demonstrates that even in the arctic parts of this territory there are a significant number of purely American taxa. Asian and American portions of the Beringian sector must be referred to different provinces. (Boris Yurtsev 1972).
It is interesting to note that authorities such as Eric Hultén and Boris Yurtsev had very differing views about the history of the Beringian Flora that continue to spur investigations into the origin and evolution of this flora. While Hultén has emphasized taxonomic similarities between eastern and western Beringia, Yurtsev emphasizes more of their differences. A major contribution to today’s flora arrived in Alaska via the Bering Land Bridge as it was exposed during successive intervals of continental glaciation (Ice Ages) and retreat (Hultén 1937). The Arctic portion of this flora is of relatively recent origin (Murray 1995). Traditionally, investigations of the Arctic have emphasized Beringia’s role in the intercontinental exchange of fauna and flora (Ickert-Bond et al. 2009). Exchange was characterized as asymmetric (Hopkins 1967) with most taxa originating in Northeastern Asia and moving to North America (Flerow 1967). In contrast, eastern Beringia (Alaska) was effectively isolated from the rest of North America by the Laurentide Ice Sheet (Pielou 1991) and therefore was then the easternmost extent of an Asian biome.
Eastern Chukotka along with Wrangel Island are hotspots of biodiversity in the Russian Arctic (Kholod 2007), harboring six [Carex norvegica ssp. cornicorostrata, Puccinellia beringensis, X Pucciphippsia czukczorum, Oxytropis beringensis, O. middendorffii ssp. submiddendorffii, Cardamine sphenophylla] and ten unique arctic endemic species [Poa hartzii ssp. vrangelica, Puccinellia wrightii ssp. colpodioides, Papaver multiradiatum, Papaver chionophilum, Oxytopis uschakovii, Oxytropis uniflora, Potentilla wrangelii, Packera hyperborealis ssp. wrangelica, Silene sorensensis, Potentilla uschakovii] respectively, as compared to four in Western Alaska [Ranunculus glacialis ssp. alaskensis, Parrya nauruaq, Primula anvilensis, Douglasia beringensis] and seven [Symphyotrichum pygmaeum, Mertensia drummondii, Puccinellia banksiensis, Poa ammophila, Puccinellia vahliana, Papaver “murrayi”, Poa hartzii ssp. alaskana] in Northern Alaska, based on a total of 28 Arctic endemic species known only to occur in one of the six Beringian floristic provinces (Daniëls et al. 2013).
While Chukotka has a particular meaning for understanding the floristic affinities of the Alaskan flora (Yurtsev et al. 2010), and vice versa, there has not been a formal comparison of these areas to understand how dispersal, vicariance, and climate have shaped the assemblage of these floras. Clearly, plant distributions are heavily driven by climate (typically expressed as temperature and precipitation). In the absence of detailed on-the-ground measurements, ecoregions have historically been defined to summarize ecological climate regimes relevant for plant occurrence and growth (Holdridge 1947, 1966) and latitudinal biodiversity gradients have been discussed widely (Ricklefs 2004; Wiens and Donoghue, 2004; Roy and Goldberg, 2007).
For Beringia, we currently find no consistent ecoregional classification scheme, and here we confront the best available ecoregion polygons for Alaska (Nowacki et al. 2001) and Chukotka (Yurtsev et al. 2010) with georeferenced specimen data (Arctos, http://arctos.database.museum/) as well as with climate data layers (temperature and precipitation) for an assessment and new insights of the climate niche for plants and their ancestors. Species have climatic preferences that can be correlated with their presence or absence in different areas and will be influenced by changing climate in the future (Young 1971; Edlund and Alt 1989, Daniëls et al. 2000; Loarie et al. 2008; Ackerly et al. 2010; Hof et al. 2012). Thus, the comparison of quantified climatic envelopes between regions can shed some light on the underlying processes affecting species composition in a particular region.
Here we use information of species diversity based on occurrence records and climatic data of the different ecoregions of Beringia (Figure 2) to explain the differences or similarity of these areas and infer hypotheses of historical biogeography. These regions are not congruent with the floristic zones and subzones as employed by the CAVM Team 2003 (Walker et al. 2005), rather the floristic zones used here reflect extensive field data assembled by Boris Yurtsev (1978) for the Russian Far East as well as those from Nowacki et al. (2001) for Alaska. While not consistent across Beringia, we chose those classifications because they are considered to be accurate, summarize ecoregions, are suitable for the sub- and arctic regions, and are the best we have available. This study will help to understand the history and the possible underlying causes of species diversification in Beringia in general, and the Arctic in particular, using ecoregions and climate data as a measure of diversity between Eastern and Western Beringia.
Materials and Methods
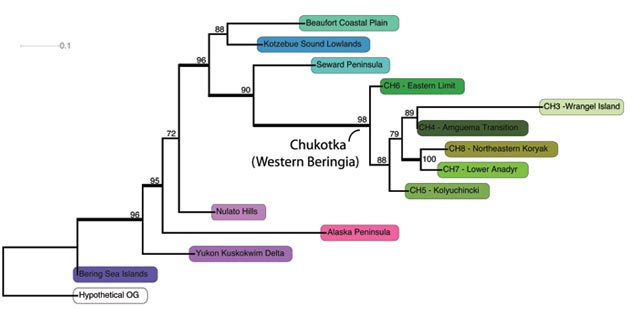
1. Fieldwork in Alaska (long-term) and in Chukotka (2010)
In the early 1990s the Herbarium (ALA) at the University of Alaska Museum secured many arctic specimens with support from the National Science Foundation’s (NSF) International Program, the National Park Service’s (NPS) Beringia Program, and from collaboration with Russian scientists. Critically important to recent work on the Panarctic Flora are collections from the Russian Far East and central Siberia acquired mostly from the Komarov Botanical Institute in St. Petersburg and the Central Siberian Botanical Garden in Novosibirsk, but also from our own collecting in the Altai and Sayan Mountains of south-central Siberia (by David Murray) and Chukotka (by Carolyn Parker). Recently, with support from the National Science Foundation and the NPS’s Shared Beringia Program, we carried out a detailed collecting program of Beringian plants to the Chegitun River, eastern Chukotka, in the summer of 2010 in collaboration with the Komarov Botanical Institute, St. Petersburg. This was done to compare with taxa from long-term collecting efforts on the Seward Peninsula in Alaska.
2. Ecoregion classification for Alaska (a) and for Chukotka (b)
a) Mapping ecosystems in Alaska has a long history. Initial attempts were trying to interpret terrain and vegetation using black and white aerial photography (Spetzman 1963). In the 1980s, remotely sensed data and a better understanding of ecosystem processes lead to global efforts of mapping ecosystems, resulting in two different maps for the state of Alaska (Gallant et al. 1995, Nowacki and Brock 1995). In an effort to unify ecoregion boundaries for Alaska, an interdisciplinary, interagency, and international team came together and produced the Unified Ecoregions of Alaska map (Nowacki et al. 2001; https://agdcftp1.wr.usgs.gov/pub/projects/fhm/akecoregions.htm). A total of 32 ecoregions were delineated within the state of Alaska based on criteria such as climate, physiography, vegetation, geology/surficial deposits, and glaciation. These were then integrated in a digital GIS (Geographic Information Systems, ArcGIS vers. 10) approach.
b) For the floristic division of Chukotka we are following a map that was compiled by Boris Yurtsev and his colleagues at the Komarov Botanical Institute of the Russian Academy of Sciences in St. Petersburg. There are numerous Russian publications that reflect the intense and long-standing scientific effort in the floristic description and subdivision of the Arctic; few of these works have been translated into English (Razzhivin 1999). Yurtsev’s work is based on a solid and extensive body of primary field data (Yurtsev et al. 1978). Using these data, Yurtsev developed an elaborated analytical approach with a range of criteria that have to be well balanced with each other in order to define the borders between the provinces or sub-provinces. The map presented here is therefore a map based on a qualitative approach (Yurtsev 1974, Yurtsev et al. 1978, Yurtsev 1994). While the output is not directly repeatable, similar to Viereck’s Alaska Vegetation Classification (Viereck et al. 1992), and not based on modern methods either like a Geographic Information System (GIS) geo-databases or underlying online databases and latest non-linear statistics, it represents the authoritative and robust classification scheme used in Chukotka and parts of the Russian Arctic.
3. Taxon-area analysis
We extracted geo-referenced records (103,074) from the UA Museum herbarium database http://arctos.database.museum/SpecimenSearch.cfm for ecoregions in Alaska (Nowacki et al. 2001), from the checklist of Flora of the Chukotkan Tundra (Yurtsev et al. 2010) as well as the Checklist of Wrangell Island (Petrovsky 1988) and constructed a presence-absence taxon matrix for selected Beringian ecoregions (Figure 2). Taxonomy follows the Panarctic Flora http://nhm2.uio.no/paf/. The dataset was examined in a Maximum likelihood framework using a Markov k model and Gamma distribution rates of heterogeneity in RAxML (Cipres Portal, www.phylo.org/sub_sections/portal/). All analyses included an all-zero hypothetical artificial outgroup for rooting purpose (Hypothetical OG; Rosen 1988; Cracraft 1991; Morrone 1994). Using the resulting taxon-area cladogram as the basis for stochastic key species reconstruction in the program Mesquite http://mesquiteproject.org/mesquite/mesquite.html we generate testable hypotheses about the history of plant populations in Beringia that can be explored with molecular sequencing data in the future.
4. Climate data assessment
For consistency, we used the publicly available data from the WorldClim data set (www.worldclim.org). For the months of July and January we used long-term means of temperature and precipitation to indicate ‘climate’. These data were re-projected in ArcGIS in a Mercator projection for the study area (Herrick et al. 2013), and we overlaid them with hand-traced shapefiles for the Ecoregions for Chukotka (Yurtsev et al. 2010) and Alaska (Nowacki et al. 2001). We used the Geospatial Modeling Environment (GME; Hawths tools) to extract data, and R and SPLUS for creating boxplots to show medians and 95% confidence intervals for each of the ecoregion polygons (subsampled).
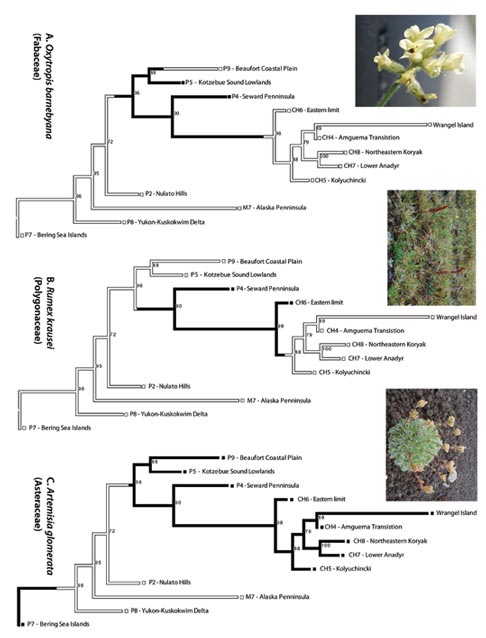
Figure 4. Stochastic character mapping of Eastern Berin-gian endemic Oxytropis barnebyana (at left), a narrowly amphiberingian rare Rumex krausei (in center) and wide-spread amphiberingian Artemisia glomerata (at right). Inset photographs of O. barnebyana, Alaska: Seward Peninsula; R. krausei, Chukotka Autonomous Region; Node support (ML Bootstrap) is indicated next to each node. Character states indicated by boxes at the terminals (black = presence, white = absence). Visualization of character changes along branches and not just at the nodes is indicated by colored branches (inferred under maximum likelihood in Mesquite).
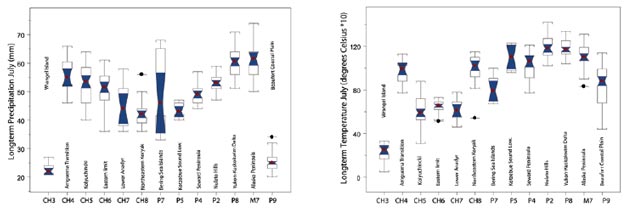
Location | Total number of taxa found | Percentage | Total number of unique taxaa found | Percentage |
---|---|---|---|---|
Alaska Peninsula | 685 | 44 | 167 | 11 |
Kotzebue Sound Lowlands | 445 | 29 | 11 | 1 |
Bering Sea Islands | 276 | 17 | 10 | 1 |
Nulato Hills | 425 | 27 | 12 | 1 |
Seward Peninsula | 777 | 50 | 75 | 5 |
Yukon-Kuskokwim Delta | 341 | 22 | 12 | 1 |
Beaufort Coastal Plain | 420 | 27 | 22 | 1 |
Chutkoka 4 - Amguema Transition | 487 | 31 | 1 | 0 |
Chutkoka 5 - Kolyuchincki | 485 | 31 | 3 | 0 |
Chutkoka 6 - Eastern Limit | 611 | 39 | 16 | 1 |
Chutkoka 7 - Lower Anadyr | 596 | 38 | 21 | 1 |
Chutkoka 8 - Northeastern Koryak | 481 | 31 | 5 | 0 |
Chutkoka 9 - Wrangel Island | 352 | 23 | 27 | 2 |
Taxa found in all four egoregions examined:
Anemone richardsonii, Arctagrostis arundinacea, Artemisia tilesii subsp. tilesii, Cerastium beeringianum var. beeringianum, Equisetum arvense, Festuca rubra subsp. rubra, Hiero-chloë alpina, Luzula kjellmaniana, Pedicularis capitata, Pedicularis verticillata, Poa arctica subsp. arctica, Poa pratensis subsp. alpigena, Pyrola grandiflora, Ranunculus hyperboreus subsp. hyperboreus, Ranunculus nivalis, Salix arctica, Salix reticulata, Saxifraga hirculus, Tofieldia coc-cinea, and Valeriana capitata
Results
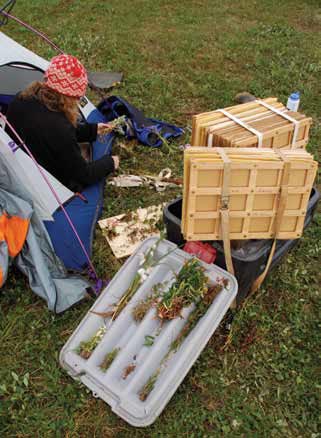
The matrix contained 1,549 unique taxon names for the regions examined. Many are shared across both Alaska and Chukotka, while there are a number of unique taxa found only within one ecoregion (Table 1). The richest communities are the Seward Peninsula (777 taxa), the Alaska Peninsula (685 taxa, albeit not in the Arctic) and the Chukotkan 6 – Eastern Limit region with 611 taxa recorded (Table 1). The regions with the least number of taxa found are the Bering Sea Islands in Alaska with 276 taxa and the Yukon-Kuskokwim Delta with 341 taxa each and Wrangel Island with 352 taxa recorded (Table 1). Only twenty taxa have such broad ecological requirements that they are able to persist in all 13 ecoregions examined (Table 2).
Maximum likelihood (ML) analysis in RAxML unambiguously groups the Chukotkan ecoregions together (ML bootstrap support 100%), which are derived from within the AK ecoregions (Figure 2). Support values (ML bootstrap support – ML BS) for all clades are strong across the topology (Figure 3). The Seward Peninsula ecoregion shares the most taxa with the ecoregions from Chukotka that form a clade (ML BS 98%) and forms a well-supported sister group to the Chukotkan ecoregions (ML BS 90%, Figure 3). The ecoregions of Northeastern Koryak (CH8) and Anadyr (CH7) are among the most recently formed in Western Beringia (ML BS 100%, Figure 3), and are characterized by the presence of many common species. They are both members of Yurtsev’s Southern Chukotka subprovince (1978).
Similarly, Wrangel Island (CH3) and the Anguema Transition (CH4) are of recent origin and form a clade (ML BS 89 %, Figure 3). The floristic composition of the Anguema Transition is very closely related to that of the clade (ML BS 100 %) composed of Northeastern Koryak (CH8) and Anadyr (CH7), which can be deduced from the short branch lengths leading to the Anguema Transition in Figure 2. In contrast, Wrangel Island is characterized by numerous endemic species as evidenced by a very long branch leading to Wrangel Island representing numerous taxa unique to the flora of Wrangel Island (Figure 3-4).
We have selected a few interesting species maps to demonstrate patterns of diversification in Beringia (Figure 4A-C). Each map represents one of a large number of possible species histories. Figure 4A shows stochastic mapping reconstruction of Oxytropis barnebyana, an Eastern Beringian endemic and suggests that the species may have been present in the entire ancestral area of the Beaufort Coastal Plain (P9), the Kotzebue lowlands (P5), the Seward Peninsula (P4), and its derived clade of Western Beringia. It may have subsequently become extinct in the Beaufort Coastal Plain and Western Beringia. Alternatively, O. barnebyana might have been present in the ancestral area of the Beaufort Coastal Plain (P9), the Kotzebue lowlands (P5), the Seward Peninsula (P4), and dispersed from the Seward Peninsula into the adjacent Kotzebue lowlands (P5) only, but the Bering Strait posed a strong barrier to dispersal for these plants into Western Beringia where they are absent today. Figure 4B shows the stochastic mapping reconstruction for the narrowly amphiberingian Rumex krausei, currently restricted to wet calcareous tundra on the Seward Peninsula (P4) and the Chukotkan Eastern Limit (CH6). The reconstruction shows that it was present in the ancestral area of the remaining five Chukotkan ecoregions of Western Beringia, but perhaps failed to establish there. Extinction might explain the absence in these Western Beringia ecoregions. Stochastic mapping reconstruction of the widespread amphiberingian Artemisia glomerata shows species occurrence in more nested ancestral areas (Figure 4C). The species diverged in the ancestral areas of the Western Alaska ecoregions (Beaufort Coastal Plain, Kotzebue lowlands, Seward Peninsula) and the derived clade of Western Beringia (CH6 – Eastern limit, CH3 – Wrangel Island, CH4 – Amguema Transition, CH8 – Northeastern Koryak, CH7 – Lower Anadyr, CH5 – Kolyuchinki). The presence in the Bering Sea Islands is possibly due to dispersal into that region.
Noticeable are the climate differences in July across Beringia, e.g. for same latitudes (CH5 Kolyuchincki and CH6 Eastern limit vs. Kotzebue lowlands (P5) and Seward Peninsula (P4) (Figure 5A, B). Chukotka appears cooler and somewhat drier. Whether this presents another barrier for plants, climatic differences in addition to the physical barrier, remains to be tested. But the separations based on climate are already quite distinct and beyond 95% confidence intervals (Figure 5A, B).
Discussion
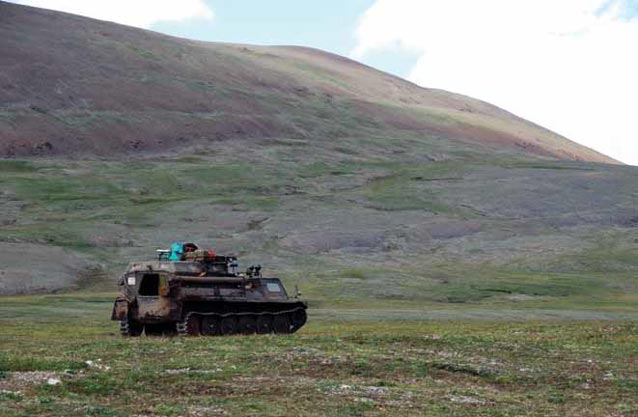
Photograph courtesy of Richard Bond
Measuring species diversity at fine scales through regional inventories and museum records is a first step in prioritizing targeted conservation management efforts. Moreover, a comparison of species assemblages between geographic regions can be used to understand the origins and history of biodiversity (Mavrodiev et al. 2012), when more detailed phylogenetic data are incomplete or lacking for a specific region.
The history of many taxa occurring in different ecoregions or areas cannot be explained by a single process of vicariance. Instead, many populations must be the result of dispersal into the area. Overall, dispersal across ecological niches is the most likely process for many endemic eastern and western Beringian disjunct distributions. In the absence of phylogenetic data, species occurrence records from herbarium specimens allowed us to generate testable hypotheses about the history of populations in a given area that can be explored using genetic data. Due to the poor comparability schemes for ecoregions within Beringia, we propose to assess and redesign them based on latest scientific data and methods.
Overall, when examining stochastic mapping reconstructions, current species distributions in Beringia have been independently shaped by dispersal, extinction, and in some cases vicariance events due to ecological or physical barriers (e.g., Bering Strait). In time, these processes have led to the independent formation of the same plant community in two disjunct areas (Western and Eastern Beringia).
As an arctic biodiversity hotspot, Wrangel Island is characterized by numerous endemic species (Petrovsky 1997). From our analysis it appears that the flora of Wrangel Island was formed mostly as the result of multiple dispersal events from the mainland; vicariance is also likely. Wrangel Island is part of the large continental shelf and was connected to mainland Beriniga until about 10,000 years ago. The island was not glaciated during at least the whole Pleistocene glaciation (Stauch and Gualtieri 2008) and was also not flooded during the late Pleistocene ocean transgressions (Bauch et al. 2001). It supports a great diversity of habitats, with numerous mountains reaching up to 1,096 m, covering over 70% of the surface of the island, and many valleys with favorable microclimate. This diversity of habitats on Wrangel Island may have contributed to the high level of endemism of the island. This increased diversity of endemic species is also reflected in an increased number of polyploids on the island and Petrovsky (1997) places it in its own floristic area “Wrangelia” (Petrovsky 1997, his Figure 1), distinct from the floristic areas of “Sewardia” that comprises most of the Chukotka Peninsula, St. Lawrence Island and the Seward Peninsula (comprising ecoregions CH5, CH6, P7 [St. Lawrence Island only], P4, P5, and P2, see Figure 1 for details) and “Mackenzia” (which extends from the Beaufort Sea [P9] to the Brooks Range and the Richardson mountains and from the Amundsen Bay in the east to the Gerald Shoal in the west).
The climate summaries for each ecoregion show some distinct patterns, differences and overlaps. Some have not been described for terrestrial parts of Beringia. For instance, we see that during July eastern Chukotka is on average 4 degrees cooler than western Alaska (Figure 5B), but while the precipitation is almost the same (there appears no relevant difference in winter climate though; results are therefore not shown here, see also Figure 5A). Young (1971) had already summarized similar findings in his often-overlooked article on the Floristic Zonation of the Arctic. When examining the northern limit of plant distribution in the Arctic, Young (1971) found that there is a close correlation between those limits and the total amount of summer warmth found in those areas. Using this information he constructed floristic zones for the Arctic that explain well, for instance, why the flora of St. Lawrence Island is so different from the Seward Peninsula despite their similar latitude and geographic proximity (Daniëls et al. 2000).
A detailed comparison of the Bering Sea islands with those of the Western Aleutian, albeit not arctic, provides another assessment of the patterns of floristic assemblage of two distinct oceanic island groups in Beringia (Garroutte and Ickert-Bond, this volume). To our knowledge, the terrestrial climate difference during the summer has not been described before when considering the ecological niche for plants within Beringia, other than the assessment made by Young (1971), with modification used by Elvebakk et al. (1999) and Daniëls et al. (2000). Likely, the influence of summer warmth on plant distribution in the Arctic are related to ocean regimes and currents and cloud cover (Ritchie and Hare 1971). These factors warrant more attention and study, and in regards to a ‘climate barrier’. Secondly, this finding has bigger implications for what specific regions actually can be compared within Beringia based on plant distributional data and beyond. The validity of the currently known ecoregions, and for Beringia overall requires adjustment and now that good plant distributional, genetic and climatic data are available, it allows us to more accurately describe ecoregions within Beringia.
Part of a series of articles titled Alaska Park Science - Volume 12 Issue 1: Science, History, and Alaska's Changing Landscapes.
Last updated: January 30, 2018