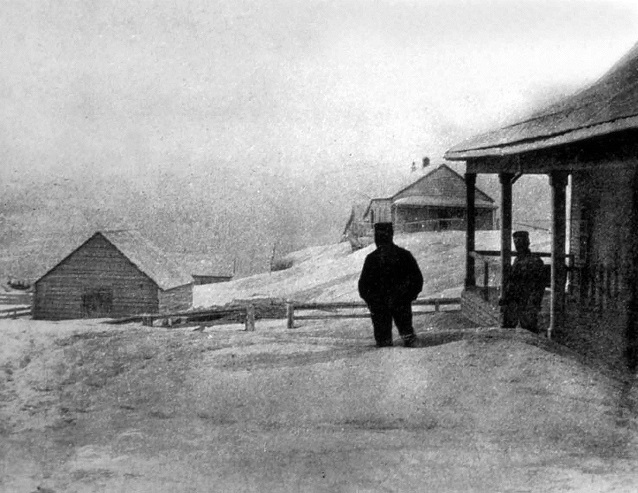
Photograph courtesy of W.J. Erskine, courtesy of National Geographic Society
A Novarupta-scale eruption is almost certain to happen again in Alaska; the question is when. Although the chance of another eruption of this magnitude occurring in any given year is small, such cataclysmic volcanic events have occurred repeatedly in Alaska. Picturesque Aniakchak caldera formed during a similar, though larger, catastrophic eruption 3,500 years ago (Neal et al. 2001). Within 500 miles of Anchorage, volcanologists have identified at least seven deposits of volcanic ash younger than 6,000 years that approach or exceed the volume of ash ejected by Novarupta in 1912, including a thick layer of ash erupted from Hayes Volcano, only 90 miles (150 km) northwest of Anchorage (Figure 2). Alaska was very sparsely populated in 1912, and there were few airplanes. Now, nearly three-quarters of a million people live in the state, and aircraft carrying many thousands of passengers and millions of dollars in cargo pass near Alaska’s more than 40 historically active volcanoes each day. The heavy ash fall produced by a Novarupta-scale eruption occurring today in southern Alaska would have economic and environmental impacts in Alaska and beyond.
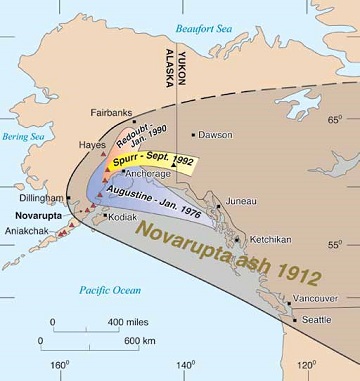
Volcano Hazards
A volcano hazard is any volcano-related process that potentially threatens life or property, with or without eruptive activity (Figure 3). Typically, several kinds of hazard will result from an eruption, the attendant risks depending upon the type and size of the eruption and location relative to the volcano. The most threatening hazards from the Katmai volcanoes include volcanic ash clouds and pyroclastic fallout, pyroclastic flows, lava domes and flows, floods and lahars, pumice rafts (large accumulations of coarse pumice floating in lakes or at sea), hydrothermal explosions, debris avalanches, volcanic gases, and phreatomagmatic eruptions through crater lakes or ice. Clearly, these dangers are initially destructive, but in many cases their lingering effects are also pronounced. Erosion and remobilization of pumice and ash deposits continue well after they are initially deposited. After the 1912 eruption, large amounts of pumice and ash were washed into creeks and rivers and quickly worked their way to the ocean. Re-vegetation can be slow on unstable, wind-blown pumice-covered surfaces, so the pumice continues to be washed into creeks for a long time. Great pumice rafts were reported still floating and clogging waterways in Shelikof Strait 22 years after the 1912 eruption (Hubbard 1935)! In any given area, the effects and extent of these volcano hazards will vary, depending on many factors, including (1) the size and duration of the eruption; (2) eruption type (for example, lava flow or explosive eruption), which can vary in time during an eruptive episode; (3) distance from the volcano; (4) proximity to any stream drainage that might become a pathway for any type of flow (lahar, flood, pyroclastic); (5) the amount of snow and ice that interacts with the eruption and eruptive products; and (6) wind speed and direction and general weather conditions.
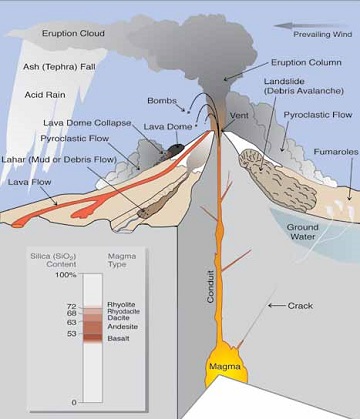
Event Frequency and Risk: Katmai Volcanoes as Examples
Explosive, ash-producing eruptions pose the greatest risk to life and property in the remote Alaska wilderness, with aircraft being most at risk (Casadevall 1994). Fortunately, although such large eruptions are devastating, they are also infrequent. More common are those eruptions like Southwest Trident (Figure 5) that erupted mainly lava flows intermittently for a couple of decades (1953-74). Eruptive histories of the Katmai cluster demonstrate the variety and scales of volcanic activity we might expect from the 80-odd volcanoes in the Alaska Peninsula and the Aleutian Chain. These eruptive histories show that, of the Katmai cluster of volcanoes, Mount Katmai and (to a lesser degree) Mount Mageik are most likely to erupt explosively, conceivably on the scale of the great 1912 outburst (Fierstein and Hildreth 2001). Such explosive eruptions would be less likely from Martin, Griggs, Trident, or Snowy, where lava flows, dome building, and small ash-plume episodes have been the norm. Although any volcanic ash plume ejected into the atmosphere is of concern for aircraft, the scale of an eruption significantly affects the extent of its associated hazards and areas at-risk. Such eruptive histories and subsequent hazard assessments have been done for nearly 20 active Alaska volcanoes by the Alaska Volcano Observatory (e.g., Neal et al. 2001, Coombs et al. 2008). Examining these volcanic histories helps geologists try to predict how each volcano might behave in the future.
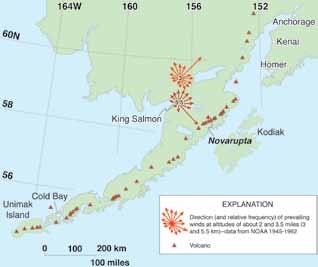
'Normal' Events
‘Normal’ eruptive events in the Katmai district, and in many places along the Alaska Peninsula and Aleutians, are characterized by the 1950s Trident eruptions, which sent several ash columns 6,600-20,000 ft (2-6 km) into the atmosphere, and a few 30,000-40,000 ft (9-12 km) high. Prevailing winds at elevations over 3.5 miles (5.5 km) would most commonly direct ash toward the southeast, whereas lower-elevation winds are most likely to direct ash toward the northeast (Figure 4). Although less common, winds do sometimes come from the Pacific, blowing north and northwestward. Such were conditions that directed most of the 1953 Trident ash (Ray 1967). Only rarely do winds in this area at any elevation blow westward, thus it is unlikely that significant amounts of ash (even from large eruptions) would be distributed much more than a hundred miles or so (a couple of hundred kilometers) west or southwest of the erupting volcano.
Size Matters
The size of the sector and extent of ash distribution depends mostly on the scale of an eruption; large eruptions affect much larger areas than small ones. Ash clouds from Southwest Trident would have severely affected aircraft overhead and in the immediate Katmai area. The larger (but still moderate-sized) eruptions of Redoubt (1990), Spurr (1992), and Augustine (1976 and 1986) affected considerably larger areas several hundred kilometers away (Figure 2). The 1912 eruption of Novarupta was exceptional; ash distribution dwarfed that of all the other historic Alaska eruptions combined. Although small to moderate-sized eruptions have been common throughout the eruptive histories of the Katmai volcanoes (no fewer than 15 such episodes in post-glacial time), there have been three ‘exceptional’ events from this cluster and a few moderately explosive pumice falls. Possibly the largest of all erupted ~23,000 years ago, another ~16,000 years ago (deposit is poorly preserved but eruption clearly was large), and the most recent was less than 100 years ago (Novarupta). Magma for all three originated beneath Mount Katmai.
Although exceptionally large events occur infrequently, the potential effects are far-reaching and severe. Now-populous Cook Inlet was severely blanketed with ash when Novarupta exploded (as the area also was 16,000 and 23,000 years ago). The Pacific slope of the Katmai volcanoes, too, was heavily mantled with pumice and ash (much of it remaining so today), and floating pumice rafts clogged Shelikof Strait and many of the bays along that coast. Ash was strongly wind-directed to the east-southeast in 1912, with the plight of inhabitants of Kodiak (Figure 1) making news headlines from San Francisco to Boston. Due to the sheer size of that eruption, significant amounts of ash fell even as far as 90 miles (150 km) upwind, to the northwest, covering what are today prime fishing and tourist areas.
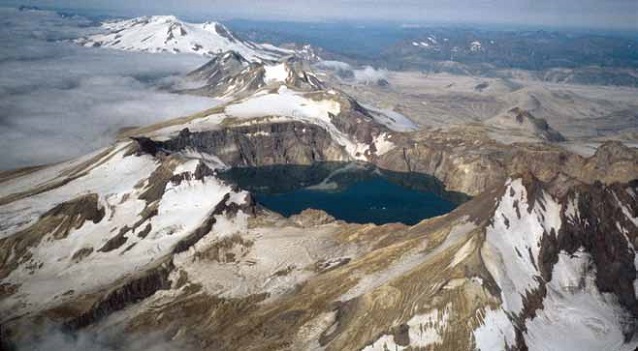
USGS photograph by J. Fierstein
Potential Impacts of Future Eruptions
A normal Trident-scale eruption (similar to the 1953-1974 episode) would affect mostly aircraft and backcountry hikers. Ash plumes, drifting ash clouds, and occasional dustings of ash on nearby airports in King Salmon and possibly Kodiak could disrupt local and regional air traffic for days to weeks at a time. Such disruption of supplies and access by air could occur sporadically for a decade or more. Impacts of a prolonged eruption may not be entirely negative; small aircraft and local guide services might be anticipated to even take the opportunity to make such an eruption a tourist attraction! Backcountry hikers, too, could be drawn to such a phenomenon. Such intrepid adventurers would be at risk for unexpected explosions, lahars, debris avalanches, and ballistic bomb showers that accompany such eruptions.
During and after an exceptional 1912-scale eruption, we might expect a range of problems that would have severe and long-lasting economic effects for Alaska. Significant ash fall could damage or significantly disrupt operation of infrastructure (airports, electrical, water, and other critical systems) in areas of southern Alaska (Wilson et al. 2011). Chronic exposure to ash can adversely affect all types of motors from chainsaws to automobiles and affect cooling and ventilation systems reliant on external air (for instance: hospitals, computer systems, and bankcard machines). Ash fall would also contaminate water supplies and would be detrimental to fishing, wildlife, and tourism for years thereafter, as well as create eye and respiratory problems for many residents and animals. Clean-up may take days or weeks following small eruptions but such efforts could extend for months after a large eruption like that of 1912. Even after initial clean-up, within 100-200 miles of the volcano, normal Alaskan windstorms would remobilize loose dust from ash-covered slopes and valleys, lofting it high into the atmosphere. Such remobilization of ash can continue for decades; remobilizing of 1912 ash continues today (Fierstein and Hildreth 2001).
Early Warning
Volcanic eruptions cannot be prevented, but monitoring volcanoes can provide early warning of pending activity. The Alaska Volcano Observatory (AVO), a cooperative program of the U.S. Geological Survey, University of Alaska, and the Alaska State Division of Geological and Geophysical Surveys, operates a network of seismometers that detects earthquakes around the Katmai cluster of volcanoes as well as on and near many other volcanoes from Cook Inlet to the Aleutians. Volcanic earthquakes, caused by movement of magma or hydrothermal fluids beneath an edifice, are used to monitor the activity level of volcanoes, since most eruptions are typically preceded for hours to months by increased seismicity.
Other tools employed by AVO to monitor volcanic unrest include: daily satellite observation to look for elevated surface temperatures or the presence of ash in the atmosphere, airborne and ground-based volcanic gas measurements, lake and spring water chemistry, and surveys of ground deformation. Pilots and hikers also commonly provide AVO with observations that are used to direct further investigations of reported phenomena. Sightings of new steaming ground, increased fumarolic activity, and possible ash plumes all prompt further investigation by AVO personnel.
Early warning signs of volcanic unrest are evaluated in light of what is known about the volcano and its eruptive history. Thus, familiarity with a volcano’s eruptive past can inform interpretations of data collected from monitoring instruments.
Alaska Volcanoes: Scenic Wildernesses and Natural Laboratories
Alaska volcanoes make for some of the most spectacular landscapes on earth. Adventurers, sightseers, artists and photographers are awed by this scenery and its wildness. Scientific researchers, too, are moved by the forces that have shaped these landscapes. For more than a century, Alaska volcanoes, including those preserved in national parks, have been host to cutting-edge scientific research that has shaped our thinking about how volcanoes work. Seismic data reveal the depth at which magma is stored beneath volcanoes and how quickly it rises toward the surface. Satellite images show ground deformation and ash plumes in remote areas. Geologic mapping and studies of a volcano’s eruptive products provide a framework in which to interpret new volcanic activity. Examination of plant, animal and human recovery from past catastrophic eruptions gives an insight as to how modern life might be affected by a similar event. Ongoing studies continue to improve our understanding of volcanoes, magmatism, and how those interact with our society today. There is room now more than ever for enthusiastic new researchers, and Alaska’s national parks provide a beautiful and dynamic natural laboratory.
Learn more about volcanic ash hazards.
References
Casadevall, T.J., (ed). 1994. Volcanic ash and aviation safety. Proceedings of the First International Symposium on Volcanic Ash and Aviation Safety. U.S. Geological Survey Bulletin 2047.
Coombs, M.L., R.G. McGimsey, and B.L. Brown. 2008. Preliminary volcano-hazard assessment for Gareloi Volcano, Garreloi Island, Alaska. U.S. Geological Survey Scientific Investigations Report 2008-5159.
Fierstein, J., and W. Hildreth. 2001. Preliminary volcano-hazard assessment for the Katmai Volcanic Cluster, Alaska. U.S. Geological Survey Open-File Report 00-489.
Hubbard, B.R. 1935. Cradle of the Storms. Dodd, Mead & Co. New York.
Meyers, B., S.R. Brantley, P. Stauffer, and J.W. Hendley. 1997. What are Volcano Hazards? U.S. Geological Survey Fact Sheet-002-97.
Neal, C.A., R.G. McGimsey, T.P. Miller, J.R. Riehle, and C.F. Waythomas. 2001. Preliminary volcano-hazards assessment for Aniakchak Volcano, Alaska. U.S. Geological Survey Open-File
Report 00-519.
Ray, D.K. 1967. Geochemistry and Petrology of the Mt. Trident Andesites, Katmai National Monument, Alaska. Ph.D. Dissertation. University of Alaska Fairbanks.
Wilson, T.M., C. Stewart, V. Sword-Daniels, G.S. Leonard, D.M. Johnston, J.W. Cole, J. Wardman, G. Wilson, and S.T. Barnard. 2011. Volcanic ash impacts on critical infrastructure. Journal of Physics and Chemistry of the Earth. DOI: 10.1016/j. pce.2011.06.006.
Part of a series of articles titled Alaska Park Science - Volume 11 Issue 1: Volcanoes of Katmai and the Alaska Peninsula.
Last updated: July 25, 2016