Last updated: October 26, 2021
Article
The Burning Tundra: A Look Back at the Last 6,000 Years of Fire in the Noatak National Preserve, Northwestern Alaska
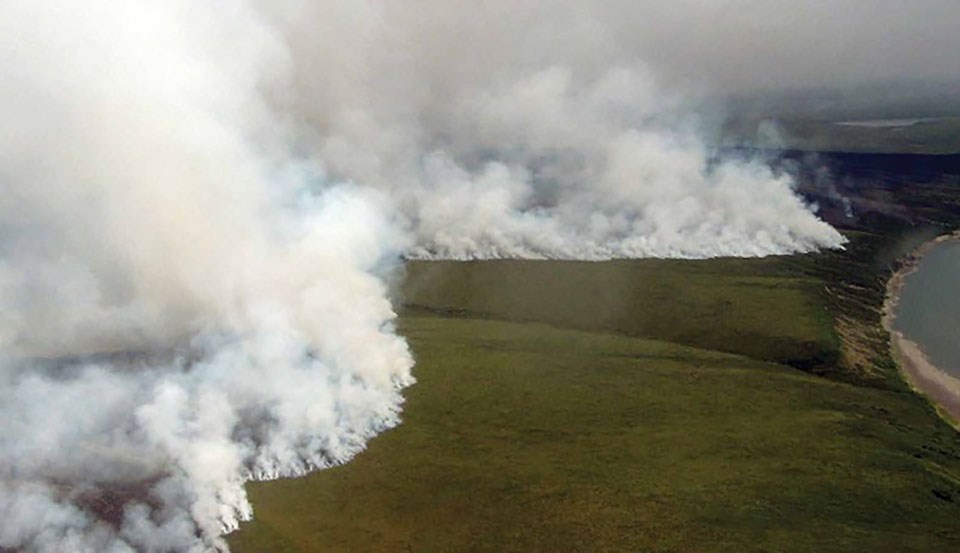
National Park Service photograph
By Philip E. Higuera, Jennifer L. Barnes, Melissa L. Chipman, Michael Urban, and Feng Sheng Hu
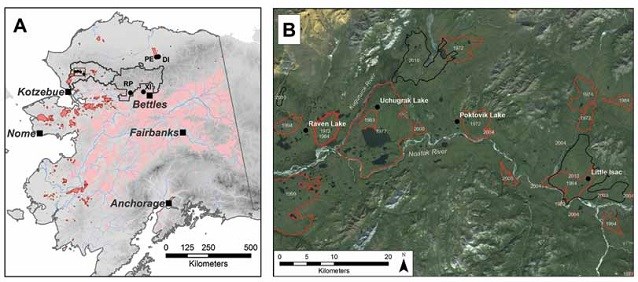
Introduction
More than 5.4 million acres (2.2 million hectares) of Alaska tundra have burned over the past 60 years (Figure 2), indicating its flammable nature under warm, dry weather conditions. Tundra fires have important impacts on vegetation composition (Racine et al. 1987, 2004), permafrost dynamics, nutrient and carbon cycling (Wookey et al. 2009), and wildlife populations (Jandt et al. 2008, Joly et al. 2010). Despite the impacts of tundra burning, relatively little is known about natural variability in fire occurrence and links to climate and vegetation change. This lack of knowledge hinders land-management and resource-planning efforts.Increasing evidence suggests that Arctic environmental change is affecting tundra fire regimes. In 2010 for example, 37 fires burned more than 106,696 acres (43,180 ha) in Noatak National Preserve (Figures 1, 2b), the largest number of fires occurring in this area since record keeping began in 1950. Three years prior, the Anaktuvuk River Fire on Alaska’s North Slope more than doubled the total area burned north of 68° N in Alaska since 1950 (Figure 2) (Hu et al. 2010, Jones et al. 2009).
Figure 2. Regional and local location of study sites. (A) Alaska fire history (red polygons) from 1950-2009, with tundra fires highlighted in dark red. Other tundra fire-history records from lake sediments are identified by black dots: Ruppert (RP) and Xindi (XI) lakes, in the south-central Brooks Range, and Perch (PE) and Dimple (DI) lakes, within the 2007 Anaktuvuk River Fire. (B) The Noatak study area: large fire perimeters from 1950-2009 are shown in red, fires that burned in 2010 are outlined in black, and the location of four lakes cored in 2007 are identified by black dots.
This event, associated with record-high temperatures and record-low precipitation and sea-ice extent, marked the first time this area burned in at least 5,000 years (Hu et al. 2010). Changes in fuel characteristics associated with increased shrub density over the past several decades (e.g., Tape et al. 2006) may also be changing tundra flammability. Paleoecological evidence from ‘ancient’ shrub tundra in and around Gates of the Arctic National Park and Preserve (Figure 2) indicates the high flammability of birch shrubs that dominated this region circa 13,000-11,000 calendar years before present (cal. yr BP) (Higuera et al. 2008). In combination with studies documenting increased birch abundances in recently-burned areas (e.g., Joly et al. 2010), these patterns raise the possibility of positive feedbacks between increased shrub density and increased tundra burning.
We investigated fire history of the past 6,000 years in the Noatak National Preserve, with the goal of understanding how fire regimes varied in relation to climate and vegetation. This work places modern tundra fire occurrence in the context of natural variability, provides critical fire-history information for fire managers, and elucidates drivers of tundra fire regimes with relevance to past, present and future tundra ecosystems.
We investigated fire history of the past 6,000 years in the Noatak National Preserve, with the goal of understanding how fire regimes varied in relation to climate and vegetation. This work places modern tundra fire occurrence in the context of natural variability, provides critical fire-history information for fire managers, and elucidates drivers of tundra fire regimes with relevance to past, present and future tundra ecosystems.
Methods
Our study capitalized on the ability to reconstruct both vegetation and fire history using lake-sediment records. Pollen grains dispersed from plants and charcoal pieces dispersed from fires are well preserved in the sediments at the bottom of lakes, where a lack of oxygen prevents decomposition. As sediments accumulate over time, so too does a record of vegetation and fire history. By collecting and analyzing individual layers of lake sediments, we reconstructed vegetation and fire regimes spanning the past 6,000 years.We collected lake-sediment cores from four lakes along an east–west transect in Noatak National Preserve (Figure 2b). These lakes span a modern gradient in climate and vegetation, with down-valley sites characterized by slightly warmer, drier summers and a greater abundance of tussock-shrub tundra and white spruce. Each lake-sediment core was sliced at 0.10-0.20 inch (0.25-0.50 cm) intervals, representing 15 years on average, and geochemical techniques (carbon 14 and lead 210 dating) were used to establish the timing of past events.
To estimate vegetation composition within approximately 0.6-3.1 miles (1-5 km) of each lake, we counted pollen grains in sediment samples at circa 250 to 500 year intervals. To reconstruct when fires burned within approximately 0.6 mi (1 km) of each lake, we counted macroscopic charcoal pieces (> 0.0071 in/180 µm diameter) in each sediment layer, and used statistical methods to identify fire events in the record (as described by Higuera et al. 2009). Estimated fire events were used to calculate fire-event return intervals (FRIs ,years between fire events), providing a conservative estimate of site-specific fire return intervals.
Results and Discussion
Charcoal records from the study area provide unambiguous evidence of burning over the past 6,000 years, with individual FRIs ranging from 30 to 840 years (Figures 3-6). This variability reflects differences across space and changes in climate and vegetation through time.Estimated fire-return intervals for the ‘recent’ past
Our best estimates of recent FRIs come from the charcoal records of the past 2,000 years, a period long enough to capture multiple fire events for a statistical assessment. Median FRIs varied among sites, from 113 years (95% confidence interval [CI] 75-150) at Uchugrak Lake to 240 years (CI 105-548) at Little Isac Lake (Figure 3). When pooled, the two down-valley sites of Raven and Uchugrak had shorter FRIs (median 150 yr, CI 101-150) than those from the two up-valley sites of Poktovik and Little Isac (median 218 yr, CI 128-285) (Figure 3).Our charcoal-based mean FRIs across the study area are shorter than previous estimates for two portions of the Noatak River watershed, based on circa 30-60 years of observational data. Down-valley sites had estimated fire cycles (equivalent to the point-specific mean FRI) of 175-193 years (Kobuk Ridges and Valley Ecoregion) (Joly et al. 2009, Kasischke et al. 2002), and up-valley sites had estimated fire cycles of 480 years (Gabriel and Tande 1983). These differences likely reflect the difficulty in estimating fire cycles greater than 150 years with a < 60-yr dataset. They may also represent real but yet undetectable changes in tundra fire occurrence and/or an overestimation of local fires in the charcoal records.
Differences in burning rates between down-and up-valley sites correspond to spatial variability in climate and vegetation. Lower growing season temperature and increased precipitation at up-valley sites limited fuel drying and thus the probability of fire ignition and spread, relative to down-valley sites. The importance of climate in controlling tundra fires is also apparent in Alaska over the last 60 years. Tundra fire regimes are most active in regions with warm and/or dry seasonal climate (Higuera et al. 2008), and tundra fires occur during years with above average temperatures and below average moisture (Hu et al. 2010; Jones et al. 2009). Interestingly, the site with the highest median FRI in the past 2,000 years (i.e., Little Isac Lake) burned in the summer of 2010, making it the second fire at this site in 26 years (Figure 2). Return intervals nearly this short (i.e., 30 yr) also occurred at this site in the past (Figures 3, 5).
Vegetation and Fire History Over the Past 5,000-6,000 Years
Over the past 6,000 years, vegetation and fire regimes fluctuated in the Noatak study area in response to millennial-scale changes in effective moisture (Clegg and Hu 2010) (Figures 5-7). An increase in Picea (spruce) pollen in all records indicates the regional expansion of white spruce circa 2000-3000 cal. yr BP, and at most sites Alnus (alder) pollen percentages gradually decreased since 4000-6000 cal. yr BP. Coincident with these vegetation changes, long-term mean FRIs also varied over time (Figure 5). Most sites experienced less frequent burning (i.e., longer FRIs) prior to 2000 cal. yr BP, when alder pollen percentages were higher and spruce pollen percentages were lower. The exception was at Little Isac Lake, where alder pollen percentages increased over time, and Betula (birch) pollen percentages in the past were higher than at other sites. Likewise, fires were more frequent prior to 2000 cal. yr BP at Little Isac Lake, despite the opposite pattern at other sites.The variability in millennial-scale mean FRIs was significantly correlated with changes in pollen percent-ages of select taxa (0.35 < |r| < 0.74) (Figure 6). Alder was most consistently correlated with fire frequencies, with an increase in alder associated with a decrease in fire frequency. Correlations with spruce, grasses, sedges and birch were more variable, but in general these taxa were positively related to increased fire frequencies.
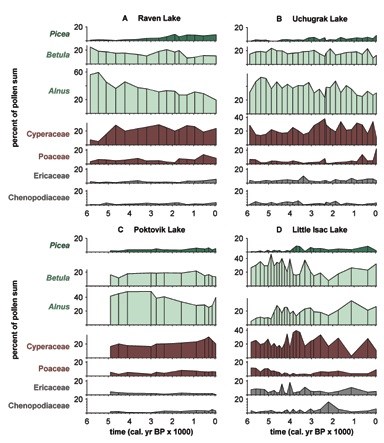
Conclusions
This study provides estimated fire return intervals (FRIs) for one of the most flammable tundra ecosystems in Alaska. Fire managers require this basic information, and it provides a valuable context for ongoing and future environmental change. At most sites, FRIs varied through time in response to changes in climate and local vegetation. Thus, an individual mean or median FRI does not capture the range of variability in tundra fire occurrence. Long-term mean FRIs in many periods were both shorter than estimates based on the past60 years and statistically indistinct from mean FRIs found in Alaskan boreal forests (e.g., Higuera et al. 2009) (Figure 2). These results imply that tundra ecosystems have been resilient to relatively frequent burning over the past 6,000 years, which has implications for both managers and scientists concerned about environmental change in tundra ecosystems. For example, increased tundra fire occurrence could negatively impact winter forage for the Western Arctic Caribou Herd (Joly et al. 2009). Although the Noatak is only a portion of this herd’s range, our results indicate that if caribou utilized the study area over the past 6,000 years, then they have successfully co-existed with relatively frequent fire.
Fire history in the Noatak also suggests that subtle changes in vegetation were linked to changes in tundra fire occurrence. Spatial variability across the study region suggests that vegetation responded to local-scale climate, which in turn influenced the flammability of surrounding areas. This work adds to evidence from ‘ancient’ shrub tundra in the southcentral Brooks Range suggesting that vegetation change will likely modify tundra fire regimes, and it further suggests that the direction of this impact will depend upon the specific makeup of future tundra vegetation. Ongoing climate-related vegetation change in arctic tundra such as increasing shrub abundance in response to warming temperatures (e.g., Tape et al. 2006), could both increase (e.g., birch) or decrease
(e.g., alder) the probability of future tundra fires.
Acknowledgements
We thank numerous National Park Service personnel from the Western Arctic Parklands and Denali Fire Crew for logistical support and field assistance, Triet Vuong and Jennifer Beamer for lab assistance, and Paul Dunnette for earlier comments on the manuscript. This work was funded by the Joint Fire Science Program through project number 06-3-1-23 (FSH, JLB) and a National Park Ecological Research Fellowship (PEH).
REFERENCES
Clegg, B.F., and F.S. Hu. 2010.
An oxygen-isotope record of Holocene climate change in the south-central Brooks Range, Alaska. Quaternary Science Reviews 29: 928-939.
Gabriel, H.W., and G.F. Tande. 1983.
A regional approach to fire history in Alaska. BLM-Alaska Technical Report 9. BLM/AK/TR-83-09.
Higuera, P.E., L.B. Brubaker, P.M. Anderson, T.A. Brown, A.T. Kennedy, and F.S. Hu. 2008.
Frequent Fires in Ancient Shrub Tundra: Implications of Paleorecords for Arctic Environmental Change. PLoS ONE 3:e0001744.
Higuera, P.E., L.B. Brubaker, P.M. Anderson, F.S. Hu, and T.A. Brown. 2009.
Vegetation mediated the impacts of postglacial climate change on fire regimes in the south-central Brooks Range, Alaska. Ecological Monographs 79 (2): 201-219.
Hu, F.S., P.E. Higuera, J.E. Walsh, W.L. Chapman, P.A. Duffy, L.B. Brubaker, and M.L. Chipman. 2010.
Tundra Burning in Alaska: Linkages to Climatic Change and Sea-Ice Retreat. Journal of Geophysical Research-Biogeosciences 115:G04002.
Jandt, R., K. Joly, C.R. Meyers, and C. Racine. 2008.
Slow recovery of lichen on burned caribou winter range in Alaska tundra: Potential influences of climate warming and other disturbance factors. Arctic, Antarctic, and Alpine Research 40 (1): 89-95.
Joly, K., F.S. Chapin, and D.R. Klein. 2010.
Winter habitat selection by caribou in relation to lichen abundance, wildfires, grazing, and landscape characteristics in northwest Alaska. Ecoscience 17 (3): 321-333.
Joly, K., T.S. Rupp, R.R. Jandt, and F.S. Chapin. 2009.
Fire in the range of the Western Arctic Caribou Herd. Alaska Park Science 8 (2): 85-91.
Jones, B.M., C.A. Kolden, R. Jandt, J.T. Abatzoglou, F. Urban, and C.D. Arp. 2009.
Fire Behavior, Weather, and Burn Severity of the 2007 Anaktuvuk River Tundra Fire, North Slope, Alaska. Arctic, Antarctic, and Alpine Research 41: 309-316.
Kasischke, E.S., D. Williams, and D. Barry. 2002.
Analysis of the patterns of large fires in the boreal forest region of Alaska. International Journal of Wildland Fire 11: 131-144.
Racine, C., R. Jandt, C. Meyers, and J. Dennis. 2004.
Tundra fire and vegetation change along a hillslope on the Seward Peninsula, Alaska, U.S.A. Arctic, Antarctic, and Alpine Research 36 (1): 1-10.
Racine, C.H., L.A. Johnson, and L.A. Viereck. 1987.
Patterns of vegetation recovery after tundra fires in northwestern Alaska, U.S.A. Arctic and Alpine Research 19 (4): 461-469.
Tape, K., M. Sturm, and C. Racine. 2006.
The evidence for shrub expansion in Northern Alaska and the Pan-Arctic. Global Change Biology 12 (4): 686-702.
Wookey, P.A., R. Aerts, R.D. Bardgett, F. Baptist, K.A. Brathen, J.H.C. Cornelissen, L. Gough, I.P. Hartley, D.W. Hopkins, S. Lavorel, and G.R. Shaver. 2009.
Ecosystem feedbacks and cascade processes: understanding their role in the response of Arctic and alpine ecosystems to environmental change. Global Change Biology 15: 1153-1172.
Clegg, B.F., and F.S. Hu. 2010.
An oxygen-isotope record of Holocene climate change in the south-central Brooks Range, Alaska. Quaternary Science Reviews 29: 928-939.
Gabriel, H.W., and G.F. Tande. 1983.
A regional approach to fire history in Alaska. BLM-Alaska Technical Report 9. BLM/AK/TR-83-09.
Higuera, P.E., L.B. Brubaker, P.M. Anderson, T.A. Brown, A.T. Kennedy, and F.S. Hu. 2008.
Frequent Fires in Ancient Shrub Tundra: Implications of Paleorecords for Arctic Environmental Change. PLoS ONE 3:e0001744.
Higuera, P.E., L.B. Brubaker, P.M. Anderson, F.S. Hu, and T.A. Brown. 2009.
Vegetation mediated the impacts of postglacial climate change on fire regimes in the south-central Brooks Range, Alaska. Ecological Monographs 79 (2): 201-219.
Hu, F.S., P.E. Higuera, J.E. Walsh, W.L. Chapman, P.A. Duffy, L.B. Brubaker, and M.L. Chipman. 2010.
Tundra Burning in Alaska: Linkages to Climatic Change and Sea-Ice Retreat. Journal of Geophysical Research-Biogeosciences 115:G04002.
Jandt, R., K. Joly, C.R. Meyers, and C. Racine. 2008.
Slow recovery of lichen on burned caribou winter range in Alaska tundra: Potential influences of climate warming and other disturbance factors. Arctic, Antarctic, and Alpine Research 40 (1): 89-95.
Joly, K., F.S. Chapin, and D.R. Klein. 2010.
Winter habitat selection by caribou in relation to lichen abundance, wildfires, grazing, and landscape characteristics in northwest Alaska. Ecoscience 17 (3): 321-333.
Joly, K., T.S. Rupp, R.R. Jandt, and F.S. Chapin. 2009.
Fire in the range of the Western Arctic Caribou Herd. Alaska Park Science 8 (2): 85-91.
Jones, B.M., C.A. Kolden, R. Jandt, J.T. Abatzoglou, F. Urban, and C.D. Arp. 2009.
Fire Behavior, Weather, and Burn Severity of the 2007 Anaktuvuk River Tundra Fire, North Slope, Alaska. Arctic, Antarctic, and Alpine Research 41: 309-316.
Kasischke, E.S., D. Williams, and D. Barry. 2002.
Analysis of the patterns of large fires in the boreal forest region of Alaska. International Journal of Wildland Fire 11: 131-144.
Racine, C., R. Jandt, C. Meyers, and J. Dennis. 2004.
Tundra fire and vegetation change along a hillslope on the Seward Peninsula, Alaska, U.S.A. Arctic, Antarctic, and Alpine Research 36 (1): 1-10.
Racine, C.H., L.A. Johnson, and L.A. Viereck. 1987.
Patterns of vegetation recovery after tundra fires in northwestern Alaska, U.S.A. Arctic and Alpine Research 19 (4): 461-469.
Tape, K., M. Sturm, and C. Racine. 2006.
The evidence for shrub expansion in Northern Alaska and the Pan-Arctic. Global Change Biology 12 (4): 686-702.
Wookey, P.A., R. Aerts, R.D. Bardgett, F. Baptist, K.A. Brathen, J.H.C. Cornelissen, L. Gough, I.P. Hartley, D.W. Hopkins, S. Lavorel, and G.R. Shaver. 2009.
Ecosystem feedbacks and cascade processes: understanding their role in the response of Arctic and alpine ecosystems to environmental change. Global Change Biology 15: 1153-1172.