Part of a series of articles titled Alaska Park Science - Volume 10 Issue 1: Connections to Natural and Cultural Resource Studies in Alaska's National Parks.
Article
Understanding Moraine Formation Around Denali's Muldrow Glacier
By Jason M. Dortch, Lewis A. Owen, Marc W. Caffee, Phil Brease
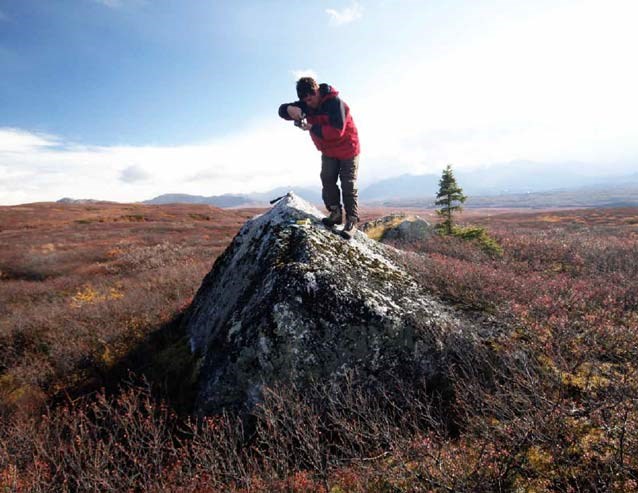
Courtesy of Dr. Jason Dortch
Glaciers are sensitive markers of current and past climatic conditions, mainly temperature and precipitation. Changes in temperature and precipitation alter the size and position of a glacier. Cooler temperatures combined with increased snowfall will cause a glacier to advance, whereas warmer temperatures and/or decreased snowfall will cause a glacier to thin and retreat.
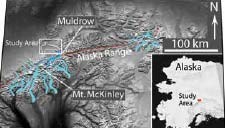
Image courtesy of IKONOS
Historically, radiocarbon dating and lichenonmetry (measurement of certain lichens that increase in size at a constant rate every year) were used to determine the ages of glacial landforms in the McKinley River area (Bijkerk 1980, Werner 1982). These techniques can only be used to date organic material younger than ~50,000 years. Since much of the glacial debris and landforms are devoid of organic material and may be older than 50,000 years old, these techniques will not work. A relatively new method, cosmogenic radionuclide dating, can be used to obtain ages on glacial debris and landforms from 100 to over 1 million years old. The secondary neutron cascade produced by galactic cosmic rays (mostly hydrogen atoms stripped of their electrons) penetrates Earth’s atmosphere and bombards the uppermost rock surfaces causing nuclear reactions in the atoms comprising the rocks. Beryllium-10 (10Be), in particular, is produced from oxygen and silica in the rock. The 10Be production rates are known. By determining the amount of this radionuclide in a sample, we can calculate how long that rock has been sitting on the surface of Earth.
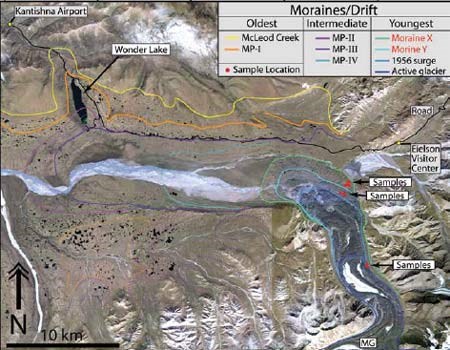
Image courtesy of IKONOS
Many of problems associated with 10Be dating have been addressed in numerous studies (Balco et al. 2009, Gosse and Phillips 2001, Hallet and Putkonen 1994, Owen et al. 2008, Putkonen and Swanson 2003, Putkonen and O’Neil 2005); however, the time lag between deposition and stabilization of moraines has received little attention (Briner et al. 2005). Quantifying the time lag between deposition and stabilization is essential for understanding and comparing recent fluctuations in glaciation that are driven by climate change.
Purpose and Methods
The primary issue we are exploring on the northern side of the Alaska Range is the lag time between deposition and stabilization of moraines. A key clue is whether unstable moraines have near zero 10Be ages. To assess these two issues we refer to two test moraines, referred to here as moraine X and moraine Y.
The deposition age of moraine X has already been defined through lichenometry, so we can quantify the time lag between moraine X’s deposition and stabilization using 10Be dating. The time between moraine stabilization and moraine deposition is determined by subtracting the 10Be age from the lichen age.
The second issue is explored in moraine Y. Werner (1982) argued that moraine Y is unstable and the active ice is by definition “unstable”. If our hypothesis is correct, then unstable landforms should yield a zero 10Be age.
Data gathering and research involved remapping the extent of moraines previously mapped by Werner (1982) in the McKinley River area using field methods, aerial photography, and IKONOS satellite imagery, provided by Denali National Park and Preserve. Samples for 10Be dating were collected by chiseling small amounts of rock from the upper 1 to 2 inches (2.5-5 cm) of large granitic boulders on moraines. Multiple samples on each landform enabled statistical analysis of age populations as well as further examination of landform stabilization processes.
The rock samples were subjected to a series of chemical leaches, dissolution, and chemical separations to isolate the Be atoms. Measurements of the separated 10Be atoms were obtained using an accelerated mass spectrometer at the Purdue Rare Isotope Measurement Laboratory at Purdue University. The number of measured 10Be atoms is divided by the rate at which they accumulate in rock surfaces to yield an exposure age. The exposure age tells us how long the boulder’s surfaces have been exposed to cosmic rays on Earth’s surface.
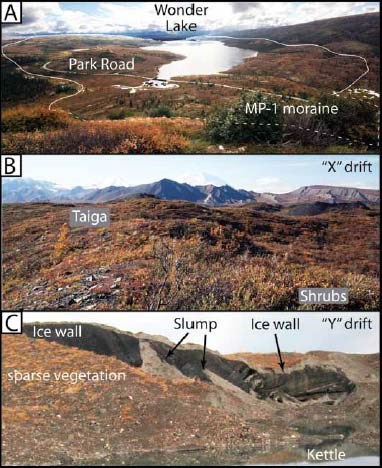
Photographs courtesy of Dr. Jason Dortch
Moraine Ages
Moraine X
Moraine X is about 1 mile (1.6 km) wide, and is stable with no evidence for active slumping or exposed ice walls (Werner 1982) (Figures 3, 4b). Seven samples were collected from six boulders for 10Be dating.Statistical analysis of 10Be ages revealed a strong grouping of 10Be ages at 540 years. This age is interpreted to represent the stabilization of moraine X. The difference between the lichen age (>1,800±100 years) (Werner 1982) which represents moraine deposition and the 10Be age which represents moraine stabilization (540 years) is the time lag between moraine deposition and stabilization (1,260 years).
While the lag time is not significant in relating glacial sediment that are >100,000 years old, it could have adverse affects on comparison of young moraines (< 10,000 years old), and between areas using mixed chronology techniques, such as radiocarbon, lichenometry, and dendrochronology. This is particularly important for studies focused on recent climate change. For example, if several moraines were determined to be 2,700 years old, some dated using lichenometry and others using 10Be, then comparing the extent of glaciation based on moraine ages would be inappropriate. The moraines dated using 10Be may in fact be 1,300 years older (i.e. were actually deposited 4,000 years ago but stabilized 2,700 years ago), which would alter estimates of glacial retreat and provide an incorrect estimation of the 2,700 year old Neoglacial extent to climate modelers.
Moraine Y
Moraine Y ranges from 0.25 to 1.25 miles (0.4-2.0 km) wide (Figure 2). Werner (1982) argued that moraine Y is unstable based on the presence of active slumping, streams originating from outcrops, and several outcrops of glacial ice (Figure 4c). We concur with this view. Four samples were collected from four boulders for 10Be dating.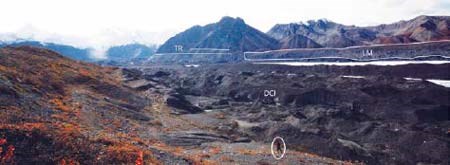
Photograph courtesy of Dr. Jason Dortch
The 10Be ages did not pass statistical analysis. This was expected because moraine Y is unstable; the 10Be ages should be close to zero and not cluster together. The 10Be ages provide insight into the stabilization process. The age of moraine Y was estimated at 900 years using lichenometry (Werner 1982). Three boulders have a zero 10Be age, which confirms our hypothesis that boulders very likely move as the ice core melts out and the moraine stabilizes.
One 10Be age was almost twice as old as the lichen age, which tells us that prior 10Be accumulation occurred when the boulder surface was still part of Mt. McKinley or when the boulder was carried on top of Muldrow Glacier. This boulder will likely be broken and rolled during the stabilization period, which will eventually reset the 10Be age to zero.
Active Ice
The active Muldrow Glacier is mainly covered by thick debris on its surface (supraglacial debris). There is also bare glacial ice, exposed ice walls, and small kettle lakes. Samples collected from boulders on active ice should have a zero 10Be concentration because they have only been recently exposed. Four boulders were sampled for 10Be dating from the active glacier to check for prior 10Be accumulation.
Three of the 10Be ages on the active Muldrow Glacier are within error of a zero age, which confirms our second hypothesis that boulders will very likely move again as the ice core melts out and the moraine stabilizes. One sample, with an age of 1,900 years, has significant prior 10Be accumulation. This one boulder will likely be broken and rolled during the stabilization period, which will eventually reset the 10Be age to zero. As with moraine Y, the importance of 10Be accumulation before moraine stabilization appears to be minimal.
Conclusions
Only two 10Be ages, one each from moraine Y and the active ice, have prior 10Be accumulation on boulder surfaces. This is likely due to the continued toppling, exhumation, and break up boulders experience during glacier transport and moraine stabilization. This shows that 10Be ages are reset to “zero” until a moraine stabilizes, which confirms our second hypothesis that 10Be dating records a moraine’s stabilization age.
Moraine X has an average 10Be age of 540 years old. Using the lichen age (1,800 years old) shows that the lag time between landform deposition and stabilization is 1,260 years. Therefore, we argue that moraines with an ice core can take approximately 1,300 years to stabilize after initial deposition, although this might vary in different climatic settings. Stabilization times need to be quantified in different climatic regimes to build a model relating landform stability and 10Be ages and determine if the lag time is consistent in both upper and lower latitude areas.
This study provides a preliminary framework for determining stabilization lag time. This data will help enable a clear understanding and more accurate comparison of 10Be ages (less than 10,000 years old) with other dating methods. Our results suggest that correlation of glacial deposits less than 10,000 years old dated with different methods will need to be reevaluated. Incorrect correlation of glacial deposits will have a significant effect on climate models, as the models need to compare changes in the position and size of glaciers over large areas that occurred at the same time. If the correlations are incorrect, the models will yield inaccurate results. Future work focused on refining the lag time between moraine deposition and stabilization will enable more accurate ages and climate driven changes in glaciation to be more narrowly defined and better understood.
The results of our studies have been published in Dortch et al. (2010a and 2010b).
We would like to thank the Murie Learning and Science Center for funding this project. Sadly, our co-author, Phil Brease, passed away on May 12, 2010. He was a kind person, interested in the processes that shape our planet. He will be greatly missed by all.
References
Balco, G., J. Briner, R.C. Finkel, J. Rayburn, J.C. Ridge, and J.M. Schaefer. 2009.
Regional beryllium-10 production rate calibration for late-glacial northeastern North America. Quaternary Geochronology 4: 93-107.
Bijkerk, A. 1980.
Lichenometry dating of Neoglacial deposits in the McKinley Park area, Alaska. Masters thesis. Grand Valley State Colleges.
Briner, J.P., D.S. Kaufman, W.F. Manley, R.C. Finkel, and M.W. Caffee. 2005.
Cosmogenic exposure dating of late Pleistocene moraine stabilization in Alaska. Geologic Society of America Bulletin 117: 1108-1120.
Dortch, J., L.A. Owen, M.W. Caffee, and P. Brease. 2010a.
Late Quaternary glaciation and equilibrium line altitude variations of the McKinley River region, central Alaska Range. Boreas 39: 233-246.
Dortch, J.M., L.A. Owen, M.W. Caffee, L. Dewen, and T. Lowell. 2010b.
Beryllium-10 surface exposure dating of glacial successions in the Central Alaska Range. Journal of Quaternary Science 25: 1259-1269 (DOI: 10.1002/jqs.1406).
Gosse, J.C., and F.M. Phillips. 2001.
Terrestrial in situ cosmogenic nuclides: Theory and application. Quaternary Science Reviews 20: 1475-1560.
Hallet, B., and J. Putkonen. 1994.
Surface dating of dynamic landforms: young boulders on aging moraines. Science 265: 937-940.
IPCC (Intergovernmental Panel on Climate Change). 2007.
Working Group II: Impacts, Adaptation and Vulnerability. 653-686. (http://www.ipcc-wg2.org/)
Owen, L.A., M.W. Caffee, R.C. Finkel, and Y.B. Seong. 2008.
Quaternary glaciation of the Himalayan-Tibetan orogen. Journal of Quaternary Research 23: 513-531.
Putkonen, J., and T. Swanson. 2003.
Accuracy of cosmogenic ages for moraines. Quaternary Research 59: 255-261.
Putkonen, J., and M. O’Neil. 2005.
Degradation of unconsolidated Quaternary landforms in the western North America. Geomorphology 75: 408-419.
Reed, J.C. 1961.
Geology of the Mt. McKinley Quadrangle, Alaska. U.S. Geological Survey Bulletin 1108-A.
Ten Brink, N.W., and C.F. Waythomas. 1984.
Late Wisconsin glacial chronology of the North-Central Alaska Range – A regional synthesis and its implications for early human settlements. In North Alaska Range Project final report on 1978-1982 geo-archeological studies.
Werner, A. 1982.
Glacial geology of the McKinley River area, Alaska: with an evaluation of various relative age dating techniques. Masters thesis. Department of Geology, Southern Illinois University.
Werner, A., and J.C. Child. 1995.
Glacial history of the McKinley River area, Denali National Park and Preserve, Alaska. Geologic Society of America Abstracts with Programs 27: 83.
Last updated: April 7, 2021