Part of a series of articles titled Alaska Park Science - Volume 17, Issue 1. Migration: On the Move in Alaska.
Article
Future Challenges for Salmon and the Freshwater Ecosystems of Southeast Alaska
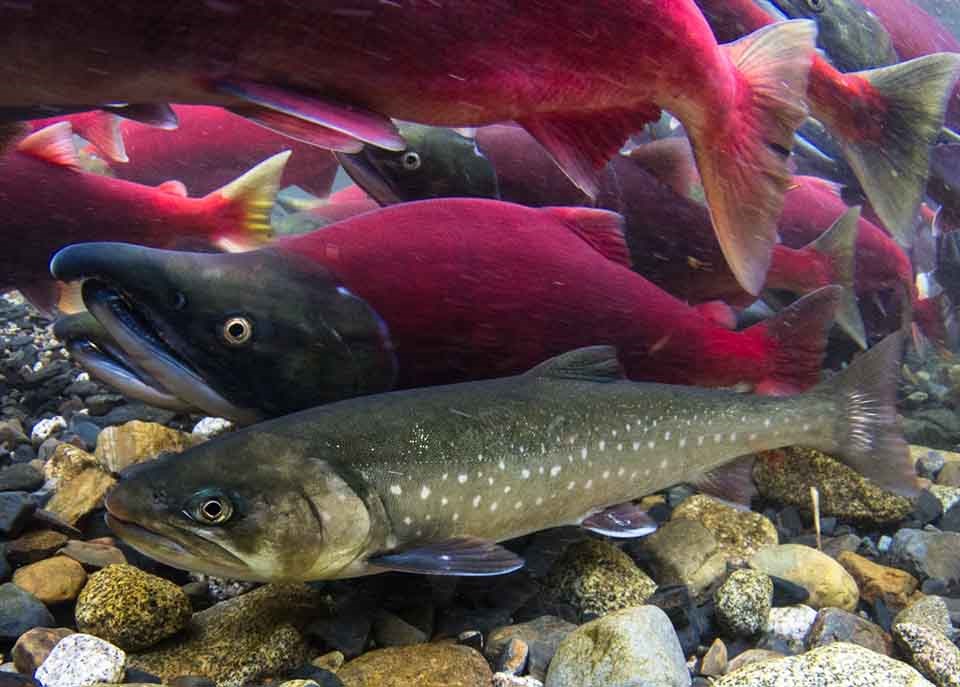
Photo courtesy of J. Armstrong
Mass animal migrations are awe-inspiring sights. Every summer and fall, residents and visitors to Alaska can witness one of the great underwater migrations: Pacific salmon returning from the ocean to their home streams, rivers, and lakeshores to spawn. Hundreds of millions of salmon return to Alaska’s freshwaters annually (Stopha 2018). These anadromous fish are born in freshwater, migrate to saltwater to accrue the majority of their mass, and then return to their natal stream to spawn and die. The diversity of habitats used by Pacific salmon is staggering: large lakes, beaver ponds, icefields, wetlands, and waterfalls all play important roles in the salmon’s web of life (O’Neel et al. 2015). Some individuals will fight strong flows for hundreds of miles to spawn in the tributaries of massive international watersheds like the Yukon River, while some will effortlessly slip several yards up one of the hundreds of tiny creeks lining the Alexander Archipelago in Southeast Alaska.
Naturalists have known since the 16th century that salmon typically return to their place of birth to spawn (Quinn 2005). This unique aspect of their life cycle continues to provide coastal and interior Alaskan communities with dependable sustenance year after year. The importance of salmon to Alaska cannot be understated. Salmon fisheries have the greatest economic impact on the Alaskan seafood industry, providing thousands of jobs to residents every year (McDowell Group 2017). In 2016, The Nature Conservancy conducted a poll showing that 96% of Alaskans believe salmon are essential to their way of life. For a group of species this important to society, it is imperative for scientists to continue learning more about the various salmon species residing in Alaskan waters and anticipate future challenges for their populations. Fluctuating ocean survival and harvest strategies undoubtedly play an important role in regulating salmon populations (Mantua et al. 1997, Mundy 1997), but in this article I concentrate on freshwater ecosystems, where the habitat provided by national parks plays a large role in the salmon life cycle.
The five primary species of semelparous (spawning once before dying) Pacific salmon each have their own unique life histories (Table 1), but all generally require cool, clean rivers with shallow water for spawning and deep pools for holding and migrating upriver. While ocean and estuary environments play critical roles in salmon growth and survival, changes in the abundance of salmon in rivers may indicate shifts in the habitat characteristics of freshwater ecosystems and potentially broader changes to park natural resources and visitor experience.
Species | Scientific Name | General Life History |
---|---|---|
Chinook | Oncorhynchus tshawytscha |
|
Chum | Oncorhynchus keta |
|
Coho | Oncorhynchus kisutch |
|
Pink | Oncorhynchus gorbuscha |
|
Sockeye | Oncorhynchus nerka |
|
Salmon benefit the freshwater ecosystems they return to, transporting energy and nutrients gained from the ocean (Gende et al. 2002) and directly feeding more than fifty species of animals (Willson and Halupka 1995). This is not news to most Alaskans, but what is surprising is that scientists continue to learn more about the current and future challenges threatening this important group of species. Here, I describe some recent salmon research in Southeast Alaska and touch on future issues of concern in the region’s three national parks: Glacier Bay National Park and Preserve, Klondike Gold Rush National Historical Park, and Sitka National Historical Park.
Climate Change Influences Salmon Migration Patterns and River Habitat
Whether it is a new river channel formed by a large landslide or completely new watershed sitting at the foot of a retreating glacier, salmon have adapted their migration pathways and timing to a constantly shifting habitat mosaic for thousands of years. The coming decades present new challenges and it is unclear if salmon will be able to keep pace with them. In Southeast Alaska, climate models predict rising air temperatures, increasing annual precipitation, and decreasing precipitation falling as snow (Shanley et al. 2015). Increased winter flooding due to higher precipitation amounts will promote active downstream transport of sediment and likely decrease the survival of salmon eggs buried in gravel during winter (Shanley and Albert 2014). The future timing of upriver migration to spawn and deposit eggs will play an important role in each population’s continued success. The extent to which increased flooding will affect important habitat characteristics such as the presence of deep pools, streambank stability, and the density of large embedded trees that help form and maintain these features is understudied in this region. Changing streamflow patterns and subsequent impacts to salmon populations were major topics of discussion in 2012, when National Park Service staff, other agency representatives, and local and tribal leaders met in Juneau for the Southeast Alaska Climate Change Scenario Planning Workshop (Winfree et al. 2014).
Even though total annual precipitation and frequency of winter floods are likely to increase in Southeast Alaska, decreasing snowpack will decrease the amount of water available for spawning salmon in the summertime. Rain- and snow-dominated watersheds without glaciers will see minimum summer flows continue to shrink during periods of low precipitation, often during periods overlapping with peak salmon spawning migrations. Recent water quality monitoring data from the Indian River in Sitka National Historical Park have demonstrated that low streamflow can combine with high salmon abundance to create low dissolved oxygen (hypoxic) events that lead to resident stream fish and salmon mortality, even in relatively cold streams that have the capacity to hold a lot of oxygen (Sergeant et al. 2017). In other words, many salmon breathing at the same time in a slow-moving river can create hypoxic conditions, which can lead to delayed upstream migration and even premature death.
Humans Magnify the Effects of Salmon Migration on Freshwater Ecosystems
Human-driven activities such as river water diversion and straying hatchery salmon (salmon that return to streams instead of their intended hatchery of origin) further increase the probability of hypoxic events by crowding more fish into smaller volumes of water. Recent straying rates in the Indian River have ranged as high as 62%, while other southern Alaska streams have approached 100% at times (Figure 1; Brenner et al. 2012, Piston and Heinl 2012, Hollowell et al. 2016). The extent that hatchery salmon impact wild salmon populations and their recovery has been debated for decades in the Pacific Northwest (Brannon et al. 2004, Mobrand et al. 2005). This is likely to become a more common debate in Alaska in the coming years. Additionally, escaped Atlantic salmon from aquaculture net pens may potentially compete with native Pacific salmon stocks by colonizing stream habitat; this remains a concern along the northwestern coastline of North America.
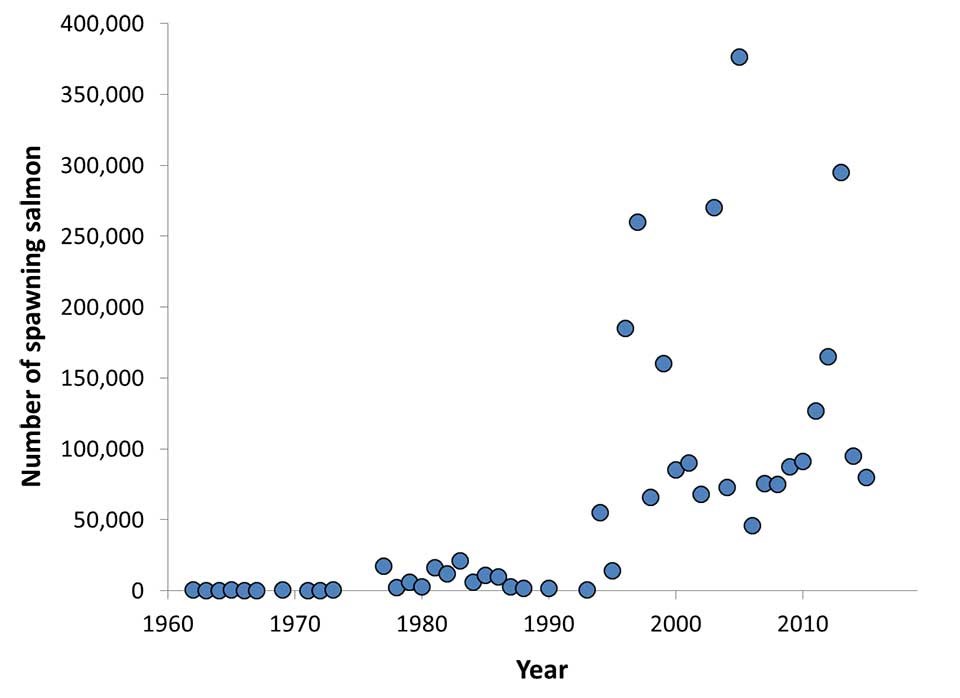
The hatchery began annual releases of pink salmon fry in 1975. From 2013-2015, hatchery stray salmon rates ranged from 0-62% depending on the time of year surveyed (S. Gende/NPS, unpublished data).
Watershed Characteristics and Salmon Combine to Influence Freshwater Contaminants
In contrast to most watersheds in the Pacific Northwest, Southeast Alaska watersheds have significant glacial and wetland coverage, and these two landscape features play important roles in contaminant transport. As glaciers continue to diminish (Loso et al. 2014), they may release contaminants previously deposited from the atmosphere and locked in ice. Sulfate-reducing bacteria in wetlands efficiently convert atmospheric mercury to methylmercury, a more toxic and bioavailable form of mercury that is dangerous to humans and other animals (Nagorski et al. 2011). Salmon returning to spawn may also act as biovectors that transport marine-derived contaminants to freshwaters. Thus, the combination of glacier, wetlands, and dense salmon populations increases the possibility that freshwater contaminants will be a continuing concern in this region. But for mercury accumulation, the role of salmon as biovectors in some streams may be counter-intuitive.
Many stream-dwelling fish, such as Dolly Varden (Salvelinus malma), have evolved to closely track the spawning migrations of salmon to gorge on their eggs and gain an important energetic boost before the lean feeding times of winter (Sergeant et al. 2015). Because salmon eggs are naturally low in mercury, recent research in Klondike Gold Rush and Sitka national historical parks demonstrated that Dolly Varden that feed on abundant salmon eggs actually have lower mercury concentrations than their cohorts residing in areas above migration barriers where salmon eggs are not available (Cyr et al. 2016). It remains unknown whether Dolly Varden foraging on salmon eggs influences the patterns of concentration of other contaminants.
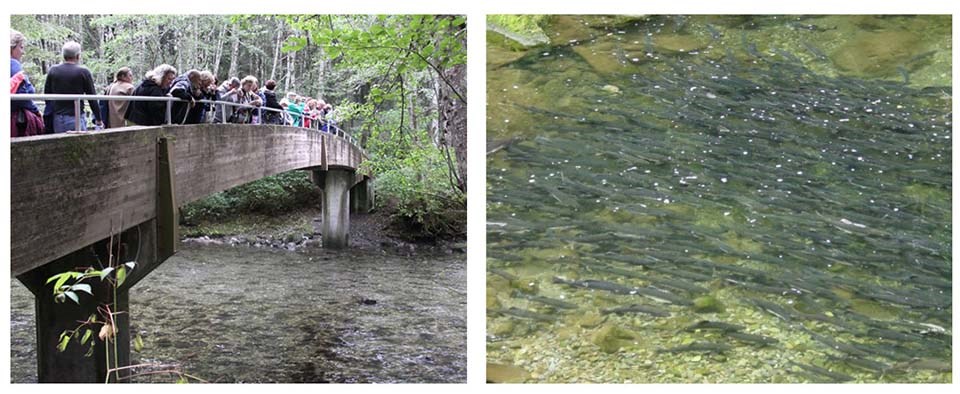
Conclusion
Scientists continue to gain a more nuanced view of the roles of salmon in the freshwater ecosystems of Southeast Alaska. We know from studies at Glacier Bay National Park and Preserve that salmon colonize new habitat and adapt to changing conditions (Milner et al. 2011, Scribner et al. 2017). This flexibility in habitat choices and migration timing in response to local changes is often called “plastic” behavior. An important question is whether the plasticity of salmon can keep pace with rapid change brought about by the climate and human development (Crozier et al. 2008).
One thing is certain, though: salmons’ roles are hard to generalize and are very species- and river-specific. For example, many sockeye salmon populations in Southeast Alaska are migrating later than they have historically, while pink, chum, and coho salmon are migrating earlier (Kovach et al. 2015). From a National Park Service natural resource conservation and visitor experience perspective, it will be important to study the unique characteristics of salmon populations within each park and track population abundance and spatial distribution of juveniles and adults to understand future change. Basic monitoring of the number of juvenile salmon migrating downstream to the ocean and the number of adults migrating upstream to spawn is missing for most park rivers. In order for natural resource managers to have a more accurate perspective of trends in salmon abundance and distribution, parks should maintain long-term data collection where it already exists and strive to consolidate existing data sets to assess the ranges of variability and rates of change in populations.
The expansive and wild character of Alaska parklands creates many field challenges. While traditional aerial survey escapement counts and weirs will likely play a role in these efforts, newer technology such as Dual-frequency Identification Sonar (DIDSON), which uses sound to image underwater migrating salmon, or aerial drones may be important technology to consider, as well. Moving into the future, Alaska parks will need to elevate the importance of salmon to the health of freshwater resources, promote fisheries conservation efforts, create or maintain salmon-based interpretive opportunities for visitors, and advance freshwater ecosystem monitoring in priority streams and rivers.
Acknowledgements
N. Chambers, L. Phillips, C. Soiseth, B. Carter, and one anonymous reviewer provided helpful reviews of this article.
REFERENCES
Brannon, E. L., D. F. Amend, M. A. Cronin, J. E. Lannan, S. LaPatra, W. J. McNeil, R. E. Noble, C. E. Smith, A. J. Talbot, G. A. Wedemeyer, and H. Westers. 2004.
The controversy about salmon hatcheries. Fisheries 29: 12-31.
Brenner, R. E., S. D. Moffitt, and W. S. Grant. 2012.
Straying of hatchery salmon in Prince William Sound, Alaska. Environmental Biology of Fishes 94: 179-195.
Crozier, L. G., A. P. Hendry, P. W. Lawson, T. P. Quinn, N. J. Mantua, J. Battin, R. G. Shaw, and R. B. Huey. 2008.
Potential responses to climate change in organisms with complex life histories: evolution and plasticity in Pacific salmon. Evolutionary Applications 1: 252-270.
Cyr, A., C. J. Sergeant, J. A. Lopez, and T. O’Hara. 2016.
Assessing the influence of migration barriers and feeding ecology on total mercury concentrations in Dolly Varden (Salvelinus malma) from a glaciated and non-glaciated stream. Science of the Total Environment 580: 710-718.
Gende S.M., R. T. Edwards, M. F. Willson, and M. S. Wipfli. 2002.
Pacific salmon in aquatic and terrestrial ecosystems. BioScience 52: 917-928.
Hollowell, G., E. O. Otis, and E. Ford. 2016.
2015 Lower Cook Inlet area finfish management report. Alaska Department of Fish and Game, Fishery Management Report No. 16-19, Anchorage.
Kovach, R. P., S. C. Ellison, S. Pyare, and D. A. Tallmon. 2015.
Temporal patterns in adult salmon migration timing across southeast Alaska. Global Change Biology 21: 1821-1833.
Loso, M., A. Arendt, C. Larsen, J. Rich, and N. Murphy. 2014.
Alaskan national park glaciers - status and trends: Final report. Natural Resource Technical Report NPS/AKRO/NRTR—2014/922. National Park Service, Fort Collins, Colorado.
Mantua, N. J., S. R. Hare, Y. Zhang, J. M. Wallace, and R. C. Francis. 1997.
A Pacific interdecadal climate oscillation with impacts on salmon production. Bulletin of the American Meteorological Society 78:1069-1079.
McDowell Group. 2017.
The economic value of Alaska’s seafood industry. Available at: http://www.mcdowellgroup.net/wp-content/uploads/2017/10/ak-seadfood-impacts-sep2017-final-digital-copy.pdf (accessed 2 April 2018)
Milner, A. M., A. L. Robertson, L. E. Brown, S. H. Sonderland, M. McDermott, and A. J. Veal. 2011.
Evolution of a stream ecosystem in recently deglaciated terrain. Ecology 92: 1924-1935.
Mobrand, L. E., J. Barr, L. Blankenship, D. E. Campton, T. T. P. Evelyn, T. A. Flagg, C. V. W. Mahnken, L. W. Seeb, P. R. Seidel, and W. W. Smoker. 2005.
Hatchery reform in Washington State: principles and emerging issues. Fisheries 30: 11-23.
Mundy, P. R. 1997.
The role of harvest management in the future of Pacific salmon populations: shaping human behavior to enable the persistence of salmon. In Pacific Salmon & their Ecosystems. P. 315-329. Springer, Boston, Massachusetts.
Nagorski, S., D. Engstrom, J. Hudson, D. Krabbenhoft, J. DeWild, E. Hood, and G. Aiken. 2011.
Scale and distribution of global pollutants in Southeast Alaska Network park watersheds. Natural Resource Technical Report NPS/SEAN/NRTR—2011/496. National Park Service, Fort Collins, Colorado.
O’Neel, S. O., E. Hood, A. L. Bidlack, S. W. Fleming, M. L. Arimitsu, A. Arendt, E. Burgess, C. J. Sergeant, A. H. Beaudreau, K. Timm, G. D. Hayward, J. H. Reynolds, and S. Pyare. 2015.
Icefield-to-ocean linkages across the northern Pacific coastal temperate rainforest ecosystem. Bioscience 65: 499-512.
Piston, A. W. and S. C. Heinl. 2012.
Hatchery Chum Salmon Straying Studies in Southeast Alaska, 2008-2010. Alaska Department of Fish and Game, Fishery Manuscript Series No. 12-01, Anchorage.
Quinn, T. P. 2005.
The behavior and ecology of Pacific salmon and trout. University of Washington Press, Seattle, Washington, USA.
Scribner, K. T., C. Soiseth, J. McGuire, G. K. Sage, L. Thorsteinson, J. L. Nielsen, and E. Knudsen. 2017.
Genetic assessment of the effects of streamscape succession on coho salmon Oncorhynchus kisutch colonization in recently deglaciated streams. Journal of Fish Biology DOI:10.1111/jfb.13337.
Sergeant, C. J., J. R. Bellmore, C. McConnell, and J. W. Moore. 2017.
High salmon density and low discharge create periodic hypoxia in coastal rivers. Ecosphere 8: e01846
Sergeant, C. J., J. B. Armstrong, and E. J. Ward. 2015.
Predator-prey migration phenologies remain synchronized in a warming catchment. Freshwater Biology 60: 724-732.
Shanley, C. S., S. Pyare, M. I. Goldstein, P. B. Alaback, D. M. Albert, C. M. Beier, T. J. Brinkman, R. T. Edwards, E. Hood, A. MacKinnon, M. V. McPhee, T. M. Patterson, L. H. Suring, D. A. Tallmon, and M. S. Wipfli. 2015.
Climate change implications in the northern coastal temperate rainforest of North America. Climatic Change 130: 155-170.
Shanley, C. S. and D. M. Albert. 2014.
Climate change sensitivity index for Pacific salmon habitat in Southeast Alaska. PLoS ONE 9: e104799.
Stopha, M. 2018.
Alaska salmon fisheries enhancement annual report 2017. Alaska Department of Fish and Game, Division of Commercial Fisheries, Regional Information Report 5J18-02, Juneau.
Stopha, M. 2015.
An evaluation of the Sheldon Jackson Salmon Hatchery for consistency with statewide policies and prescribed management practices. Alaska Department of Fish and Game Regional Information Report 5J15-07.
Willson, M. F. and K. C. Halupka. 1995.
Anadromous fish as keystone species in vertebrate communities. Conservation Biology 9: 489-497.
Winfree, R., B. Rice, N. Fresco, L. Krutikov, J. Morris, D. Callaway, D. Weeks, and J. Mow. 2014.
Climate change scenario planning for southeast Alaska parks: Glacier Bay, Klondike, Sitka, and Wrangell-St. Elias. Natural Resource Report NPS/AKSO/NRR—2014/831. National Park Service, Fort Collins, Colorado.
Last updated: June 8, 2018