In July 2012, aquatic ecologist Amy Larsen and pilot Eric Sieh discovered multiple mammoth bones along the edge of a lake in Bering Land Bridge National Preserve. They reported finding at least one tooth and a semi-articulated humerus and ulna (Figure 1). Archaeologist Jeff Rasic, Louise Farquharson (a PhD student at the University of Alaska Fairbanks), and Eric Sieh revisited the site in September, to assess the site’s vulnerability to erosion and potential for paleoecological research.
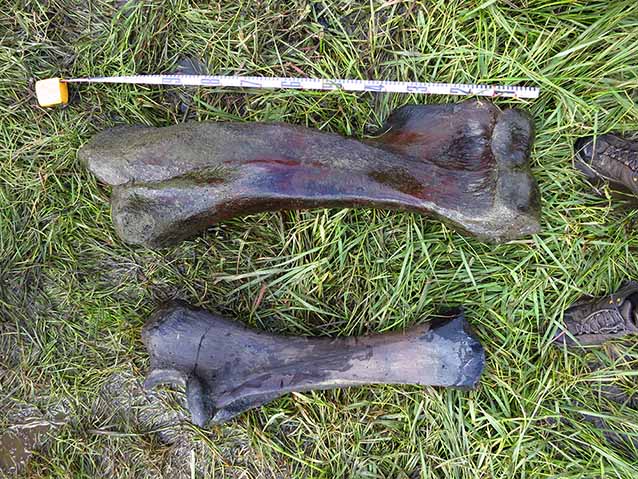
NPS Photo / Jeff Rasic
In addition to relocating the mammoth humerus and ulna, they discovered two mammoth vertebra, a mammoth rib fragment, potential mammoth cranial bones, caribou antler fragments, and a moose metapodial (Figure 2). They found the majority of the mammoth bones clustered together, indicating they likely originated from a single individual. Finds of multiple mammoth bones from the same skeleton are relatively uncommon in Alaska, making this one of the more complete mammoth skeletons known.
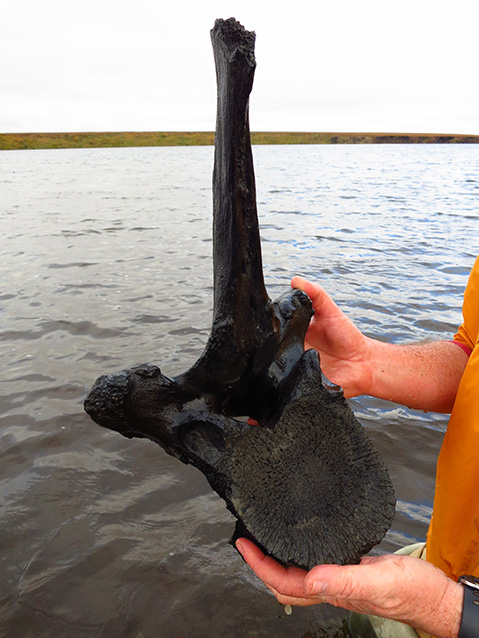
NPS Photo / Jeff Rasic
While exciting and valuable, fossil finds of this nature are not uncommon from within the Arctic parks. Pleistocene (0.01–2.6 Ma [million years ago]) mammal fossils, such as the mammoth bones from Bering Land Bridge, can be found eroding out surficial deposits throughout the parks (Figure 3). Furthermore, many of the Paleozoic (252–541 Ma), and to a lesser extent Mesozoic (66-252 Ma) rocks that form the characteristically majestic landscapes of the Arctic parks contain abundant marine fossils, including trilobites, ammonites, brachiopods, gastropods, and many more. These fossils tell the story of how this area has evolved through millions of years, from a time when trilobites swarmed the ocean floors, to when huge Ice Age megafauna traveled freely between Siberia and North America. Without an understanding of this story told through fossils, it would be nearly impossible to understand the geologic history of the Arctic parks, Alaska, and the Earth as a whole.
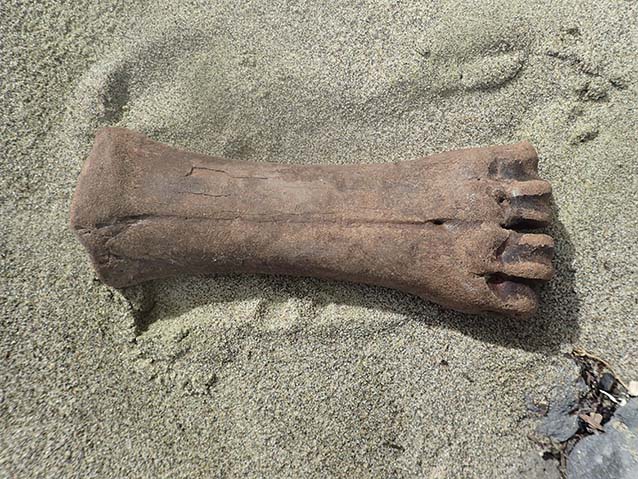
NPS Photo / Mariana Dryak
What is a Fossil?
When most people hear the word “fossil,” they typically picture the enormous dinosaur skeletons that are often displayed in museums. As majestic as these striking paleontological specimens are, they make up only a small portion of the wide variety of organisms that have been preserved as fossils. Fossils, strictly speaking, are any evidence of past life that has been preserved in the rock record. This encompasses microscopic 3.5 billion year-old cyanobacteria, 15,000 year-old wooly mammoths, and everything in between. The study of these ancient organisms (paleontology) allows an understanding of how life on Earth has changed through time. Because life is always changing, the fossils in a rock directly reflect the time, place, and environment in which that rock was deposited. This makes the fossil record an invaluable tool not only for studies that focus on how life has changed and evolved through time, but also for any geologic study that could benefit from spatial, temporal, or environmental constraint.
Paleontological Inventory
Fossils can provide geologists a wealth of important information about the rocks in which they are found. When and where were these rocks deposited? What was the environment like then and how has it changed? What other organisms composed this ancient ecosystem? Fossils can answer all of these questions and many more. Because of their inherent value, it is vital to document where fossils have already been found and identify areas of high fossil potential. This information enables park staff to ensure the preservation of these important, non-renewable resources and facilitates further paleontological research. Toward this end, we are compiling a comprehensive report and database of the paleontology of the Arctic parks, which will identify what paleontological resources the parks contain, the condition of these resources, and their potential vulnerability to disturbance. The following is a brief account of just a portion of the interesting paleontological research that has already been conducted within the Arctic parks, highlighting cases when fossils provided key evidence that enabled scientists to resolve complex geologic questions.
Geologic History
Arctic parks, like most of Alaska, are composed of multiple accreted geologic terranes (Figure 4). A terrane is a fault-bounded package of rocks with a geologic history different from surrounding rocks. Alaska is almost entirely composed of terranes, each with its own history, that have been transported from where they were originally deposited and amalgamated together through tectonic forces.
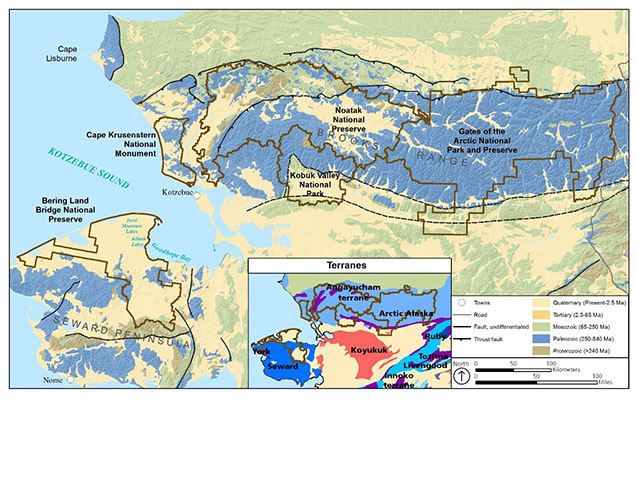
One of the largest of these terranes is the Arctic Alaska terrane, underlying all of the Arctic parks except Bering Land Bridge National Preserve, which contains rocks of the Seward and York terranes (Silberling et al. 1992). Within the park boundaries these terranes are primarily Paleozoic in age and were deposited on the marine margins of a continent. The biogeographic affinities of the fossils found in the Early Paleozoic rocks indicate that they were deposited in close proximity to Siberia, rather than North America. This Siberian aspect differentiates them from coeval carbonate deposits of the Canadian passive margin, indicating that despite being transported to northern Canada by the end of the Paleozoic, these rocks have an exotic origin with respect to North America.
The Arctic Alaska terrane, along with the terranes of Bering Land Bridge and parts of northeastern-most Russia (notably Chukotka), are interpreted as belonging to a continental block called the Arctic Alaska-Chukotka microplate. The Arctic Alaska-Chukotka microplate has been suggested by some to have rifted away from the Canadian margin during the Mesozoic with the opening of the Canada Basin (Till 2016). This coincides with the beginning of the Brookian orogensis, a mountain-building event caused by the collision of the continental Arctic Alaska terrane and the Koyukuk arc, closing the oceanic Angayucham Ocean (Moore et al. 2015). This collision resulted in the folding and thrusting of the Arctic Alaska terrane, emplacement of the Angayucham terrane onto the Arctic Alaska terrane, and formation the Brooks Range and the Colville basin (Moore et al. 1994). The Arctic parks straddle the Brooks Range, an area of uplift (rather than deposition) during the Mesozoic, and therefore deposits sourced from the uplifted area are not nearly as widespread within the parks as Paleozoic deposits.
In addition to older rocks, the Arctic parks contain a wealth of unconsolidated Pleistocene and Holocene (Present–0.01 Ma) sediments. During these epochs, the Earth went through a series of major glaciations. Ice sheets tied up vast quantities of water on land, causing sea level to drop and the shallow marine platform between Alaska and Siberia to emerge, forming the Bering Land Bridge (Hopkins 1959). The Bering Land Bridge occupied the center of an area known as Beringia that stretched from Siberia to Northern Canada and remained largely unglaciated during the last glacial maximum (LGM; 14,000–28,000 years before present; Hofle et al. 2000). The Arctic parks are located in what was central and eastern Beringia, an arid steppe that hosted large herds of grazing animals such as horses, bison, and mammoths. These deposits preserve the record of how animals and plants interacted through periods of biotic interchange and dramatic climate change at high latitudes (Lenz et al. 2016).
Paleozoic Paleontology
To determine the geographic placement of the Arctic Alaska, York, and Seward terranes during the Early Paleozoic, geologists have relied on the fossilized organisms found within them. Fossils usually reflect the time and place of that rock’s deposition. Species that show provincialism (endemic species, meaning that they are restricted to a small geographic region), are especially good for providing geographic constraint. Because of their depositional setting, the Arctic Alaska, Seward, and York terranes contain abundant fossils typically found in Paleozoic carbonate sequences, including trilobites, brachiopods, sponges, corals, mollusks, and conodonts. Some of the macrofauna, and to a lesser extent the macroflora, demonstrate ties to Siberia (Blodgett et al. 2002), while the early Paleozoic conodonts recovered from these terranes display a mixed Siberian and North American affinity (Dumoulin et al. 2002).
There are numerous and varied fossils from Cambrian (485–541 million years ago) to Mississippian (323–359 Ma) strata of the Arctic Alaska terrane that are endemic to Siberia (Figure 5). These include Cambrian trilobites from the eastern and central Brooks Range (Dutro et al. 1984, Palmer et al. 1984), and Mississippian plant macrofossils that were previously only known from Siberia (Blodgett et al. 2002). Numerous Ordovician- (444–485 Ma) to Devonian- (359–419 Ma) aged species of brachiopods recognized from the Arctic Alaska terrane also indicate a depositional environment geographically linked to Siberia (Figure 6; Blodgett et al. 2002). In addition, microfossils demonstrate Paleozoic ties to Siberia; Early Paleozoic conodonts from portions of the Arctic Alaska, York, and Seward terranes are of a mixed Siberian and North American affinity (Dumoulin et al. 2002). These paleontological data indicate that the northern Alaskan terranes were proximal to Siberia from the Cambrian to Devonian, and possibly into the Mississippian (Blodgett et al. 2002).
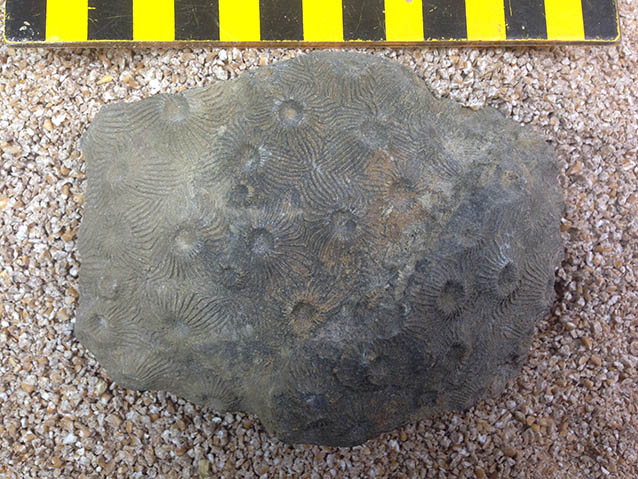
Photo courtesy of Robert B. Blodgett
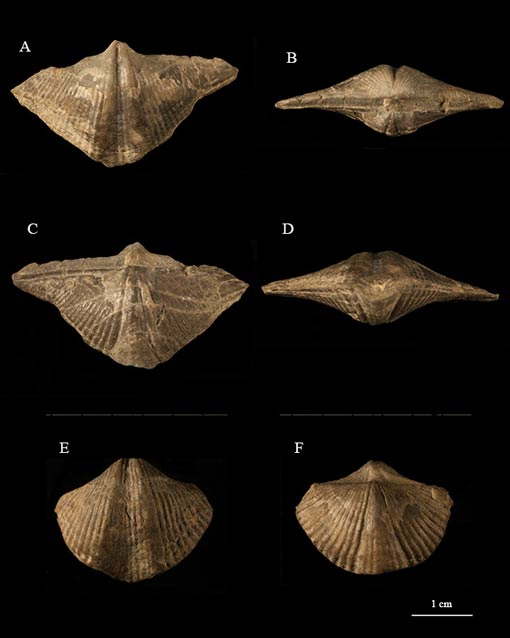
Courtesy of Robert B. Blodgett
Quaternary Paleontology
Fossils can be found throughout the Arctic parks, eroding out of surficial Pleistocene and Holocene deposits. These deposits contain a wide variety of fossils, notably the bones and teeth of Ice Age megafauna, but also abundant pollen, insect, ostracod, and plant fossils.
One of the most unique deposits that provides a window into the past environment of Beringia is the 21,570 year-old Kitluk Paleosol, found within the Bering Land Bridge National Preserve. In the area between Devil Mountain and Cape Espenberg, Pleistocene and Holocene volcanism produced ash deposits with at least one of these eruptions producing an ash layer that buried an area of approximately 750 km2 (289 square miles). The deposition of this ash caused the permafrost to advance upward rapidly and essentially froze the soil in place (Hofle and Ping 1996). This exquisitely preserved fossilized soil, named the Kitluk Paleosol, is now exposed along the perimeters of the many thermokarst lakes on the Seward Peninsula (Kuzmina et al. 2008). During the summers of 1993, 1994, and 1995, a multi-disciplinary team of scientists excavated the Kitluk Paleosol, collecting insect fossils, arachnid fossils (Kuzmina et al. 2008), and plant macrofossils (Goetcheus and Birks 2001). These fossils, along with the other characteristics of the ancient soil, allowed scientists to reconstruct the past environment of a large portion of central Beringia at a single point in time. When integrated with studies from Alaska, Siberia, and Canada, we begin to get a picture of this glacial refuge that enabled the survival and dispersal of various Ice-Aged plants and animals, including the first humans of North America.
During the Pleistocene, Beringia was home to variety of large, cold-adapted mammals. Some of the most commonly found remains include those belonging to wooly mammoth (Mammuthus primigenius), horse (Equus sp.), steppe bison (Bison priscus), caribou (Rangifer tarandus), muskox (Ovibos moschatus), and grizzly bear (Ursus arctos; Figure 7). These fossils can be found eroding out of stream banks and coastal bluffs in the Arctic parks and provide excellent material to study the megafauna that inhabited Beringia, their subsequent extinction, and the circumstances surrounding it (Rivals et al. 2010). The majority of scientists agree that climate change and the loss of habitat were the main factors behind the decline of the Ice Age megafauna, however, some have argued that the migration of humans into North America also played a role.
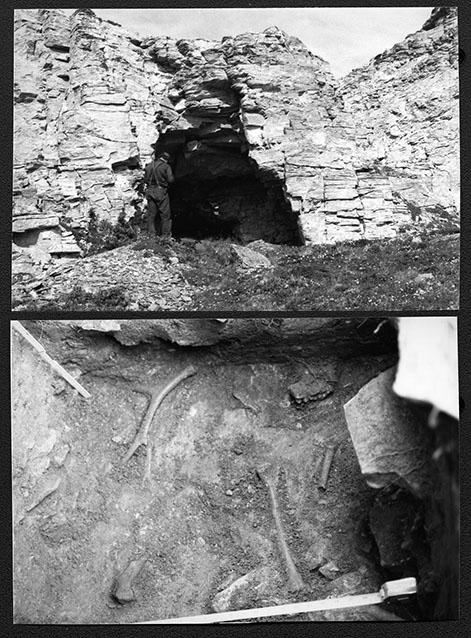
NPS archives; Bering Land Bridge Cultural Resource Inventory, 1985
During the Pleistocene and Holocene, sea-level oscillations caused the sea level to drop as much as 100–150 meters (328-492 feet) and rise at least 20 meters (65 feet) above present position (Hopkins 1967, Hopkins 1988, Kaufman and Brigham-Grette 1993, Brigham-Gette and Hopkins 1995, Muhs et al. 2003). During times of high sea level, marine fossils were deposited in flooded areas that are presently above sea level. The majority of these marine deposits are obscured by overlying terrestrial sediment, however, we can find marine mollusk shells in areas along the coast of Bering Land Bridge National Preserve and Cape Krusenstern National Monument (Brigham-Grette and Hopkins 1995). Interestingly, the Devil Mountain Lakes, two maar lakes located on the northern portion of the Seward Peninsula, are ringed by rocks containing marine mollusk shells. The Devil Mountain maars were formed 7,100 and 17,000 years ago, when the interaction of magma and groundwater caused violent volcanic eruptions. The marine mollusk shells are found in volcanic ejecta that was brought up from the subsurface during these eruptions, indicating that Pleistocene marine deposits extend at least as far inland as these lakes (Hopkins 1988). Times of high sea level are important to understand, because while the flooding of the Bering Land Bridge restricted the migration of terrestrial life between North America and Asia, the opening of the Bering Strait altered ocean circulation and allowed for the exchange of Arctic and Pacific marine organisms.
Virtual Paleontological Specimens
As part of our inventory of Arctic paleontology, we are creating a database to provide park staff with easy access to paleontological data and highlight opportunities for future research and outreach. One project idea that stemmed out of the database is the creation of three-dimensional models of park fossils. Three-dimensional models are an especially exciting new tool in paleontology because they allow anyone the opportunity to observe and interact with fossils. To create the models, fossils are photographed from multiple angles and the images are stitched together in a process known as Structure from Motion (photogrammetry; Figure 8). We’ve created three-dimensional models of some of the key park fossils, including the mammoth tooth collected from Bering Land Bridge National Preserve. This provides the opportunity for researchers everywhere to study and appreciate the vast paleontological resources Alaska’s parks have to offer.
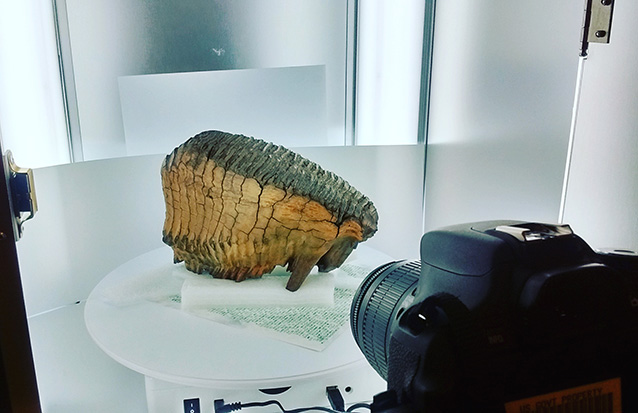
NPS Photo / Amanda Lanik
Acknowledgements
The authors would like to thank Jeff Rasic, NPS Archaeologist, and Andrew Tremayne, NPS Archaeologist, for providing field photos of fossils. We would also like to acknowledge the support of the Geoscientists-in-the-Parks Program through the Environmental Stewards and the Geological Society of America.
References
Blodgett, R. B., D. M. Rohr, and A. J. Boucot. 2002.
Paleozoic links among some Alaskan accreted terranes and Siberia based on megafossils. Pages 273-290 in E. L. Miller, A. Grantz, and S. L. Klemperer, eds. Tectonic Evolution of the Bering Shelf—Chukchi Sea—Arctic Margin and Adjacent Landmasses. Geological Society of America Special Paper 360. The Geological Society of America, Boulder, Colorado.
Brigham-Grette, J. and D.M. Hopkins, 1995.
Emergent marine record and paleoclimate of the Last Interglaciation along the northwest Alaskan coast. Quaternary Research 43:159-173.
Dumoulin, J. A., A. G. Harris, M. Gagiev, D. C. Bradley, and J. E. Rapetski. 2002.
Lithostratigraphic, conodont, and other faunal links between lower Paleozoic strata in northern and central Alaska and northeastern Russia. Pages 291-312 in E. L. Miller, A. Grantz, and S. L. Klemperer, eds. Tectonic Evolution of the Bering Shelf—Chukchi Sea—Arctic Margin and Adjacent Landmasses. Geological Society of America Special Paper 360. The Geological Society of America, Boulder, Colorado.
Dutro, J.T., A.R. Palmer, J.E. Repetski, and W.P. Brosgé. 1984.
Middle Cambrian fossils from the Doonerak anticlinorium, central Brooks Range, northern Alaska. Journal of Paleontology 58(6):1364-1371.
Goetcheus, V. G. and H. H. Birks. 2001.
Full-glacial upland tundra vegetation preserved under tephra in the Beringia National Park, Seward Peninsula, Alaska. Quaternary Science Reviews 20:135-147.
Hopkins, D. M. 1959.
Cenozoic History of the Bering Land Bridge. Science 129:1519-1528.
Hopkins, D. M. 1967.
Quaternary Marine Transgressions in Alaska. Pages 47-90 in D. M. Hopkins, ed. The Bering Land Bridge. Stanford University Press, Stanford, California.
Hopkins, D. M. 1988.
The Espenberg Maars: A record of explosive volcanic activity in the Devil-Mountain-Cape Espenberg area, Seward Peninsula, Alaska. Pages 262-321 in J. Schaaf, ed. The Bering Land Bridge National Preserve: an Archeological Survey 1. National Park Service – Alaska Region. Research/Resources Management Report AR-14, Anchorage, Alaska.
Hofle, C. and C. L. Ping. 1996.
Properties and soil development of late-Pleistocene paleosols from Seward Peninsula, northwest Alaska. Geoderma 71:219-243.
Hofle, C., M. E. Edwards, D. M. Hopkins, D. H. Mann, and C. L. Ping. 2000.
The full-glacial environment of the northern Seward Peninsula, Alaska, reconstructed from the 21,500-year-old Kitluk paleosol. Quaternary Research 53:143-153.
Kuzmina, S., S. Elias, P. Matheus, J. E. Storer, and A. Sher. 2008.
Paleoenvironmental reconstruction of the Last Glacial Maximum, inferred from insect fossils from a tephra buried soil at Tempest Lake, Seward Peninsula, Alaska. Palaeogeography, Palaeoclimatology, Palaeoecology 267:245-255.
Lenz J., G. Grosse, B. M. Jones, K. M. Walter Anothony, A. A. Bobrov, S. Wulf, and S. Wetterich. 2016.
Mid-Wisconsin to Holocene permafrost and landscape dynamics based on a drained lake basin core from the northern Seward Peninsula, northwest Alaska. Permafrost and Periglacial Processes 27:56-75.
Moore, T. E., W. K. Wallace, K. J. Bird, S. M. Karl, C. G. Mull, and J. T. Dillon. 1994.
Geology of northern Alaska. Pages 49-140 in G. Plafker and H. C. Berg, eds. The geology of Alaska. Geological Society of America, Boulder, Colorado.
Moore, T. E., P. B. O’Sullivan, C. J. Potter, and R. A. Donelick. 2015.
Provenance and detrital zircon geochronologic evolution of lower Brookian foreland basin deposits of the western Brook Range, Alaska, and implications for early Brookian tectonism. Geosphere 11(1):1-30.
Muhs, D. R., J. F. Wehmiller, K. R. Simmons, and L. L. York. 2003.
Quarternary sea-level history of the United States. Development in Quarternary Science 1:147-183.
Palmer, A. R., J. T. Dillon, and J. T. Dutro. 1984.
Middle Cambrian trilobites with Siberian affinities from the central Brooks Range, northern Alaska. Geological Society of America Abstracts with Programs 16(5): 327.
Rivals, F., M. C. Mihlbachler, N. Solounias, D. Mol, G. M. Semprebon, J. deVos, and D. C. Kalthoff. 2010.
Palaeoecology of the Mammoth Steppe fauna from the late Pleistocene of the North Sea and Alaska: Separating species preferences from geographic influences in paleoecological dental wear analysis. Palaeogeography, Palaeoclimatology, Palaeoecology 286:42-54.
Silberling, N. J., D. L. Jones, J. W. H. Monger, and P. J. Coney. 1992.
Lithotectonic terrane map of the North American Cordillera. U.S. Geological Survey, Miscellaneous Investigations Series 1-2176.
Till, A. B. 2016.
A synthesis of Jurassic and Early Cretaceous crustal evolution along the southern margin of the Arctic Alaska–Chukotka microplate and implications for defining tectonic boundaries active during opening of Arctic Ocean basins. Lithosphere 8(3):219-237.
Part of a series of articles titled Alaska Park Science - Volume 16 Issue: Science in Alaska's Arctic Parks.
Last updated: July 1, 2020