Last updated: February 26, 2024
Article
Lehman Caves: Little Understood but World Class, Part 2
This article was originally published in The Midden – Great Basin National Park: Vol. 18, No. 2, Winter 2018.
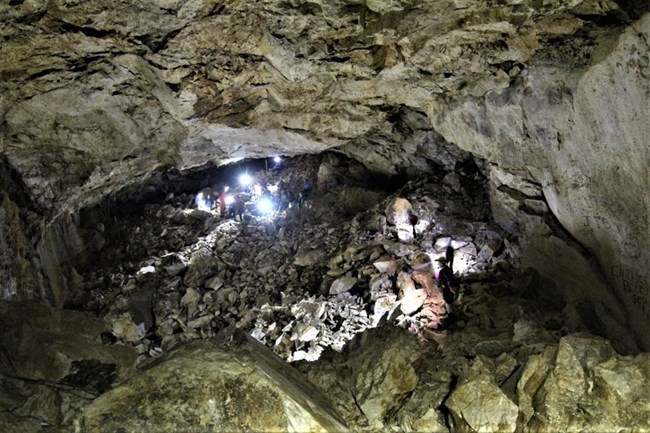
Gretchen Baker
The following article is excerpted from a paper I prepared for the Great Basin National Park staff on the Geologic Story of Lehman Caves. Last issue, I wrote about Stage 1, the Sulfde-rich, Hypogenic Speleogenesis time. This note reveals the second of four stages of speleogenesis I have documented in the cave.
STAGE 2 – SECONDARY DEPOSITS – FINDING STABILITY IN A NEW ENVIRONMENT
Once completely drained of hypogenic waters (more than 2.2 million years ago and likely about 8 Ma), the cave worked to achieve stability in its new environment. Three types of debris covered the cave floor before the dramatic speleothems formed. First, as soon as the water table dropped below the cave passages, gypsum paste fell off the walls and ceiling to cover the floor with gypsum sand and dust. What remained on the walls dried out and formed crystalline gypsum crust.
The second type of debris is mud, silt and sand. Some of this material is the insoluble residue from the dissolved marble which settled to the cave floor as the cave was actively enlarging. Some of it washed in from the surface, particularly near the natural entrance area.
The third type of debris resulted from collapse of boulders and rocks from the ceilings and floor. There were numerous causes of the “breakdown” in the cave.
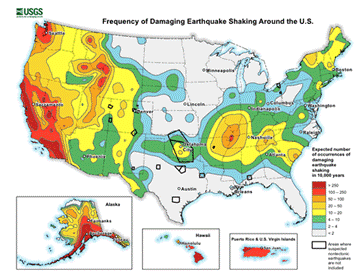
2. Movement along faults both in the cave and nearby during the Basin and Range tectonism resulted in earthquakes. Those events shook the entire area. Breakdown in the cave likely accompanied earthquakes at that time. The area now holds low risk of damaging earthquakes occurring (Fig. 1). As the area has been mostly earthquake-free for the last 8 million years, and the cave is more than 2.2 million years old, the cave ceiling and walls have likely reached a condition of metastability and present no more danger to visitors of spontaneous ceiling and wall collapse than the average cave visit or walk in the mountains anywhere.
3. The ceilings, no longer experiencing the partial buoyance of water, and walls, no longer under pressure from the water-filled passage, probably experienced the most active period of collapse as they moved towards stability right after the cave dewatered (millions of years ago).
4. As the area was uplifted and erosion removed most of the overburden, the release of lithostatic pressure (weight of rocks above) would have resulted in expansion of joints and other fractures in the bedrock, probably facilitating ceiling and wall collapses.
5. Several varieties of gypsum are abundant in the cave and crystal wedging undoubtedly contributed to the accumulation of breakdown on the cave floor. The conversion of limestone (CaCO3 ) to the much larger gypsum (CaSO4 . 2H2 O) molecule is accompanied by significant expansion within the rock. If the process takes place along joints or other cracks in the bedrock, the forces generated by the conversion pry the bedrock apart and commonly results in breakdown on the floor and forms blocky domes in the ceiling. This is a common process in Mammoth Cave, for example.
In conclusion, there is no reason to believe the cave is now particularly prone to ceiling or wall collapses and plenty of evidence from over 120 years of safe tours that it is as stable as most mountainous recreational sites. Most of the previous collapse occurred millions of years ago.