Last updated: November 7, 2022
Article
Final Report on The Friction Cone Penetrometer
June 15, 2017
Project Agreement Number 2210-13-0001 Between The National Center for Preservation Technology and Training Natchitoches, Louisiana and The Southeastern Archeological Center Tallahassee, Florida
Michael Russo and Chiang Shih
Executive Summary
This report describes the results of the Friction Cone Penetrometer (FCP) project partially funded by the National Park Service’s (NPS) National Center for Preservation Technology and Training (NCPTT) with funds provided in 2013 and the NPS’s Southeastern Archeological Center (SEAC) with funds provided from 2013 to 2016. Using the NCPTT funds, SEAC entered into a Cooperative Ecosystems Study Unit (CESU) agreement with the Department of Mechanical Engineering, Florida State University (FSU) to develop the prototype FCP. In the end, FSU contributed an untold amount of additional funding in support of its students working on the project as they endeavored to fix problems beyond the timeline funded by NCPTT and SEAC.
Ultimately, FSU teams could not manufacture a workable prototype FCP due to mechanical problems whose corrections lay beyond the given time constraints and funding of the project. In December 2016, FSU returned all purchased equipment to SEAC and submitted their final report (Pace 2016; Appendix F) which described the problems with the final prototype, one of three prototypes FSU constructed. In the end, we conclude that the concept of distinguishing archeological soils from non-archeological soils by measuring differences between soil cohesion and soil resistance is theoretically sound. But taking proven large-scale hydraulic technology that measures these differences effectively down to a portable mechanical instrument was too problematic to produce a working prototype with a limited budget.
Background
In 2013, SEAC’s Michael Russo applied for and obtained a grant from NCPTT to develop and test a prototype FCP which would measure soil resistance and cohesion by pushing a small diameter rod (< ½”) through the ground. The purpose of the FCP would be to test large tracts of land requiring archeological survey by identifying positive FCP responses for archeological soils, typically called organic midden soils, instead of using more labor intensive shovel tests.
For centuries, the method of pushing simple metal probes through the soil has been used to locate archeological objects and features by registering the operator’s feel of the different resistance and the sound the rod made as when it encountered objects. This simple technique has been used to find pots and skeletons in ancient Native American sites (Moore 1918) and across the world to find archeological objects from small items to large stone pyramids and monuments buried under Egyptian sands. One downside of probes is that they are susceptible to the subjectivity of the prober—some probers may feel an object as the probe grazes it, while others may not. Any positive hit would also require groundtruthing. For the modern archeologist seeking not to find objects, per se, but to define the boundaries of entire sites, hand-pushed probes were of limited utility in that extensive groundtruthing might be required if hundreds of positive hits were encountered. In addition, for archeological sites typically contain mostly soil with only a relatively small number of small artifacts, the chances of a probe hitting a perceivable object are statistically unlikely. As such, surveying land for archeological sites using only a probe that requires skilled and experienced probers, extensive ground truthing, or dense deposits to be successful is problematic. In the absence of skill or dense deposits, entire sites could be overlooked. For these reasons, extensive probing to find or bound sites has never gained favor among most archeologists and simple shovel testing has become the standard archeological survey methodology to find and define site boundaries.
In the 1960s, in addition to the simple metal rod, another type of metal probe, the cone penetrometer (CP) began to be used sparingly by archeologists. Employed in engineering and agriculture, CPs are used to measure soil resistance or compactness to determine the soil’s suitability for road construction or crop growth. Although many CPs are pushed through the ground just as simple metal rods are, others use weights to systematically pound a rod through the soils in question. In either case, CPs overcome the subjectivity of simple hand-pushed metal rods by providing an objective measure of soil resistance through a mechanical cell attached behind the probe tip that can be read by the operator on a mechanical, analog or digital gauge usually attached to the handle. Archeologists have typically to used CPs find unmarked pits at abandoned cemeteries where the disturbed soil of grave backfilled soil is usually less compacted than the surrounding undisturbed soils (e.g., Hailey and Ball 2006; Trinkley 1999, 2007; Trinkley and Southerland 2007; Zeidler 2005).
Adapted from the CP, a much larger, hydraulically driven instrument, the Friction Cone Penetrometer (FCP) has been commercially developed recently to distinguish one type of soil type from another. Mounted on large trucks and used extensively in mining exploration, in building and road construction, and to a lesser degree and more recently in agriculture, the tool measures both the compactness (resistance) of the soil as well as the soil friction and other soil traits to establish whether the behavioral characteristics of the soils are suitable for mining, road construction, or planting crops. Comparing the measures of the FCP to standard soil behavioral types (SBT), traits of soil can distinguish between SBTs such as clay, silts, sands and gravels.
These soils types are by standard definition distinguished by grain sizes. Grain size differences result in differences in resistance and friction as a probe is pushed through the soil. Russo hypothesized that grain size differences and relatively loose compactness are two features that might distinguish archeological middens from their surrounding natural soil matrices. As such, Russo proposed to develop a portable version of the hydraulic FCP to locate archeological midden sites.
National Need
Because current FCPs on the market require enormous trucks to haul the hydraulically driven probe and because the typical probe is too large (>3”) to test for an archeological site without destroying large chunks of the site in the process, Russo proposed that a smaller version of the FCP, powered by pushing or pounding the probe through the soil matrix could be developed to test for archeological soils. The probe footprint would be less than ½” (1.3 cm) diameter, thus reducing the amount of soil disturbance associated with the larger hydraulic 3” (8cm) probe and typical 12” (30 cm) shovel tests. The FCP could aid in the discovery and bounding of certain types of archeological sites, i.e., midden sites, without producing a lot of shovel test holes or artifacts, the latter of which are currently overwhelming the limited storage capabilities of curation facilities in the U.S.
This “curation crisis,” in part, has arisen over the last half century from the enforcement of the National Historic Preservation ACT (NHPA) Sections 110 and 106 and other government requirements to find and bound the extent of archeological sites. In the recovery of excessive numbers of artifacts and ecofacts resulting from these legally mandated surveys, artifacts languish in museums and other curations facilities awaiting expensive analysis, cataloging, and curation that never seem to come. The proposed FCP would help minimize the number of archeological objects that require expensive processing and storage by producing proxy data for artifacts by identifying the organic midden soils in which they are found at some sites instead of recovering the artifacts themselves. With the use of the FCP in place of shovel testing, the number of artifacts typically associated with large-scale survey recovery could be reduced, while at the same time the extent of a midden site could be demarcated.
The FCP could also be used to mitigate sites in advance of sea level changes. Due to rising sea levels, many coastal and riverine archeological sites are being submerged and lost before archeologists can map and investigate them. Coastal shell middens and mounds are particularly prone to exclusion from intensive survey efforts, in part, because agencies are often not required to excavate in areas of standing or high groundwater where these sites are typically located. Technological tools (e.g., GPR, magnetic resistivity, gradiometery, LiDAR) that could help map site and feature locations in lieu of excavations are also of limited use in these environments due to either the sites’ inaccessibility and/or the remote sensing technologies’ inabilities to operate in fresh- or salt-water-saturated or submerged soils. Russo proposed that the FCP would be a technology that could overcome or reduce the logistic and technological restrictions of near-coast midden sites and could aid in the identification, recordation and even preservation of these marginally recorded sites.
Project Proposal
In 2013 Russo submitted the proposal entitled “Cone and Friction Cone Penetrometer Applications to Archeological Organic Midden Deposits” to NCPTT. He identified the research priorities as adapting an inexpensive and portable technology to measure resistance and other soil characteristics to help archeologists determine the horizontal and vertical extents of midden soils while minimizing excavation. He suggested that use of the minimally invasive tool would reduce the number of artifacts recovered and the concomitant need for their curation when performing Section 110 and 106 level surveys and other tasks requiring the identification and mapping of shell and other organic middens. He noted that if successful, shell and other middens susceptible to coastal erosion could be more quickly found and bounded in mandated surveys using the proposed FCP.
Although through the course of testing prototypes, the partnering Department of Mechanical Engineering at FSU would frequently change the main features of the FCP, initially Russo proposed to adapt an off-the-shelf hand operated CP. The Dynamic Cone Penetrometer Data Acquisition System by Vertex measured only soil resistance. Russo propose to turn it into an FCP by adding a friction sleeve. As Russo envisioned the prototype, the FCP would be equipped with a laser depth gauge, Bluetooth system, and data logger to speed up recording. The speed would make the tool economically efficient for large-scale mapping in logistically difficult terrains.
Project Science
In his own work, Russo had for over 20 years been systematically pushing probes up to 4 meters in length to map the depth and distribution of subsurface archeological shell middens and other mounded deposits (e.g., Russo et al. 2002). When he trained his colleagues in the probing technique, however, he discovered that not all could determine where shell began and ended in the vertical column of the probing rod. Some could not “feel” shell at all if the deposits were not dense. This failure in replicating measures of shell depth among probe practitioners resulted in Russo searching for a more objective and replicative probe. He first looked at off-the-shelf cone penetrometers (CPs) that measured only soil resistance as a possible alternative.
In CPs, Cone Index (CI) is the measure of force required to push a cone point through soil. The amount of force correlates with soil resistance which may be linked to a number of causes including the chemical and granular make-up of the soil or, for the surface soils that archeologists most commonly deal with, the amount of compaction. If a soil is disturbed, dug up and redeposited, the amount of compaction may be less than that of the undisturbed soil surrounding it. For road construction, a CP may be used to test the suitability of soil for supporting the road and the CI is usually compared to a known standard. For archeologists, the amount of compaction/disturbance is typically inferred from the CI when compared to a control, undisturbed sample of the same soil type, usually nearby.
An as yet untested application of the CP in archeology is the identification of differential subsurface resistance due to factors other than soil compaction. Because archeological objects (e.g., pots, bricks, shell) are more resistant than even the most compacted soils, the objects should yield indices of force that differentiate them from the surrounding soil matrices. While using CPs to locate widely dispersed objects such as potsherds or lithic debitage would be like looking for needles in haystacks, identifying the vertical and horizontal extent of dense deposits of objects such as shell or organic midden soils could be quite effective with CPs. CP recordings recovered from systematically placed points across known or unsurveyed areas would allow the location, depth, and thickness of those shell deposits or organic soils to provide a detailed three dimensional map of the middens (e.g., see midden density maps in Russo et al. 2002; Russo et al. 2011).
As mentioned, different soils are classified in part by differences in their grain or particle sizes, with, for example, clays and silts consisting of finer particles while sands and humic layers consist of coarser grains. Because fine-grained sediments have more surface area than coarse- grained sediments, they are more cohesive. That is, they result in a greater amount of friction when a probe is pushed through them because more grain than air rubs against the rod. (Anyone who has worked in soils intuitively knows that clay is harder to dig than sand). This variability in friction among differently-textured soils can be measured with a cohesion sleeve placed above the tip of the cone penetrometer (Kianirad 2011:26–27; Rooney et al. 2001). The addition of the friction sleeve turns the CP into an FCP. The proposed FCP was intended to distinguish organic archeological middens soils (typically composed of coarse sands with coarser-grained organic inclusions consisting in part of decaying plant and animal remains as well as objects of human manufacture) from finer-grained, undisturbed minerals soils such as finer-textured sands, silts, and clays.
While no hand-held portable FCPs existed at the time of the proposal, two types of portable CPs were described in the literature: static and dynamic. Portable static CPs rely on a steady push by its operator into the soil at a specified rate. Static CPs have been criticized due to inter- and intra- operator variability in the required “steady” application of force (Herrick and Jones 2002; Jones and Kunze 2004:3). The claim is that no one, let alone two people can push at the same reliable rate from top to bottom of a probing. However, some claim that with practice, consistent results, assumed to have been derived from consistent application of force can be obtained (Trinkley 1999:8).
In contrast to the static CP where consistency of force may be hard to maintain, the dynamic CP or DCP relies on a given weight being dropped from a known height to obtain a reliable measure of applied force. While the static model is potentially more portable (i.e., fewer parts, less weight to carry around) and faster (Kees 2005:9), Russo proposed to build a dynamic model to assure replicability and quantification of tests. Although somewhat counterintuitive, personal experiences with probes suggested to Russo that due to increased friction, sand required more force than shell to push a probe through. Shell requires quick, momentary impulse force to pierce the shell, but less force to pierce the loosely packed soil and air between shells. That is, to get through shell with a probe, one must lift the probe up and slam it down with a lot of force. Once an obstructive piece of shell is pierced by the probe tip, however, little force may be needed to push it through air pockets or loose soil spaces between shells. Sand, silt and clay-based midden soils, on the other hand, due to less air and more grains would need more impact force overall, meaning more lifts and drops. Part of the proposed laboratory testing goals was to determine the best dynamic weight protocols to use to pound the probe through all types of soil. Russo anticipated that shell deposits would record graphically and numerically as peaks and valleys of greater and smaller resistances as shell and air pockets were encountered alternately. Non-shell bearing soils, he reasoned, would more likely record as an increasing but steady graph line as depth increased. He anticipated that data loggers and strain-gauges to accommodate the wider- ranging force variables would need to be obtained or manufactured.
Developing the Prototypes
One of the initial goals of the project would be to test and determine the limits of stress that the cohesion sleeve and strain gauges could handle. The FSU engineering teams constructing the FCP might need to use stouter sleeves or gauges to facilitate its application to severe archeological conditions that contained dense amounts of hard objects like shell. The overall goal would be to develop an FCP that measures both shell and other organic midden deposits by recording the cone index obtained by the CP, the friction recorded by the FCP, and the ratio of the two in order to differentiate shell middens and other midden deposits lacking shell from background non-archeological soils.
Despite all the inspiration shell middens provided for the initial idea of the FCP, ultimately the idea of testing shell middens would be abandoned. Once into the project, the engineering teams and Russo worried that repeated impacts with shell would break the fragile friction sleeves. In addition, large weights would need to be carried to break shell, thus reducing the idea that the instrument would be lightweight and portable. Difficulties in adjusting weights needed to break through hard shell or other objects had been identified and a research model of an FCP (cf., Kianirad 2011:27). With these caveats in mind, the organic middens that would be tested and to which the FCP would be designed for were limited to organic soils with little or no shell or that contained shell deposits that were not dense.
SCDP Team 16 FCP Prototype
The project began with the award of the NCPTT grant for $25,000 to SEAC in 2013. In June and July, NCPTT and SEAC signed an agreement (Appendix A) outlining the goals of the project, the budget, the tentative schedule, a list of outreach products related to the FCP, and the deliverables, which include this final report. Prior to the award Russo had contacted Dr. Chiang Shih, head of Florida State University’s Mechanical Engineering Department to develop the FCP prototype and testing through his student Senior Design Capstone Program. The program is run through two semesters of the final year of graduating engineering students who have mastered all the skills within the traditional engineering disciplines.
“The purpose of the Senior Design Project is to pull (the engineering disciplines) all together and apply them towards the design and implementation of a ‘product’, and to afford the students an opportunity to experience team-based design under conditions that closely resemble those that will be encountered in industry. Students must develop and sharpen skills in team organization, time management, self-discipline, and technical writing, in order to be successful in this course. An important goal of this course is to expose students to a ‘hands-on’ experience in which they have to specify, design, and produce a full-system beginning from relatively ill-posed needs as stated by a ‘customer’. This objective has to be accomplished while working as a team, and under time pressure.” (https://eng.fsu.edu/me/senior_design/)
Russo chose to partner with Shih through Capstone because of Shih’s enthusiasm for the project and the close proximity of his FSU facilities and students (just two buildings down from the SEAC facilities). Alternate professional mechanical engineering firms were approached but were not interested in the project due to low probability of high economic returns. In June of 2013, Shih submitted his proposal and budget for the project (Appendix B), largely modeling it on the NCPTT/SEAC Project Agreement (Appendix A).
In the summer of 2013, Shih and Russo chose the Dynamic Cone Penetrometer Data Acquisition System for the student team to adapt into an FCP (Figure 1). Before the 2013 Capstone project began, Shih hired a student, Richard Carter to work with Russo and get background research completed on off-the-shelf cone penetrometers and other data the Capstone Team would need before they started in the fall. Carter’s report for the students entitled “Dynamic Cone Penetrometer With Friction Sleeve” was included in Russo’s 2014 Progress Report to NCPTT as Appendix 1: “Preliminary Introduction and Guide to the Friction Cone Penetrometer Project” (Appendix C).
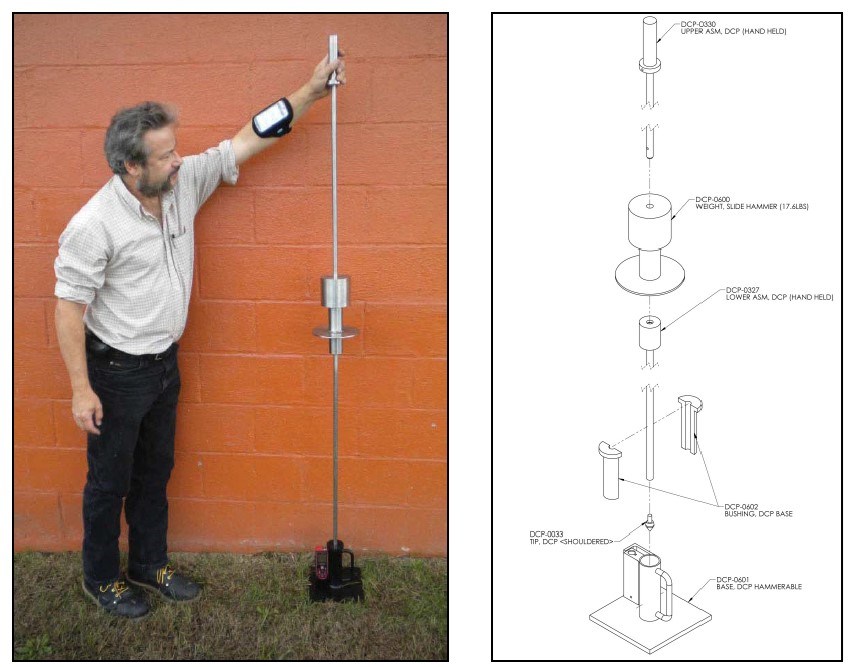
Carter suggested that a friction sleeve with its own load cell could be added behind the already existing cone tip load cell to produce the prototype FCP. His report was submitted to the student team in the fall of 2013 for consideration.
One other partner needed for the project was a soil scientist. For that role, Russo contacted Dr. Lynn Coultas, retired Soils Science Professor at Florida Agricultural and Mechanical University (FAMU). Russo took Coultas to the Byrd Hammock archeological site near Tallahassee, Florida, which was to serve as the testing ground for the prototype FCP once it was in working order.
Coultas’ first project was to analyze seven five gallon samples of different organic and mineral soil samples that the students could use in the lab to test the effectiveness of their prototype in distinguishing among soil types. The goal was to distinguish midden soil from various other soils like clay, sand and silt, and six types of soil were chosen for comparison to a sample of organic midden soil from Byrd Hammock. Coultas demonstrated that in terms of grain size, amount of organic matter, water and sand content, the Byrd Hammock midden soil was indeed significantly different from all other soils. With the exception of cow manure, the midden contained far more organic matter than the other soils, more silt, and a higher capacity for holding water. Coultas’s report was included in Russo’s 2014 progress report (Appendix C) as Appendix II. Unfortunately, as the reader shall see, a prototype never reached a point where field testing could be effectively completed, and as such Coultas’ services were not further called upon.
The student design team first met on September 20, 2013, as team number 16 of the Student Capstone Design Program (SCDP), the FSU Engineering one-year program designed to introduce seniors to the real-life industrial engineering world and help them transition into professional careers (Luongo et al. 2007). For the FCP project, SCDP Team 16 consisted of 6 senior FSU Mechanical and Electrical engineering students (Arias Austin, Alexander Beckers, Joseph Collar, Kevin George, Shayla George, and Roberto Toledano) chosen by FSU faculty with SEAC (Russo) serving as the client. To initiate the SCDP project, students met with Russo who provided background data, references on cone and friction cone penetrometers, and his goal and ideas on the adaption of the Vertex DCP into an archeological instrument capable of distinguishing organic midden deposits from other soil types.
A month later the team met with Shih and Russo with their initial design incomplete. They could not decide whether to use load cells or string gauges to measure resistance and cohesion or to use one or two of the measuring instruments. On December 3, 2013, the team again met with Shih and Russo still unsure of their final design. They did not know if they could accommodate a wireless system to record readings or how to protect the electronics in the friction sleeve from water intrusion if placed into wet soils. On February 20, 2014, they met again having decided to go with load cells rather than string gauges. But they were still not sure how strong the cells should be. The stronger the load cells are in resisting impacts from hammering into the soil and hitting hard objects, the less sensitive they are to measuring small differences in soil behaviors.
Russo recommended to the team to get the smallest, most sensitive load cell they could purchase, while suggesting at the same time that they were behind schedule.
On April 17, Team 16 presented their results in a student forum designed to display the final products of all Capstone team efforts. However, at this showing Team 16 failed to produce a final product. Shih and Russo concluded there had been communication and personality problems between the electrical and mechanical engineering students in Team 16 with no one taking the lead to bring the project in on time. On April 29, Shih met with the team and failed all mechanical engineering students, making them take an extra summer semester to produce a testable prototype. Shih then hired an advanced graduate student, Alexander Beckers during the summer to work on a new design with the team.
On June 10, 2014, Russo met with Beckers and Shih. Beckers presented a Power Point discussion showing a new design for the friction sleeve to keep out water and soil from the load cells and contrasting it to the old design that was susceptible to leaks. He also presented data from his new prototype (Figure 2) that seemed to be able to distinguish differences among soil types. The results of Beckers’ Power Point presentation were called “Friction Cone Penetrometer Design Update” and were originally included as Appendix IVof Russo’s (2014) progress report to NCPTT. That paper is included in this report as Appendix C. Shih directed the student to complete the production of a prototype to be tested in the field at an archeological midden site by July. That deadline was not met and Shih, even though there was no agreement obligation to continue working on the project, suggested to Russo that he would assign a new SCDP team to the project at no extra cost to NPS to try again to produce a successful prototype in the fall of 2015.
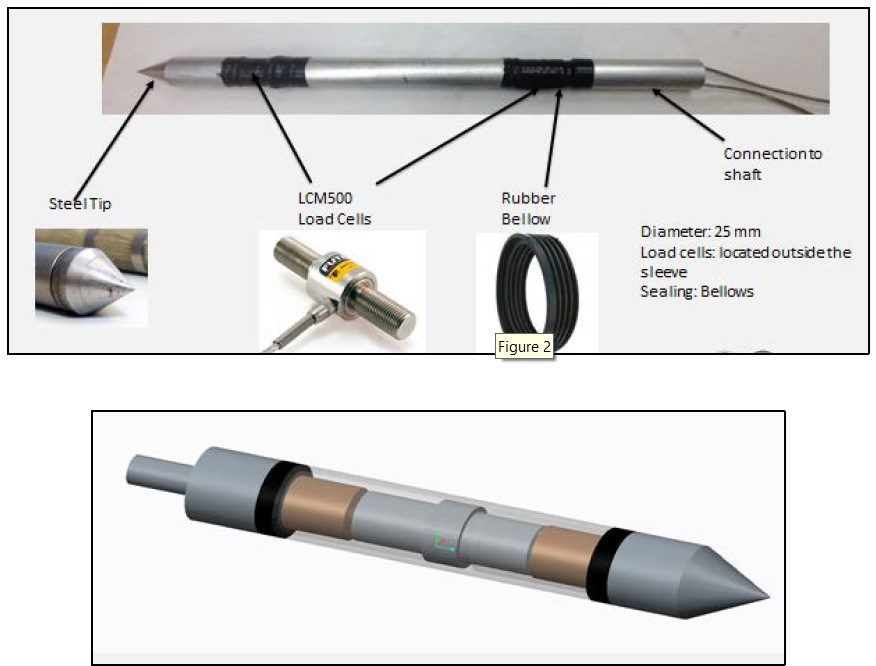
SCDP Team 18 FCP Prototype
On August 25, 2014, a new SCDP Team, #18, was assigned to assess Beckers’ prototype and redesign the FCP to overcome some of the problems with it. The team included four mechanical and three electrical engineering senior students including Carren Brown, Deneuve Brutus, Peter Hetteman, Sean Kane, Natalie Marini, Mitchell Robinson, and Maritza Whittaker. In September, the new team and Russo drove to the Byrd Hammock archeological site to test Becker’s prototype in real-life midden soils. As noted in their final report (Appendix D), the team discovered that the prototype at that time was not very portable. It required three electronic units to record data, a folding table to accommodate them and a generator to run the probe, as well as the two weights to hammer the probe into the ground and the probe itself which was not wireless as designed, but which was wired somewhat inelegantly to the recording equipment on the table. The testing consisted of one positive test that did record potentially readable data until a wire broke within the friction sleeve housing. In-field rewiring attempts proved unsuccessful and the testing was abandoned.
The field excursion, nonetheless, was successful to the extent that it taught the new team early on the problems associated with the prototype. They concluded that the probe diameter was too large with the shaft material too weak to withstand repeated hammerings (they noted cracks in the shaft). In addition, the electronics needed to be wireless and better protected from soil intrusion. The portability of the instrument also needed to be improved for use by one or two operators. From their field observations, they determined their design goals would be to make the instrument:
- lighter, less than 50 pounds
- smaller in diameter by using smaller load cells
- operable by one or two people
- better sealed/protected from soil intrusion on the electronics
- shaft more resistant to repetitive compressive forces
To fix these problems, Team 18 came up with four possible designs. The first two were similar to the prototype in which they had used two unconnected load cells to measure cohesion and resistance separately (Figure 3). The differences being that the new designs used smaller load cells to reduce the diameter of the probe rod. Design A placed the load cells near the cone tip, theoretically protecting the electronics from environmental damage with better housing, while Design B protected the cells and wiring by moving them nearer the top of the probe where they would not penetrate the soil at all. The team noted potential problems moving the electronics and cells up the rod, namely that they were unsure if the resistance and cohesion loads encountered near the cone tip could be accurately transferred to cells near the top of the rod. Designs C and D differed from A and B only in that they used strain gauges instead of load cells, with Design C placing the strain gauge vertically along the shaft and Design D, horizontally (Figure 3).
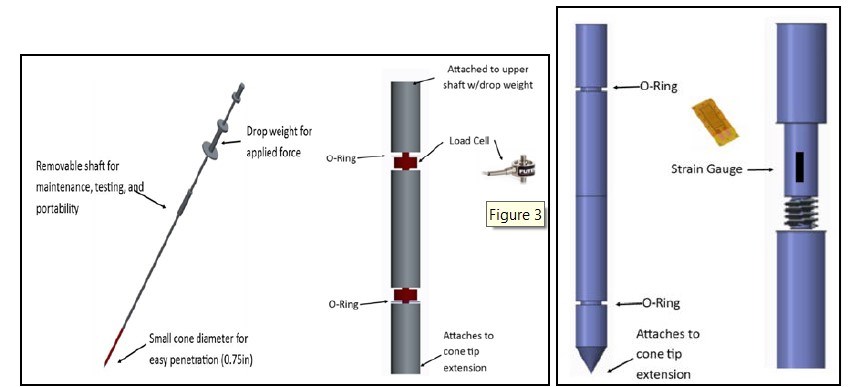
While the Team 18 students favored Design D, upon meeting with Shih and Russo they were persuaded that strain gauges were too sensitive for repetitive pounding and soil intrusion and would require frequent re-calibration. Ultimately, the team took on the ideas behind Design B, moving the cells and electronics farther up the rod, dividing the instrument into three components with the housing of the load cells being placed between the drop weight platform and the cone tip and rod (Figure 4).

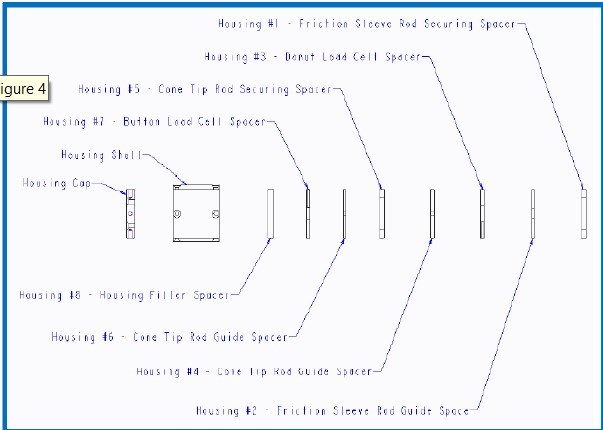
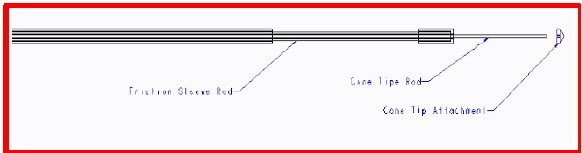
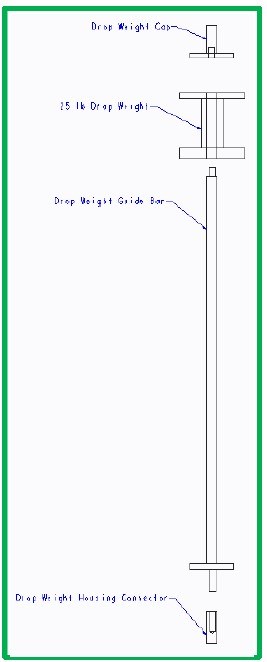
Poor time management resulted in a delayed production of the new prototype. As with Team 16, Team 18 was late in deciding on their final design and failed to accurately gauge how long it would take to purchase and manufacture parts for the instrument’s production. As of April 10, 2015 when they submitted their final report to Shih for review, a prototype had been built and tested in the lab but was not sufficiently portable to be tested in the field. The team did record friction coefficient readings on two samples of soils in the lab, but did not compare them to midden soil samples. So whether midden soils could be distinguished from natural soils with the FCP remained an open question.
James Pace Design and Production
With Team 18 having made some success in developing their new design, with FSU funds Shih hired James Pace, a mechanical engineering student to firm up a workable prototype. Pace began work in the summer of 2015, submitting a report on his progress in December 2016 (Appendix E). He stated that the major difference between his and Team 18’s design was that his penetrometer was made mostly of aluminum, as opposed to steel, resulting in a much lighter instrument. Also his penetrometer held a more refined sensor casing to house and protect the load cells and its data acquisition unit. He abandoned the Bluetooth technology that measured depth of the cone penetration into the soil that came with the original Vertex cone penetrometer, because it could not “speak” to any of the previous Teams’ data recording systems due to proprietary and technological limitations.
The result of Pace’s initial work was a series of tests on the laboratory soils that Coultas had classified as sands with variable amounts of silts, clays, and organic matter mixed in. The first tests indicated that the load sensors were indeed working but there were problems with the voltage. Increasing the voltage in the second test fixed the problem, but caused others. For one, the maximum force readings exceeded Pace’s scale of measure. An accurate maximum force reading was needed to develop a standardized cohesion coefficient for comparison among soils. Two, the resistance force graph was out of synch with the cohesion force graph (Figure 5). That is, the peaks of friction lagged slightly behind the peaks of resistance. And three, he suspected that vibration from the dropped weights was being recorded as “background noise,” throwing off the synchronization and giving false readings. To fix the problems, Pace optimized the voltage readings on his recorder, reduced the height from which the weight was dropped to push the rod through soil, and placed an additional bushing in the casing holding the resistance load cell—all in the hope to reduce vibrations.
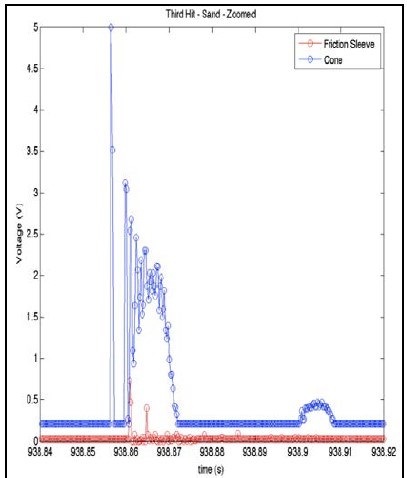
These changes produced cohesion coefficients for the laboratory soil samples with data indicating that the behavioral differences in the varied soils were potentially distinguishable. Midden soils could indeed be distinguished from other types of soils. Unfortunately, though these fixes had reduced the asynchronous graph lines somewhat, it had increased ten-fold the number of drops it took to move the probe short distances through the soils. This made the instrument very inefficient.
In late 2016, after Pace participated in discussions with Shih and Russo, Shih and Russo agreed that no more time could be spent on the project given the limits of funding and Pace submitted his final report (Appendix F) and operations guide for the FCP (Appendix G). Pace’s two major contributions to the project were: one, the development of a wireless system operable from any cell phone that could link the force sensor data and the Vertex laser range finder data to produce graphic displays; and two, the testing of a series of laboratory soil samples that indicated, despite problems with reverberation, that natural soil types could potentially be distinguished from midden soils. Accurate readings without the vibrations induced by the dropped weight, however, would be critical to the calculation of cohesion coefficients necessary to distinguish among soils types.
In his final report (Appendix F), Pace suggested that his initial tests on the laboratory soils demonstrated that differences in soils could be distinguished with cohesion coefficients provided by his FCP prototype, but that he could not repeat those readings in subsequent tests. He attributed this unrepeatabilty to operator error or design flaws, but was unsure which or how these might be corrected. The background noise that interfered with clean readings of the maximum force impulses recorded for each drop of the weight continued to be a puzzle. Pace suggested they likely resulted from the visually imperceptible microscopic bouncing of the weight on the receiving platform like dropping a basketball on the floor and having repeatedly smaller bounces until the ball came to a halt. Pace saw this problem as a mechanical problem that should be fixable given enough time and money.
Russo and Shih Conclusions
Russo agreed with Pace that many of the problems with the current design of the FCP could be overcome with more time and creative thinking. But major problems in the design may not be surmountable. That is, the use of the dynamic type of cone penetrometer as the model for the FCP may obviate its portability. As stated, the difference between a static and dynamic penetrometer is that the static is pushed through the soil with the same measurable, continual force. This is usually achieved with hydraulic power. But the dynamic penetrometer uses dropped weights to push the probe rod through the soil. With the idea that the FCP should be sufficiently portable for one operator to carry from probe location to probe location quickly and with little effort, the varied designs were perforce limited to using the dynamic model rather than the static since a lone individual could not carry on their own a large or complex hydraulic system.
The various designs for the FCP all centered around the idea of an individual carrying a 10 or 25 pound weight along with the probe itself, and possibly a stand to make sure the probe maintained verticality throughout the pounding. These requirements of heavy weights along with the long probe rod, recording equipment, and possibly a probe rod stand essentially render the FCP un- portable for one operator to handle.
In theory, the idea was that the FCP would take less than ten minutes to obtain a reading. At that rate, the operator could produce nearly 50 readings in a day. Twenty-five is about the maximum number of shovel tests that an efficient archeologist can dig in a day, and Russo reckoned that the FCP would need to be at least twice as efficient to make it an attractive alternative to shovel testing in the field. Although none of the FSU designers tested the speed at which a reading could be obtained in the field by one operator, based on observations in the lab, the process was slower than anticipated. Once set up to take a reading, which took maybe 20 minutes, subsequent readings in the same location could be repeated in relatively rapid order, maybe in as little as a couple minutes. But in the field, after each reading, the entire FCP machinery would have to be collected and moved to another location and set up again. This collection and setup might take as much as 30 minutes for each move. Assuming the FCP could give accurate readings, unless the efficiency of moving and taking readings could be improved, archeologists could dig shovel tests far faster than taking FCP readings.
Shih has noted the problems efficiency (as well as the technological problems of vibrations) are all linked to the teams’ choice of going with the dynamic cone penetrometer (DCP) rather than the static cone penetrometer (SCP) as the instrument to be adapted for the FCP. He suggests that the FCP could be converted to a steady thrust, static model. As stated earlier, one of the main reasons for using DCP relative to the SCP is that it can produce relatively consistent measurements since it penetrating force is independent of human operator subjectivity. As discussed by Pace, while dropping given weights at known heights can generate repeatable impact forces on the DCP, other complications are introduced to make the DCP data difficult to interpret:
- The short duration of the impact force (order of milliseconds) requires high speed data collection and a high sampling frequency (order of kHz) is needed to capture the impulse forces (both impact and the frictional forces) generated by the impact. In addition, higher uncertainties are introduced due to the limited data that can be obtained during the short impact. All told, these limitation restricted to the DCP require advanced data acquisition system and sophisticated programming schemes that were beyond the reach of the team’s time and funding.
- As the DCP model reacts to the sudden impact it bounces up and down generating oscillatory data pattern further contaminates the data.
- The dynamic response of the impact force is quite different from that from the frictional force since the former is more sensitive to the soil rigidity as compared to the latter. Without comprehensive characterization of the soil dynamics, the DCP results might not be reliable.
- The high impulse force due to the sudden impact makes the selection of the optimal load cell difficult. A more robust cell is needed to make sure that the impact forces do not exceed the operating limit of the cell; however, the measurement resolution is compromised as a result. Therefore, there are still many issues to be resolved for the DCP system to function effectively.
The difficulties encountered in the teams’ production of an operative FCP based on the DCP model, turns our attention back to the SCP model as a potentially workable alternative. As noted, the SCP is either pushed through soils with direct human muscle action, or in larger models, is pushed through soils with hydraulic pressure. The teams initially abandoned the SCP type of penetrometer as a model for the FC due to the fact that human muscle action is not measurable or repeatable and larger hydraulic systems that employ impulse thrust in measurable ways were not susceptible to the required miniaturization to make the FCP portable by one person. Shin, however, suggests another non-manual powered FCP based on the CSP model might work.
Instead of requiring a human operator to push down on the CP manually, a simple mechanical system for the operation could be designed to push the cone, friction sleeve and rod. A station to support a cranking mechanism connecting to the FCP rod would allow the CP to penetrate freely into the ground through a guiding channel. The mechanism would designed with a rotary crank that would allow the operator to repeat the motion more at the same, repeatable rate (e.g., 1 rotation per 5 seconds), as opposed to the manual push down motion which varies in force relative to traits of soils, strength and height of the operator, and fatigue variance. Alternatively, it could be operating by a computer-interface motor with a precisely-controlled speed. In either case, calculations and experimentation would be needed to calculate the size of the mechanical gears or power of the interface motor needed to penetrate most midden soils and their surrounding natural soil matrices. The penetration depth of the static FCP could be obtained accurately through the motion of the craning mechanism thus eliminating the use of laser range finder. Such a static system could produce more consistent data while avoiding many complications introduced by following the DCP model of dropped weights.
Had the teams realized the problems that would be encountered following the DCP model instead of the SCP model, they may have had more success developing an alternate thrust mechanism that could replace hydraulics. That being said, perhaps the best data to come out of the project are those identifying the DCP model for the FCP as highly problematic. The next productive data to be rendered are Pace’s measures of soils through cohesive coefficients. These suggest that midden soils are indeed potentially distinguishable by the measure of two soil behaviors, resistance and cohesion. With this knowledge we hope that future engineers may be able to take the results of our designs and tests to perfect a portable FCP that can aid archeologists in defining the locations of midden deposits without the need to excavate large volumes of soil.
Bibliography
- Hailey, Tommy and Elizabeth Ball 2006 The Sandiferd Cemetery Revisited: Locating Unmarked Burials Using a Digital Soil Compaction Meter. Paper presented at the 71st Annual Society for American Archaeology meetings, San Juan.
- Herrick, Jeffrey E. and Tim L. Jones 2002 A Dynamic Cone Penetrometer for Measuring Soil Penetration Resistance. Soil Science Society of America Journal 66:1320–1324.
- Jones, Dave and Matt Kunze 2004 Guide to Sampling Soil Compaction Using Hand-held Soil Penetrometers. CEMML TPS 04-1, Colorado State University, Fort Collins. Available at: http://www.cemml.colostate.edu/assets/pdf/ TPS_04-1_Sampling_Compaction.pdf
- Kees, Gary 2005 Hand-held Electronic Cone Penetrometers for Measuring Soil Strength. 2E22E60 – Soil Strength Tester. USDA Forest Service, Technology and Development Program, Missoula. Available at: http://www.fs.fed.us/t-d/pubs/pdfpubs/pdf05242837/pdf05242837dpi300.pdf
- Kianirad, Ehsan 2011 Development and Testing of a Portable In-Situ Near-Surface Soil Characterization System. PhD. Dissertation, Northeastern University, Boston.
- Kratt, Hank, Charles L. Coultas, and Michael Russo 2008 The Lewis Camp Mound: An Example of a Petrocalcic Horizon in Jefferson County, Florida. Paper presented at the Joint Meeting of The Geological Society of America, Soil Science Society of America, American Society of Agronomy, Crop Science Society of America, Gulf Coast Association of Geological Societies with the Gulf Coast Section of SEPM, Houston.
- Luongo, Cesar A., Chiang Shih, James W. Surges, David C Bogle, R.A. Wright 2007 Senior Design Projects in Mechanical Engineering – Active Involvement of Industry Partners and Advisory Council. Copy available at the Department of Mechanical Engineering Florida A&M and Florida State University, Tallahassee.
- Moore, Clarence B. 1918 The Northwestern Florida Coast Revisited. Journal of the Academy of Natural Sciences of Philadelphia 16:513-581.
- Rogers, J. David 2006 Subsurface Exploration Using the Standard Penetration Test and the Cone Penetrometer Test. Environmental and Engineering Geoscience Vol. 12, No. 2, pp161–179.
- Rooney, Dan, John Norman, and Mark Stelford 2001 Mapping Soils with a Multiple-Sensor Penetrometer. Paper presented at the Wisconsin Crop Management Conference. Also see: www.soils.wisc.edu/extension/wcmc/proceedings01/Rooney- etal.PDF
- Russo, Michael 2004 Measuring Shell Rings for Social Inequality. In Signs of Power: The Rise of Cultural Complexity in the Southeast, Jon Gibson and Phillip Carr, eds., pp. 26–70. U. of Alabama Press, Tuscaloosa.
- 2006 Archaic Shell Rings of the Southeast U.S. National Historic Landmark Theme Study: www.nps.gov/nhl/themes/Archaic%20Shell%20Rings.pdf
- Russo, Michael, Carla S. Hadden, and Craig Dengel 2009 Archaeological Investigations of Mounds and Ring Middens at Hare Hammock: Tyndall Air Force Base. Submitted by the Southeast Archeological Center to Tyndall AFB. Copies available from the Southeast Archeological Center.
- Russo, Michael, Craig Dengel, and Jeffrey Shanks 2012 Public Plazas as Mediating Loci at Weeden Island Coastal Settlements. In Ceremonial Spheres of the Eastern Woodlands, an invited symposium at the Society for American Archaeology 77th Annual Meetings, Memphis.
- Russo, Michael, Craig Dengel, and Jeffrey Shanks 2014 Finding the non-Quotidian at Putative Quotidian Swift Creek and Weeden Island Ring Middens in Northwest Coastal Florida Coastal Settlements. In New Histories of Pre-Columbian Florida, Neill J. Wallis and Asa R. Randall, editors, pp. 121–142. University Presses of Florida, Gainesville.
- Russo, Michael, Craig Dengel, Jeffrey Shanks, and Thadra Stanton 2011 Baker’s and Strange’s Mounds and Middens: Woodland Occupations on Tyndall Air Force Base. Southeast Archeological Center, National Park Service, Tallahassee. Submitted to Tyndall Air Force Base, Panama City, Florida. Copies available from the Southeast Archeological Center.
- Russo, Michael, Gregory Heide, and Vicki Rolland 2002 The Guana Shell Ring, Historic Preservation Grant No. F0126. Submitted to the Florida Department of State, Division of Historic Resources, Tallahassee. Copies available from the Southeast Archeological Center.
- Shanks, Jeffrey, Michael Russo, and Craig Dengel 2012 Combining Low and High Technologies to Overcome Phased Survey Limitations. In Dig it! The Intersections between Excavation Methods and Recording Systems, an invited symposium at the Society for American Archaeology 77th Annual Meetings, Memphis.
- Trinkley, Michael 1999 Penetrometer Survey of Settler’s Cemetery, Charlotte, Mecklenburg County, North Carolina. Chicora Research Contribution 273, Chicora Foundation, Inc., Columbia, South Carolina.
- 2007 Penetrometer Study of the Macedonia Baptist Church, Cherokee County, South Carolina.
- Chicora Research Contribution 465, Chicora Foundation, Inc., Columbia, South Carolina.
- Trinkley, Michael, and Nicole Southerland 2007 Penetrometer Survey of the Nance Property–Moss Family Cemetery, Stanley County, North Carolina. Chicora Research Contribution 464, Chicora Foundation, Inc., Columbia, South Carolina.
- USDA, 2012 Cone Penetrometer, Chapter 11. In Part 631 Geology, National Engineering Handbook. Available at: http://policy.nrcs.usda.gov/OpenNonWebContent.aspx?content=31850.wba
- Zeidler, James A., editor 2005 Military Impacts and Archaeological Site Mitigation Methods at the Firebreak Site (41CV595) Fort Hood Texas. Publication TPS-04-08, Center for Environmental Management of Military Lands, Colorado State University, Ft. Collins.