Part of a series of articles titled Reckoning with a Warming Climate.
Article
What Happens When Northern Oceans Get Too Warm
RESEARCH REVIEW
Heather Coletti, Ecologist, Southwest Alaska Network, National Park ServiceNina Chambers, Science Communicator, Alaska Region and Inventory & Monitoring Division, National Park Service
Jamie Womble, Program Manager, Southeast Alaska Network, National Park Service
Christine Gabriele, Wildlife Biologist, Glacier Bay National Park and Preserve, National Park Service
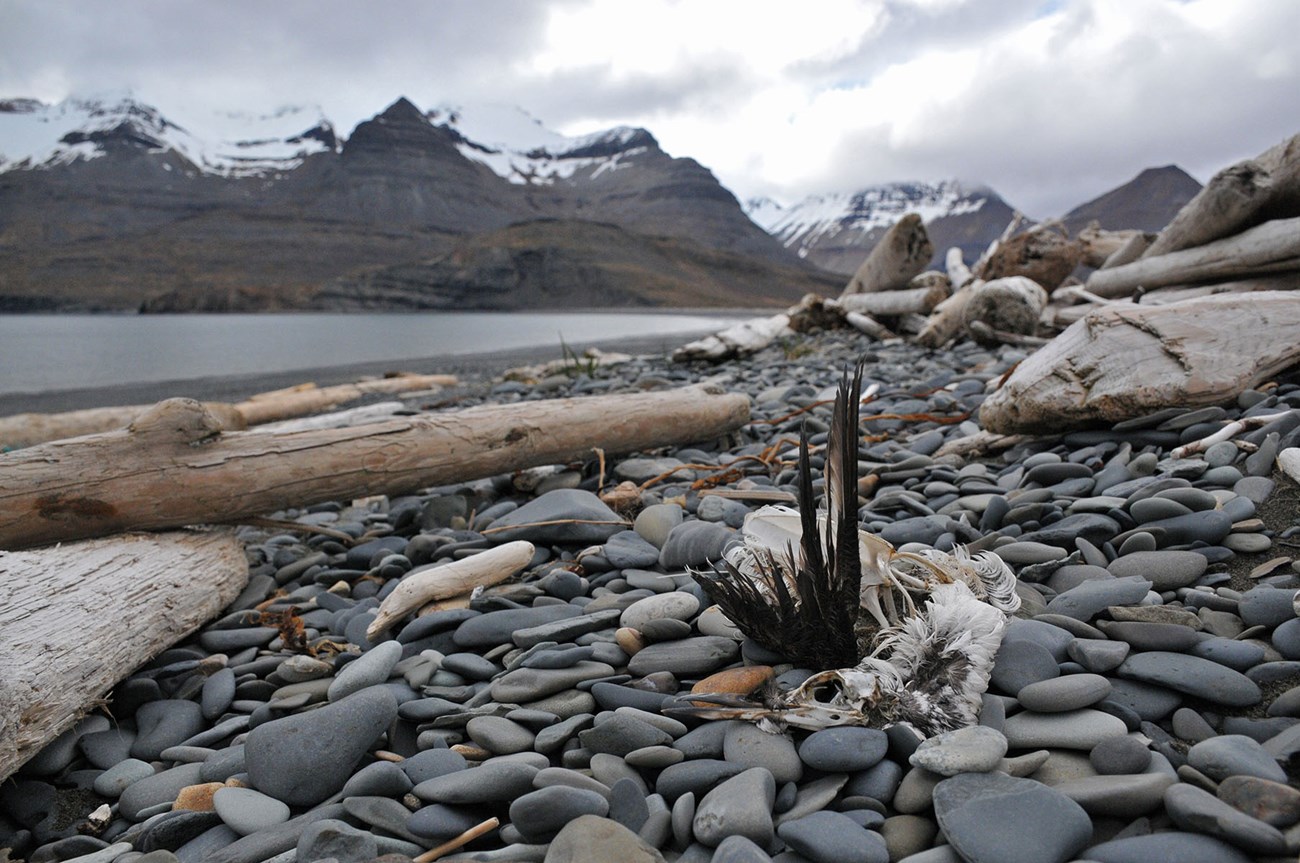
USGS/SARAH SCHOEN
Alaska is surrounded on most of its border by oceans, with an estimated length of coastline likely exceeding 33,904 miles. These oceans have been integral to the lifeways of people for hundreds of generations. Ecologically, Alaska’s productive northern oceans and seas provide abundant and commercially important fisheries and habitat for marine mammals (whales, polar bears, seals), seabirds, shorebirds, and many other species. In addition, much of Alaska’s weather, exceeding over a thousand miles inland, is influenced by the ocean, including the severity of storm fronts, precipitation patterns, and rapid temperature flux.
Marine Heatwaves and How They Impact Ecosystem Components
Marine heatwaves are a consequence of changing ocean conditions and have increased globally in recent years (Gruber et al. 2021). They are abrupt, extreme events that are likely to occur more frequently and across larger areas than in the past due to a warming climate (Frölicher et al. 2018). In 2013, researchers noticed ocean temperatures were rising across the North Pacific Ocean, which represented the onset of a marine heatwave. The Pacific Marine Heatwave (PMH) persisted for more than three years and had profound and lasting effects on everything from phytoplankton to whale watching in Hawaii, to trawl fisheries in northern Alaska. It was the most impactful heatwave owing to its spatial extent, magnitude, and duration. For example, persistent high ocean temperatures of 5-11 degrees Fahrenheit (3-6 degrees Celsius) above average were recorded from Southern California to the Gulf of Alaska (Piatt et al. 2020, Bond et al. 2015).
Thanks to a suite of studies, informed by long-term monitoring and research conducted by scientists and resource managers at Alaska parks in collaboration with university and agency partners, we now have a better understanding of how marine food webs, fish, marine mammals, and seabirds responded to the profound changes during and after the heatwave. Climate projections predict more marine heatwaves, and questions persist as to how, when, and why species may (or may not) recover from these large-scale perturbations.
The Pelagic Food Web Response was Severe
When marine heatwaves occur in normally cold water off the coast of Alaska, primary productivity goes down.
Everything from small fish to seabirds to whales rely on plankton. Plankton is made up of phytoplankton (tiny plants that are the primary producers in the pelagic ecosystem) and zooplankton (small animals that can be primary consumers of phytoplankton). Zooplankton, such as copepods, were smaller and less nutritious during the heatwave. Certain species of copepods became more prevalent, and these warm-water species tended to be smaller and lipid-poor (less fatty) than the larger, lipid-rich (fattier) copepods generally found in cold water. This translates to lower-quality food for animals that feed on zooplankton (Arimitsu et al. 2021).
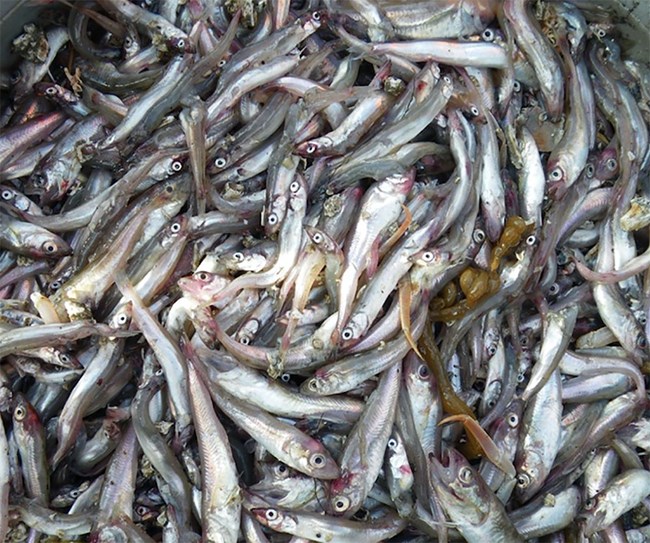
NOAA
Forage fish populations collapsed.
Small, cold-water fish that feed on plankton are collectively (and appropriately) termed forage fish. They are important food sources for larger fish and other marine animals. In normal conditions, different species of forage fish have diverse life histories resulting in variability in timing of life stages such as larvae, juveniles, and spawning. This diversity in life history strategies varies their abundance across space and time, providing a buffer against low availability to marine mammals and seabirds. In this case, the heatwave was so large that multiple forage fish species concurrently collapsed (Arimitsu et al. 2021). The result was large-scale starvation of many seabirds, marine mammals, and other fishes.
What forage fish remained were smaller and in poorer condition due to a lack of nutrients and metabolic stress.There was likely more competition for these dwindling numbers of forage fish, too, because both seabirds and larger fish needed more of the smaller forage fish for food. When the water warmed, the fish warmed with it, and a warmer fish is a more active fish—and a hungrier one. Neither pelagic fish nor seabirds could meet their metabolic needs (Arimitsu et al. 2021, Piatt et al. 2020).
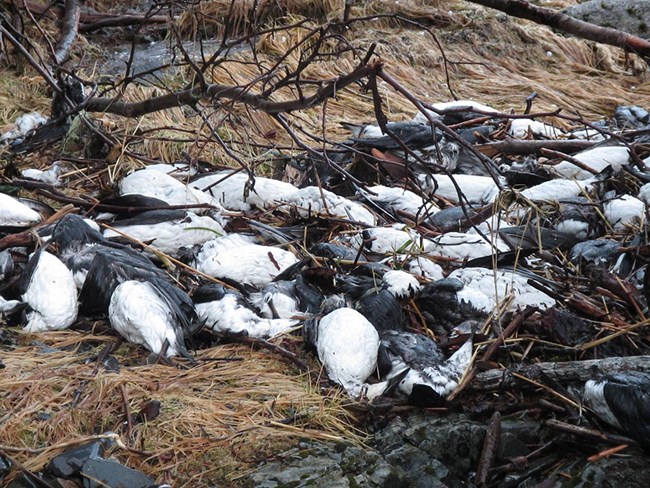
USGS/TONY DEGANGE
With little to eat and only/mostly low-quality forage available, there was a massive seabird die-off.
The 2014-2016 marine heatwave resulted in a wide-spread common murre die-off and repeated nesting failure of breeding colonies from the southern Bering Sea to the south coast of California. At least 500,000 and perhaps as many as just over 1 million seabirds, most of them common murres, died in the Gulf of Alaska and all along the west coast of the U.S. Seabird die-off events are not unusual; they occur on an irregular basis throughout the world’s oceans when food supplies are depleted or otherwise unavailable. But what made this die-off globally unprecedented was the extreme extent—the large geographic area that was impacted, how long it lasted, and the vast number of dead birds. They starved because their food source changed; prey became less available and what was available was smaller, leaner, and less nutritious than usual (Piatt et al. 2020, also see How Marine Heatwaves are Changing Ocean Ecosystems).
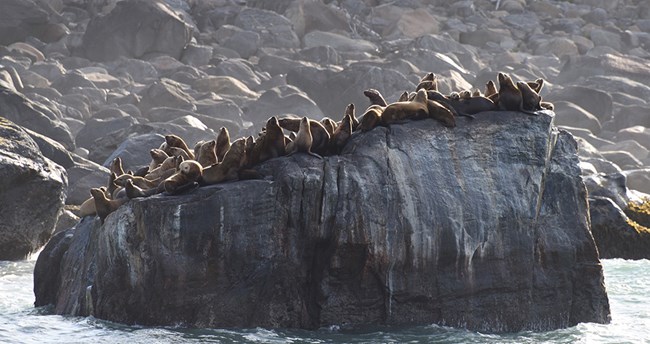
NPS/JAMIE WOMBLE
Population declines, reduced survival, and unusual mortality events occurred in marine mammals.
Across the Gulf of Alaska, population declines, reduced survival, and unusual mortality events were also documented for whales and pinnipeds. For example, higher whale mortality was reflected in Glacier Bay National Park’s long-term monitoring of humpback whales; their abundance declined by 56% between 2013 and 2018, followed by increases in 2019-2020 (Gabriele et al. 2022). Calf survival dropped by a factor of ten (from 39% down to 3%) during and after the heatwave. Females also began having calves much less frequently. For a five-year period during and after the PMH (2015-2019) there was about one calf born for every 25 adult females (0.041 calves per adult female) in contrast to one calf per three females (0.27 calves per adult female) prior to the PMH.
Long-term monitoring of pinniped populations in Glacier Bay documented declines in Steller sea lions at the primary haul-out site at South Marble Island (Whitlock et al. 2020). The number of harbor seals also declined in Johns Hopkins Inlet, a tidewater glacier fjord in Glacier Bay (Womble et al. 2020), but seal abundance in fjords is also influenced by the availability of ice habitat and changing tidewater glaciers (Womble et al. 2021). Studies across the broader region of the Gulf of Alaska also demonstrated declines in abundance, changes in diet, and reduced survival of Steller sea lions following the marine heatwave (Sweeney et al. 2022, Hastings et al. 2023, Maniscalco et al. 2023).
Unusual mortality events (UMEs) are defined as a significant die-off of any marine mammal population as described under the Marine Mammal Protection Act. Hundreds to thousands of sea lion pups died in 2015 off the California coast and fur seals died in large numbers and experienced reproductive failures in the North Pacific. During the 2015 Large Whale UME in the Gulf of Alaska, a record number of humpback and fin whales were found dead in 2015-2016 along the Alaska and British Columbia coasts (Gabriele et al. 2022). The timing and location of these events coincided with warm-water conditions and forage depletion, further demonstrating the far-reaching impacts of the marine heatwave through all levels of the food chain.
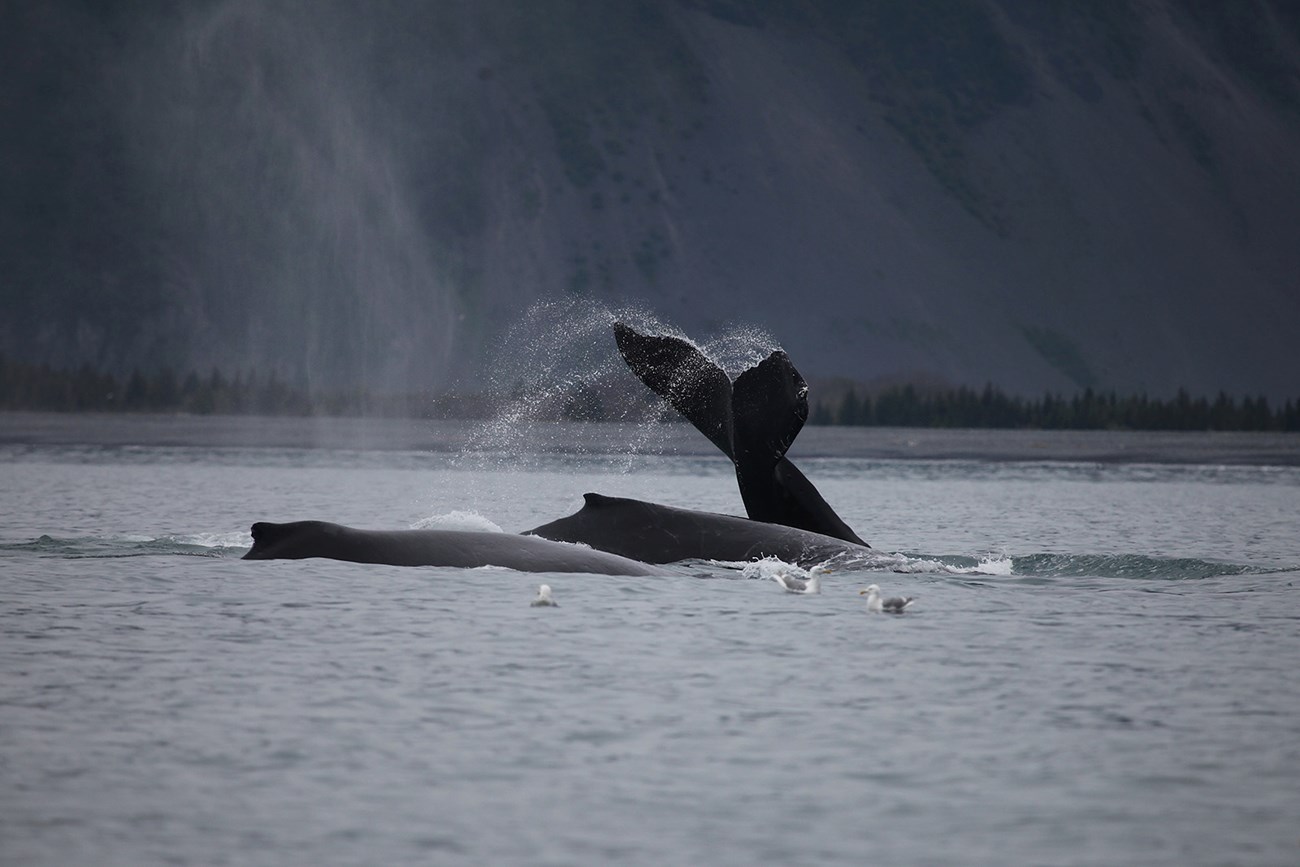
NPS PHOTO TAKEN UNDER AUTHORIZATION OF NATIONAL MARINE FISHERIES SERVICE SCIENTIFIC RESEARCH PERMIT #21059
Nearshore Food Web Responses Were Mixed
The nearshore is defined as the relatively shallow waters that run along the coastlines and, in the North Pacific, is constrained by light-level penetration. Hence, the food web is primarily driven by kelps and seagrasses with higher trophic-level consumer species that include invertebrates (for example, clams and mussels), nearshore sea birds, and sea otters. Primary consumers (macroinvertebrates) like mussels, clams, and sea urchins act as conduits of energy from the primary producers to the higher trophic-level consumers. Sea stars and sea otters, both keystone predators in the nearshore, shape the ecosystem in which they reside through their foraging behaviors by consuming a large variety of macroinvertebrates. Black oystercatchers, a charismatic shorebird that feeds on mussels and other intertidal macroinvertebrates, resides in the nearshore and nests in the intertidal (Dean et al. 2014).
The physical environment of the nearshore is also important. The intertidal zone (the area exposed to air at low tide and covered by water at high tide), within the nearshore ecosystem, experiences a wide range of extreme physical conditions due to the daily rising and falling tides. Low tides expose organisms to air, which could mean desiccation or freezing depending on the local conditions. While submerged during low tides, changes in water chemistry, such as decreased salinity or rising water temperatures, may stress organisms as well. Space is also a limiting factor in the intertidal, so large-scale disturbances that open up space generally result in competition between species for which ones can settle first. The species that reside in the nearshore are well adapted to these extremes.
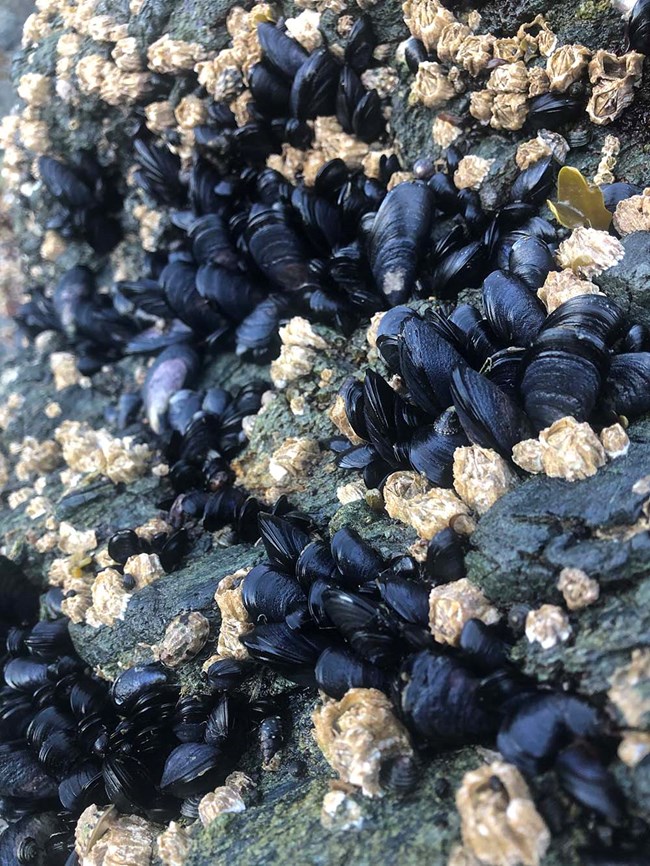
Diverse rocky intertidal communities became more similar across the Gulf of Alaska.
Responses to the marine heatwave at rocky intertidal sites indicated major changes in community structure. Sites monitored from Prince William Sound west to Kenai Fjords National Park, Kachemak Bay, and Katmai National Park and Preserve have historically been very different from each other, with measurable differences between species composition and abundance across sites, likely driven by the local-scale conditions of each site. However, these sites became more similar with similar species composition and abundance than in years prior to the heatwave. This homogenization manifested itself as a shift from communities dominated by macroalgae (kelps and seaweeds) to communities dominated by mussels and barnacles, essentially indicating a decline in the primary producers at the base of the nearshore food web concurrent with the PMH. The decline in macroalgae also likely created open space for mussels and barnacles to settle (Weitzman et al. 2021).
Sea stars declined, but mussels increased.
Sea stars, a top-level predator and keystone species in the intertidal, suffered significant population declines likely due to increased prevalence of sea star wasting (SSW; Konar et al. 2019), a phenomenon thought to be exacerbated by warm water temperature anomalies (Eisenlord et al. 2016). Due to the decline in macroalgae (described above), the increase in available space and decrease in predation pressure from the decline in sea stars likely allowed mussel populations to flourish (Traiger et al. 2022). In general, we also found that other benthic invertebrate prey species remained relatively stable (Robinson et al. in press).
A variable upper trophic-level response to changing prey availability.
Even with a general increase in mussel abundance, sea otter populations did not seem to change with the increased availability of mussels, a common prey item. However, preliminary analysis of diet and foraging effort indicates that there was a shift in the sea otter diet to one consisting of a higher proportion of mussels (Traiger et al. 2022). Another mussel-eating predator in the nearshore, the black oystercatcher, had a different response than sea otters. The abundance of black oystercatchers increased sharply in the eastern and western Gulf of Alaska concurrent with an increase in mussels, however, there was no observable response in the productivity of black oystercatchers (Suryan et al. 2021). Nearshore marine birds that rely on benthic prey (mussels, clams, and other intertidal invertebrates) also did not show a strong response to the PMH. This is in contrast to the forage-fish reliant seabirds such as the common murre, which collapsed during the PMH.
The stark differences in prey availability and predator response between the pelagic and nearshore ecosystems during the PMH indicates that a large-scale perturbation, such as the PMH, may impact distinct trophic pathways in the marine system differently (Robinson et al. in press).
Impacts from the Heatwave are Persistent Across Marine Ecosystems
Many biological shifts attributed to the heatwave, from changes to offshore primary production to shifts in nearshore rocky intertidal communities, persisted for at least five years after the onset of the Pacific Marine Heatwave. In many cases, the perturbation was strong enough to override typical local-scale drivers of the system (Suryan et al. 2021). Marine heatwaves are expected to become more common and widespread because of climate change. Our studies offer insight into the varying extent of responses to this broad-scale perturbation.
The studies highlighted here are the result of coupling long-term monitoring data with focused research studies to assess changes over time in marine ecosystems in and adjacent to national parks in Alaska. In the absence of long-term monitoring data, documenting responses to such a large-scale perturbation as the Pacific Marine Heatwave would not be possible. Our oceans are experiencing myriad changes, both direct and indirect, and subsequent consequences from climate change such as (but not limited to): ocean acidification, deoxygenation, changes in stratification, and decreased productivity. Documenting how species respond to these changes will allow us to anticipate potential outcomes of future perturbations and monitor how and when recovery may occur.
References
Arimitsu, M. L., J. F. Piatt, S. Hatch, R. M. Suryan, S. Batten, M. A. Bishop, R. W. Campbell, H. Coletti, D. Cushing, K. Gorman, R. R. Hopcroft, K. J. Kuletz, C. Marsteller, C. McKinstry, D. McGowan, J. Moran, S. Pegau, A. Schaefer, S. Schoen, J. Straley, and V. R. von Biela. 2021.
Heatwave-induced synchrony within forage fish portfolio disrupts energy flow to top pelagic predators. Global Change Biology 27(9): 1859-1878.
Bond, N. A., M. F. Cronin, H. Freeland, and N. Mantua. 2015.
Causes and impacts of the 2014 warm anomaly in the NE Pacific. Geophysical Research Letters 42(9): 3414–3420.
Dean, T. A., J. L. Bodkin, and H. A. Coletti. 2014.
Protocol Narrative for Nearshore Marine Ecosystem Monitoring in the Gulf of Alaska: Version 1.1. Natural Resource Report NPS/SWAN/NRR - 2014/756. Fort Collins, Colorado.
Eisenlord, M. E., M. L. Groner, R. M. Yoshioka, J. Elliott, J. Maynard, S. Fradkin, M. Turner, K. Pyne, N. Rivlin, R. van Hooidonk, and C. D. Harvell. 2016.
Ochre star mortality during the 2014 wasting disease epizootic: Role of population size structure and temperature. Philosophical Transactions of the Royal Society B: Biological Sciences 371(1689): 20150212.
Frölicher, T. L., E. M. Fischer, and N. Gruber. 2018.
Marine heatwaves under global warming. Nature 560: 360-364.
Hastings, K. K., T. S. Gelatt, J. M. Maniscalco, L. A. Jemison, R. Towell, G. W. Pendleton, and D. S. Johnson. 2023.
Reduced survival of Steller sea lions in the Gulf of Alaska following marine heatwave. Frontiers in Marine Science 10: 1127013.
Gabriele, C. M., C. L. Amundson, J. L. Neilson, J. M. Straley, C. S. Baker, and S. L. Danielson. 2022.
Sharp decline in humpback whale (Megaptera novaeangliae) survival and reproductive success in southeastern Alaska during and after the 2014–2016 Northeast Pacific marine heatwave. Mamm Biol 102: 1113-1131.
Gruber, N., P. W. Boyd, T. L. Frölicher, and M. Vogt. 2021.
Biogeochemical extremes and compound events in the ocean. Nature 600: 395-407.
Konar, B., T. J. Mitchell, K. Iken, H. Coletti, T. Dean, D. Esler, M. Lindeberg, B. Pister, and B. Weitzman. 2019.
Wasting disease and static environmental variables drive sea star assemblages in the northern Gulf of Alaska. Journal of Experimental Marine Biology and Ecology 520: 151209.
Maniscalco, J. M. 2023.
Changes in the overwintering diet of Steller sea lions (Eumetopias jubatus) in relation to the 2014–2016 northeast Pacific marine heatwave. Global Ecology and Conservation 43: e02427.
Piatt, J. F., J. K. Parrish, H. M. Renner, S. K. Schoen, T. T. Jones, M. L. Arimitsu, K. J. Kuletz, B. Bodenstein, M. Garcia-Reyes, R. S. Duerr, R. M. Corcoran, R. S. A. Kaler, G. J. McChesney, R. T. Golightly, H. A. Coletti, R. M. Suryan, H. K. Burgess, J. Lindsey, K. Lindquist, P. M. Warzybok, J. Jahncke, J. Roletto, and W. J. Sydeman. 2020.
Extreme mortality and reproductive failure of common murres resulting from the northeast Pacific marine heatwave of 2014-2016. PLOS ONE 15(1): e0226087.
Sweeney, K. L., B. Birkemeier, K. Luxa, and T. Gelatt. 2022.
Results of Steller Sea Lion Surveys in Alaska, June-July 2021. Memorandum to The Record. Results of Steller Sea Lion Surveys in Alaska, June-July 2021 (noaa.gov)
Robinson, B., H. A. Coletti, B. Ballachey, J. L. Bodkin, K. Kloecker, S. B. Traiger and D. Esler. In press.
Lack of strong responses to the Pacific marine heatwave by benthivorous marine birds indicates importance of trophic drivers. Marine Ecology Progress Series.
Suryan, R. M., M. L. Arimitsu, H. A. Coletti, R. R. Hopcroft, M. R. Lindeberg, S. J. Barbeaux, S. D. Batten, W. J. Burt, M. A. Bishop, J. L. Bodkin, R. Brenner, R. W. Campbell, D. A. Cushing, S. L. Danielson, M. W. Dorn, B. Drummond, D. Esler, T. Gelatt, D. H. Hanselman, S. H. Hatch, S. Haught, K. Holderied, K. Iken, D. B. Irons, A. B. Kettle, D. G. Kimmel, B. Konar, K. J. Kuletz, B. J. Laurel, J. M. Maniscalco, C. Matkin, C. A. E. McKinstry, D. H. Monson, J. R. Moran, D. Olsen, W. A. Palsson, W. S. Pegau, J. F. Piatt, L. A. Rogers, N. A. Rojek, A. Schaefer, I. B. Spies, J. M. Straley, S. L. Strom, K. L. Sweeney, M. Szymkowiak, B. P. Weitzman, E. M. Yasumiishi, and S. G. Zador. 2021.
Ecosystem response persists after a prolonged marine heatwave. Scientific Reports 11:6235.
Traiger, S. B., J. L. Bodkin, H. A. Coletti, B. Ballachey, T. Dean, D. Esler, K. Iken, B. Konar, M. R. Lindberg, D. Monson, B. Robinson, R. M. Suryan, and B. P. Weitzman. 2022.
Evidence of increased mussel abundance related to the Pacific marine heatwave and sea star wasting. Marine Ecology : e12715
Weitzman, B., B. Konar, K. Iken, H. Coletti, D. Monson, R. Suryan, T. Dean, D. Hondolero, and M. Lindeberg. 2021.
Changes in rocky intertidal community structure during a marine heatwave in the northern Gulf of Alaska. Frontiers in Marine Science 8: 1-18.
Whitlock, S. L., J. N. Womble, and J. T. Peterson. 2020.
Modelling pinniped abundance and distribution by combining counts at terrestrial sites and in-water sightings. Ecological Modelling 420: 108965.
Womble, J. N., J. M. Ver Hoef, S. M. Gende, and E. A. Mathews. 2020.
Calibrating and adjusting counts of harbor seals in a tidewater glacier fjord to estimate abundance and trends 1992 to 2017. Ecosphere 11(4): e03111.
Womble, J. N., P. J. Williams, R. W. McNabb, A. Prakash, R. Gens, B. S. Sedinger, and C. R. Acevedo. 2021.
Harbor seals as sentinels of ice dynamics in tidewater glacier fjords. Frontiers in Marine Science 8: e634541.
Last updated: November 9, 2023