Part of a series of articles titled Reckoning with a Warming Climate.
Article
Alaska’s Changing Vegetation Processes and Patterns: Plant Responses to Unprecedented Levels of Warming in the Far North
RESEARCH REVIEW
Carl Roland, Plant Ecologist, Denali National Park and Preserve and Central Alaska Network, National Park Service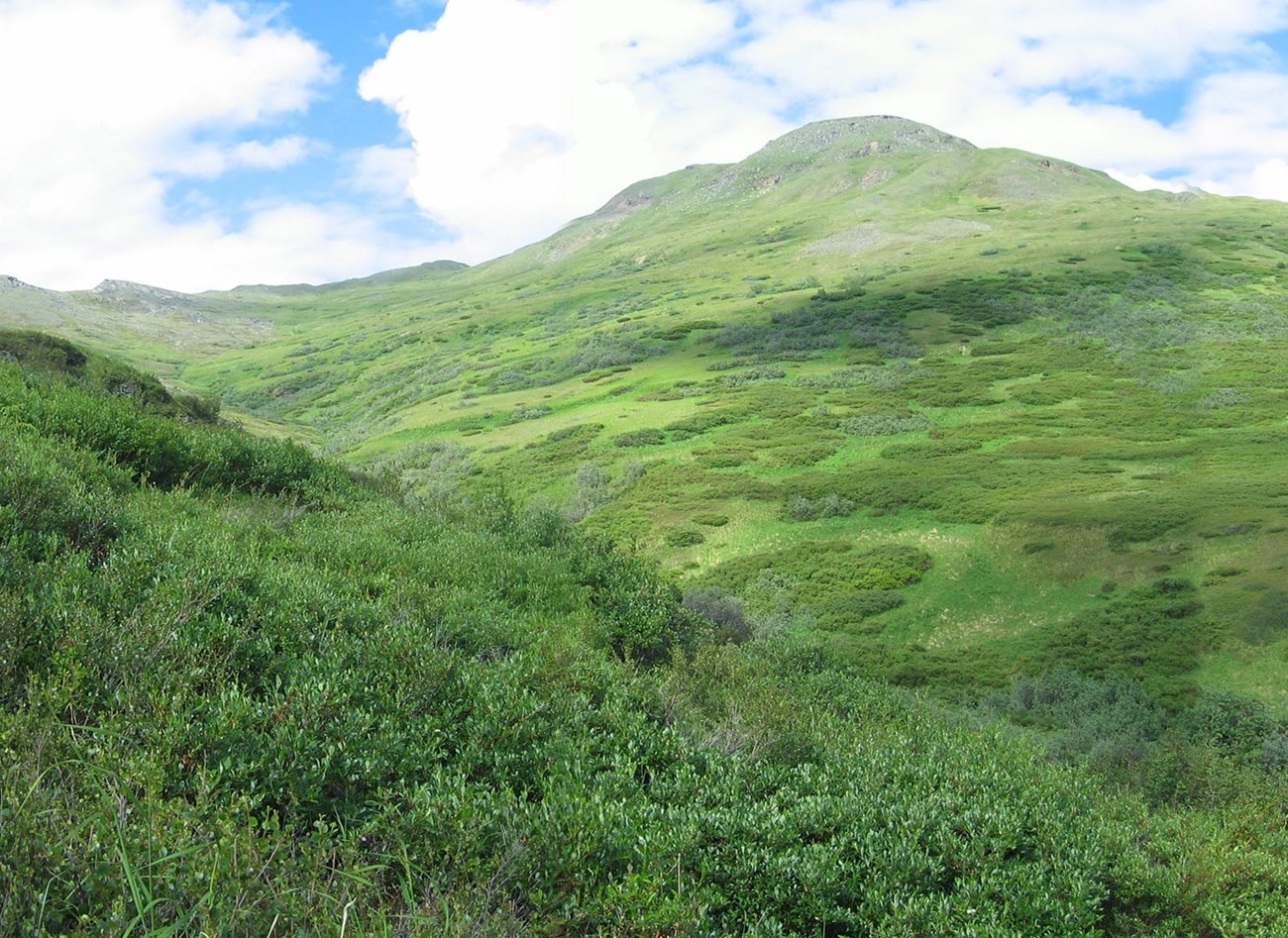
NPS/CARL ROLAND
In one sense, the vegetation of Alaska is in a constant state of flux, with the individual plants that make up familiar vegetation types that we see while traversing Alaska’s landscapes (e.g., forest, tundra, meadows) all busy with the processes of establishment, growth, reproduction, senescence, and decay, with each plant constantly responding to its environment during the growing season. In another sense, there is considerable stability in Alaska’s hardy vegetation. We can recognize clear, repeating patterns that reflect the varying conditions (including temperature, precipitation, solar energy, drainage, soil factors, and disturbance, among others) that influence the structure of plant communities discernible as we move through these northern landscapes.
For example, while traversing a river valley in Alaska, adjacent to each stream you will find open, gravelly soils deposited by flowing water and dotted with scattered colonizing plants including legumes. These open areas give way to a lining of willow thickets and then successively grade into stands of poplars, and then into spruce forest in places that have not been disturbed by flowing water in many decades. Similarly, as you ascend a mountainside from the forested lowlands, you soon emerge from under the forest canopy into shrubby treeline areas where shorter seasons, cold temperatures, and thin soils may inhibit the growth of trees thereby allowing more space and light so that smaller members of the plant kingdom can thrive there. Ultimately, if you climb high enough, you will traverse low tundra with its scattered cushion plants, mosses, lichens, and dwarfed plant species to reach the outer limits of plant growth in these cold, rocky high alpine areas. There are occasional splashes of green—the small alpine plants adapted to harsh conditions nestled among the slide rock and snow patches that dominate Alaska’s high mountain landscapes. These sorts of clear, reliable, and repeating structural patterns in the vegetation all reflect the strong control of plants by their environment in high-latitude areas such as Alaska.
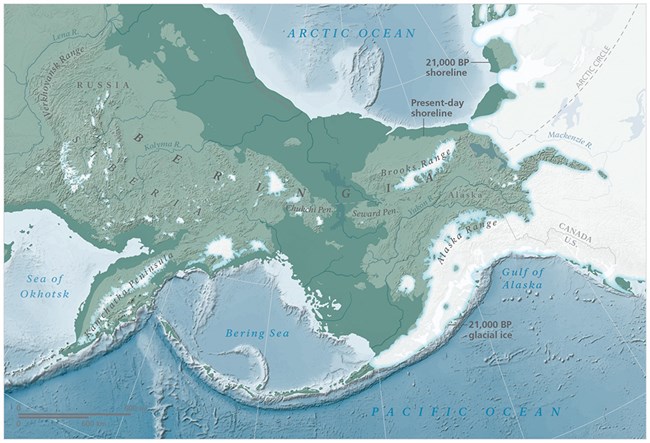
NPS MAP
A Brief Ecological History of Alaska
On a different timescale altogether, the science of paleoecology (which is concerned with the dynamics of ecological history going back thousands of years) gives us insights into another realm of stability and change—the massive ecological perturbations associated with the repeated epochs of advance and retreat of continental-scale sheets of ice hundreds of meters thick in Alaska: the ice ages! During the cold-phase periods of the Pleistocene Epoch, when Earth’s climate cooled, the accumulation of large snowpacks that failed to melt fully each summer steadily built gargantuan ice sheets that isolated Alaska from the rest of North America (what is now Canada was fully blanketed by glacial ice). At the same time, a good portion of Earth’s water was tied up in ice sheets in Earth’s high latitudes and mountains, which drew down sea levels, and consequently exposed many land bridges around the world. This is how the shallow continental shelf between Alaska and northeastern Asia (the Bering platform) was exposed and became the Bering Land Bridge creating Beringia—a large terrestrial ecosystem occupying areas formerly lying beneath the waves and adjacent areas of North America and Asia (Hopkins 1982).
During periodic glacial advances, forests and associated boreal woody vegetation types mostly vanished from Alaska’s landscapes (Matthews 1982, Edwards et al. 2000, Blinnikov et al. 2011), replaced by an open vegetation mosaic that included steppe-tundra, tundra, meadows, and shrublands in tune with the prevailing cooler, drier climatic conditions of the era. Ice-age eastern Beringia (what is now Alaska) was a refuge for biota surrounded by massive ice sheets to the south and east and the Bering Land Bridge to the west northward to the Arctic Ocean, which had receded toward the pole with the falling sea levels. The huge amounts of silt and other sediments ground up and transported by the icefields was another prominent feature of Alaska’s ice-age landscape—deep blankets of silt were deposited by running water and the fierce winds that blew across the open, treeless landscapes far from any ocean. Periodically, as the climate warmed again, the glaciers and ice fields once again shrank, revealing newly exposed and deeply gouged, barren landscapes as the copious meltwaters flowed down to the sea to refill the ocean basins and again flood the Bering platform and other land bridges as warmer and wetter inter-glacial conditions returned to the Far North.
In addition to shrinking the glaciers, conditions in these relatively warm and wet interglacial periods also prompted the expansion of trees and shrubs across the landscape of Alaska as boreal forest and other taller-statured woody vegetation types formerly confined south of the icesheets migrated in and displaced the relatively open and low plant communities characteristic of the ice age intervals in Beringia (Matthews 1982, Edwards et al. 2000, Blinnikov et al. 2011). Thus, the recognizable and widespread boreal forests that we now experience as Alaska’s vegetation are, in reality, relative newcomers that were mostly absent from these landscapes prior to the onset of our current interglacial period, the Holocene, starting about 12,000 years ago.
A brief consideration of the ecological history of what is now Alaska yields two clear conclusions. The first is that climate is an overarching and fundamental driver of the character, structure, and distribution of plant communities in the Far North. Secondly, periodic and massive change is deeply woven into the fabric of Alaskan ecosystems, which have been subject to repeated, dramatic shifts precipitated by disturbance and changing climatic conditions, among other drivers.
A Time of Unprecedented Change
The climate of Alaska is changing very rapidly. Startling levels of warming are being driven primarily by heat-trapping gases released by processes associated with industrialized human activities, which are predicted to intensify in coming decades (Box et al. 2019, Ballinger and Overland 2022). Based on what we know of Alaska’s ecosystems, it is likely that rapid warming and the associated changes in various atmospheric properties and processes (including humidity, precipitation, windiness, and other dynamics that directly affect biota) will have profound consequences for all life that resides here. Indeed, given the precipitous current rate of climate change, it seems increasingly likely that the transformations occurring in our lifetimes may render much of Alaska almost unrecognizable to us in what is, geologically speaking, the blink of an eye. Indeed, it seems likely that for many species of animals and plants, dependent solely on the legacy of their adaptations to heretofore ‘normal’ local conditions and relatively predictable (in both space and time) variation in the ecosystems of the Far North will face many arduous and difficult circumstances trying to cope with the unprecedented cascading changes that may be precipitated by rapid climate change. At the same time, some species (especially those with ranges extending to more southerly regions) may thrive in the new and unfamiliar warmed conditions spreading across the region.
There are a variety of ways to evaluate and categorize the types of changes that occur in ecosystems that help us to understand the biological significance and ramifications of such change. One useful way to do this is to distinguish process-related changes in an ecosystem (those that primarily occur in the moment in response to stimuli) from changes that are related to alterations in the structure or composition of the ecosystem (which usually integrate changes happening over longer intervals, such as a site changing from tundra to a forested condition). An easy way to think about this is that process-related changes are equivalent to individual plants and populations responding to weather events—for example, how warm or wet or sunny it is today or over this growing season. In contrast, structural changes in plant communities generally reflect modifications in response to climate—the prevailing norms such as long-term averages and extremes experienced at a site. These structural changes in ecosystems also most often reflect changes on the part of multiple interacting sets of organisms. In other words, one hot day or even a particularly warm summer will not convert tundra into forest, but a span of 50 summers with increasing warming combined with the availability of tree seeds, may do so.
While process-related and structural changes are closely inter-related (and not mutually exclusive) this framing does offer a useful template for us to think about how unprecedented warming is exerting various and transformative pressures on Alaska’s plant life. Some examples of important plant processes include phenology (the timing of biological events such as green-up in the spring), the rate and manner of uptake of water and nutrients, rates of vegetative growth, reproductive effort (the production of flowers and fruits, etc…), the effects of plant-eating insects on growth and development, and the germination and establishment of seedlings and related processes. These are processes that may be affected in a continuous way, as temperature, available moisture, or incident light changes through the course of a day or a growing season continuously affects the instantaneous rates of growth and respiration of plants, for example.
Structural or compositional changes in an ecosystem are transformations that involve changes in the species or growth forms of the organisms that make up a plant community. Such changes often result in very different spatial arrangements of the biomass in an ecosystem. For example, forested areas are structurally complex and have biomass arranged in multiple vertical layers (ground-level, sub-canopy, tree canopy), including high above the soil surface, whereas tundra areas are structurally simpler and all plant tissues occur quite close to the ground. For obvious reasons, then, structural changes usually require longer intervals of time to occur as (for example) one set of organisms replace another in response to altered conditions. Changes in plant process and function in the moment, if sufficiently pronounced and long term, will ultimately lead to changes in the structure and composition of plant communities. For example, if photosynthesis in a single spruce tree shuts down during a warm and dry afternoon, that is a transitory change that occurs in the moment and is physiological in nature. If this circumstance repeats itself over long periods of time and in many trees over large areas of the landscape, resulting in reduced wood production, then over time this will ultimately result in structural changes to the vegetation (reduced biomass of spruce). The distinction between the two types of change, then, is a matter of temporal and spatial scale of a change dynamic.
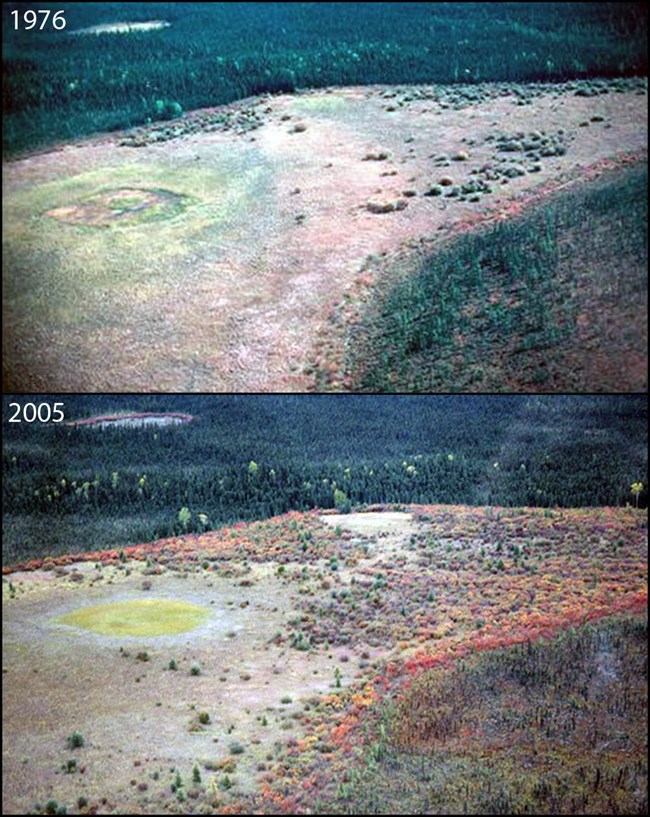
NPS/CARL ROLAND
An example of this dynamic that is directly relevant to Alaska is the process of wetland conversion. In this process, plants growing within a formerly submerged, but gradually drying wetland will suffer increasing drought stress and will thus grow and reproduce more slowly. Over time, seedlings of wetland plants will not be able to establish in periodically dry conditions, and gradually as members of these species perish due to altered conditions, they will be replaced by more drought-tolerant species that had previously been excluded from the site by their inability to establish or grow in saturated soils or standing water, thus eventually completing the transformation of the vegetation structure of the site over time. Ultimately, this process can inexorably lead to the transition of herbaceous sedge-dominated wetlands comprised of plants adapted to growing in saturated substrates into a shrub or tree-dominated woody terrestrial plant community with plants adapted to well-drained soils that periodically dry out. Such a transformation would represent an important structural change in the vegetation mosaic with many consequences for other members of the ecosystem, likely precipitating a transformation in the animal communities inhabiting the site. For example, insects, birds, and other animal species that nest among open wetlands or subsist upon herbaceous wetland plants for their diets likely do not also use shady, well-drained forested habitats or consume woody shrub plant tissues as a regular part of their diet.
While most process-related changes in plant function require sustained time periods to affect ecosystem structure and composition, major disturbances are an example of processes that frequently have immediate and important structural implications for vegetation patterns. For example, when a crown fire consumes a forested site and eliminates the vegetation, leaving behind an ashy plain, or when floodwaters erode away an intact stand of vegetation, replacing the plant community with newly deposited barren silt and gravel. Similarly, the catastrophic draining of a lake due to permafrost thaw, which is a phenomenon being observed repeatedly in Alaska of late (Swanson 2019) may result in a much faster conversion of lakeshore strand wetlands to upland vegetation than gradual drying related to increased evapotranspiration.
A survey of the recent ecological literature reveals that Alaska’s vegetation is already responding in myriad ways to our changing climate with profound consequences for resident biota. There is copious evidence for both process-related and structural changes being manifested with important and lasting implications for both humans and Alaska’s ecosystems. I discuss a selection of some of the most important changes that have been observed below, organized in terms of the two types of change—process-related or functional change, and change manifested by altered structure or composition of Alaskan plant communities.
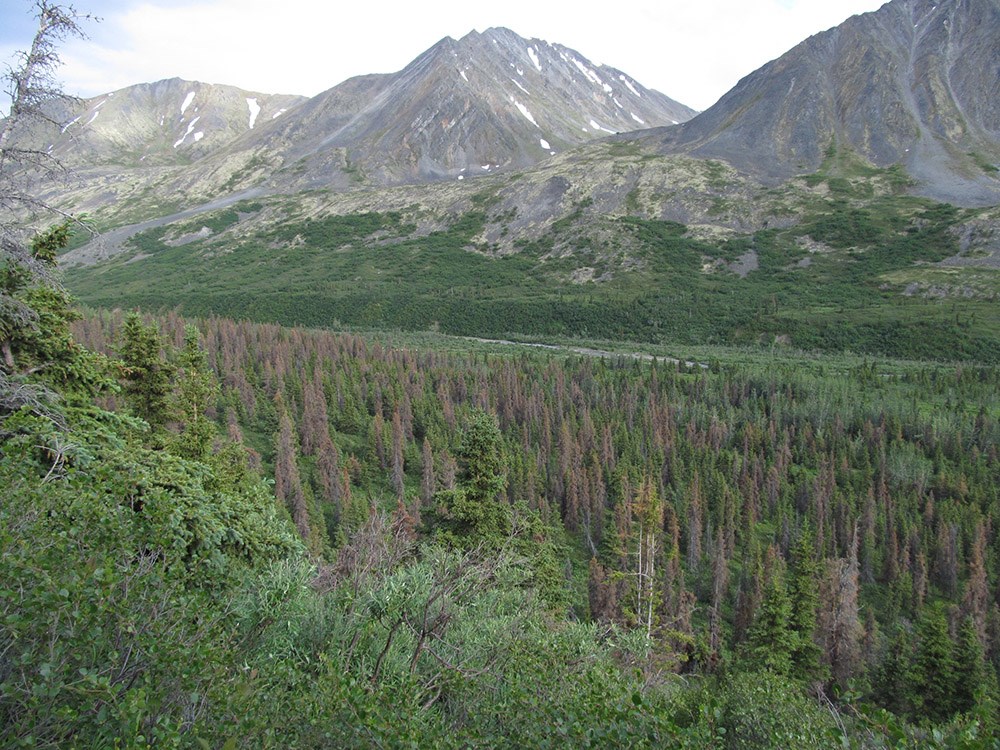
NPS/SARAH STEHN
Examples of process- and disturbance-related dynamics relating to climate change that have been observed and published for Alaska:
- Researchers have documented geo-graphically variable trends in Normalized Difference Vegetation Index (the relative greenness of the vegetation in summer associated with varying levels of annual productivity) across Alaska with some areas in colder and/or moister regions of the state showing trends of increasing summer greenness (reflecting greater productivity with warming) and some warmer, drier regions showing a “browning” trend over time related to heat and drought stressors on plant life (Jia et al. 2003, 2006, Verbyla 2008, Beck and Goetz 2011, Epstein et al. 2012, Pastick et al. 2018);
- Researchers have shown that changes in vegetation phenology have been observed at very large spatial scales, which include evidence of longer growing seasons that follow from earlier snowmelt and warmer early season temperatures (Potter and Alexander 2020, Chen et al. 2022, Zheng 2022);
- Changes in reproductive effort and output of plants have been related to increasing productivity or changes in growing season conditions (for example, longer and warmer growing seasons (e.g., Roland et al. 2014, Barrett et al. 2015);
- Ecological studies including many areas of Alaska have shown plant responses to warming soils and thawing permafrost with examples that include localized landscape “wetting” from ice-wedge degradation (Jorgenson et al. 2001, 2018) and effects of soil active layer depth and air temperature on tree growth (Nicklen et al. 2016, 2019, 2021);
- In many areas of Alaska, there have been widespread or severe insect outbreaks that are likely enhanced by climate warming and have resulted in plant damage and mortality (see Berg et al. 2006, Verbyla 2008, Parent and Verbyla 2010, Wagner and Doak 2013, Cahoon et al. 2018);
- Researchers have documented changes in the frequency and severity of fire disturbance over time in Alaska (Kasischke et al. 2010) as well as the consequences of these changes in generating novel post-fire successional trajectories and changes in forests (Hu et al. 2015, Johnstone et al. 2006 a,b, Johnstone et al. 2010, Shenoy et al. 2011).
- Trends in annual tree growth in response to variations in temperature and pre-cipitation over long spans of time as encoded in tree rings (e.g., Walker and Johnstone 2014, Sullivan et al. 2017, Nicklen et al. 2021) resulting in changes in tree productivity across the Alaskan landscape.
- Changing seasonal dynamics relating to the timing and establishment of an insulating layer of snow in forested areas of Southeast Alaska due to changing winter precipitation regimes has left tree roots (such as those of yellow cedar) vulnerable to thaw-freeze events and in combination with multiple stressors relating to a changing climate, is causing substantial tree decline and mortality in the region (Hennon et al. 2012, Comeau and Daniels 2022).
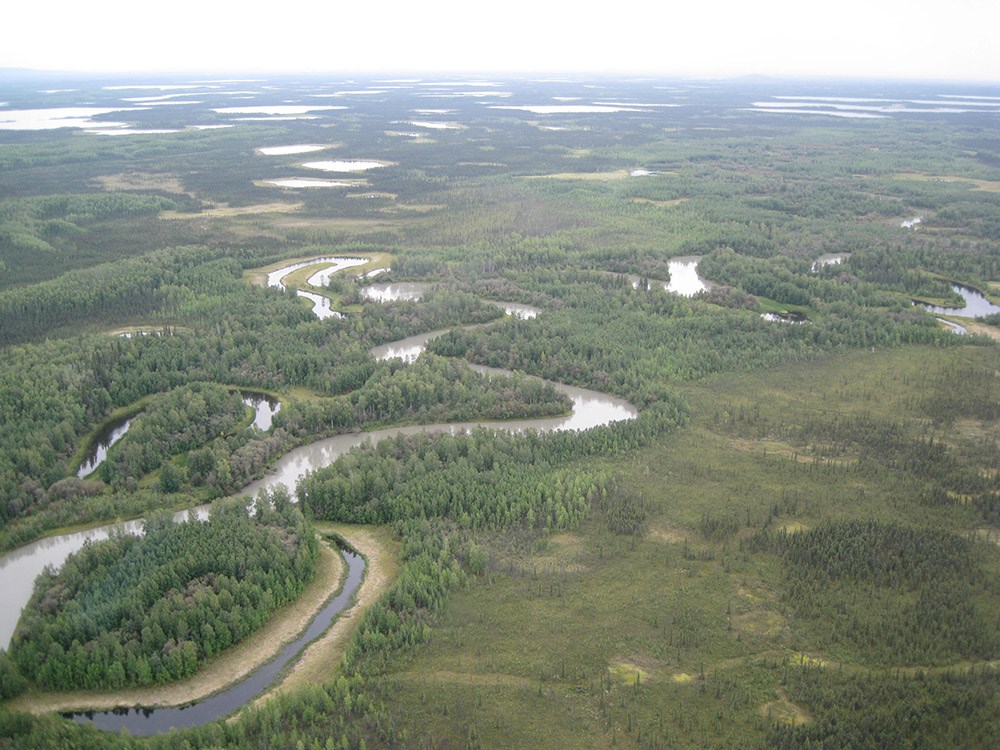
NPS/CARL ROLAND
Examples of warming-driven changes in vegetation structure and composition recorded in Alaska’s ecosystems:
- A group of Alaskan researchers recently published an extraordinary description of rapid northward expansion of white spruce trees into tundra in areas of northwestern Alaska in the journal Nature, which has major implications for the future (Dial et al. 2022). Far to the south, another group of Alaskan researchers showed that warming along the southern coastal region has driven a wave of spruce seedling establishment there as well (Miller et al. 2017);
- Diverse studies from widely scattered locations across Alaska have documented the expansion of woody and other vegetation on floodplains and similar early successional environments (including recently deglaciated sites) resulting in conspicuous increases in the stature and density of vegetation and reducing the extent of these heretofore open areas of the landscape over recent decades (Klaar et al. 2015, Roland et al. 2016, Brodie et al. 2019, Pastick et al. 2018, Frost et al. 2023, Tape et al. 2016, Fryday and Dillman 2022);
- Examinations of extensive-scale long-term patterns in the growth-form cover attributes of Alaska’s vegetation using remotely sensed imagery have revealed a variety of changes occurring over time, including net increases in deciduous shrubs, evergreen shrubs, broadleaf trees, and conifer trees with concomitant decreases in moss, lichen, and graminoid cover, among others (e.g., Cornliessen 2001, Macander et al. 2022, Pastick et al. 2018, Bao et al. 2022),
- Researchers predict that one important consequence of increases in the amount of forested area burned and shrinking time intervals between fires associated with a warming boreal zone is the possible widespread conversion of areas that have long been dominated by coniferous species to deciduous woodlands and forest dominated by birch, aspen, and balsam poplar. These broadleaved species produce huge, easily dispersed seed crops that thrive in recently burned terrain, where these rapidly growing species can thrive in the relatively nutrient rich, warm soils after high-severity fires (Johnstone et al. 2006 a, b, 2010).
- Another active area of research has focused on the spatial and temporal patterns of surface water fraction on the landscape of Alaska. This body of work has identified warming as one driver of the shrinking surface area occupied by lakes and ponds in recent in parts of Alaska (Yoshikawa and Hinzman 2003, Roach et al. 2013, Rupp and Larsen 2022). Associated with shrinking of surface waters, the (perhaps related) drying of wetland areas has catalyzed invasions of herbaceous wetland areas by shrubs in parts of Alaska (Klein et al. 2006, Berg et al. 2009).
- Researchers working in the Chugach Mountains and Kenai Mountains of Southcentral Alaska have found that tall shrubs are moving upslope into tundra at a faster rate than the treeline (Dial et al. 2015). Similar ecological dynamics of woody plants encroaching into tundra have been observed in other regions of Alaska as well, including the North Slope (Sturm et al. 2001) and areas of western Alaska (Terskaia et al. 2020) not to mention floodplains across large areas (Frost et al. 2023). Overall, Alaska has likely become a much woodier place over time as the climate has warmed, with many important consequences for the human cultures and animals that live here.
- There are other, perhaps less dramatic, but still important changes that botanists have forecasted given the various processes related to the development of a warmer, woodier, and more fire-prone Alaskan landscape (Roland and Schmidt 2015, Roland et al. 2017, Roland et al. 2019, Roland et al. 2021). For example, we can expect the gradual displacement of many of the endemic plant species and wildflowers that are unique to eastern Beringia and neighboring areas by more widespread, larger boreal plant species that will likely outcompete the endemic flora in a changed northern world. These endemic species have persisted over millennia in this region and reflect the long-term evolution of Alaska’s flora in relation to climate—they persist in areas with open vegetation, often very cold areas in high-elevation sites, refugees from the expanding woody vegetation types in the valleys below. While this change may not be as significant from an ecological point of view, in terms of changing bird and wildlife habitats or causing infrastructure issues for humans, it could represent another kind of loss—the further contracting of the superbly cold-adapted biota that has witnessed many changes over the millennia, including the comings and goings of vast icesheets across our northern homelands.
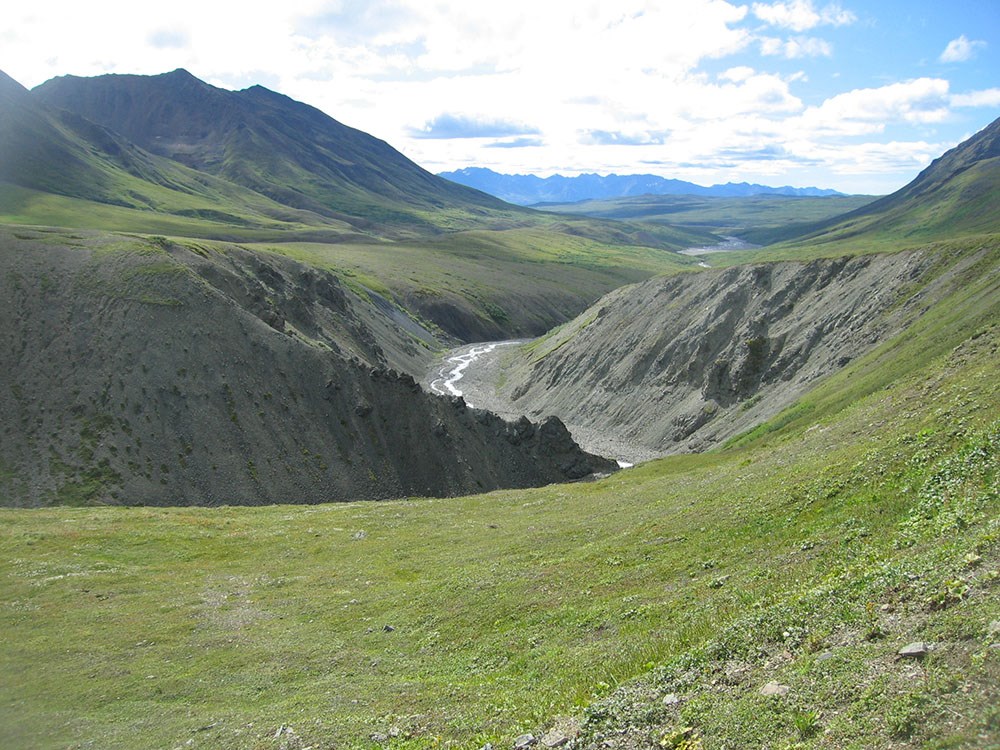
NPS/CARL ROLAND
As the selection of examples I have described above reveals, the cumulative scope and scale of the changes described in the scientific literature thus far are a harbinger of profound alteration of Alaska’s vegetation mosaic, especially given the strong likelihood that such changes will continue apace and even intensify in the future. However, the changes being observed are not evenly distributed across the landscape and certain areas, species, and plant communities are more or less sensitive to certain types of changes (e.g., Swanson 2015, Roland et al. 2016, Raiho et al. 2022). One source of resilience is that the dominant boreal forest species are, for the most part, quite widespread and occur over large gradients of climate and site conditions thus possessing relative wide ecological tolerances and plasticity to endure different sets of conditions. Additionally, site factors may also provide some resistance to rapid changes (Swanson 2015, Roland et al. 2016, Raiho et al. 2022), such as conditions associated with well-insulated and thus-far undisturbed permafrost being more resistant to observable structural vegetation change even over long spans of time. However, the redoubts where vegetation may be currently protected by the “inertia” afforded by substantial frozen ground and thus slower rates of change (Swanson 2015, Roland et al. 2016, Brodie et al. 2019) are not permanently protected given the current projections for disappearance of permafrost in large areas of Alaska in the coming decades (e.g., Panda 2014, Ballinger and Overland 2022).
One thing is certain, however, the vegetation changes instigated by a warming and changing climate will exert consequential and far-reaching influences on most of Alaska’s biota including invertebrates, birds, mammals, and humans, among others. This is because plants form the energetic foundations of terrestrial ecosystems (they are the primary base of all food webs) and define the primary structural elements of habitat for most animal species. For example, the insect and avifauna of tundra and other open habitats differs markedly from that of neighboring spruce forests and while there are many moose traversing the boreal forested lowlands of Alaska, it is much rarer to find caribou in these dense, taller-statured vegetation types in any numbers (and vice versa). Indeed, most animals, and particularly herbivores, are creatures of particular habitats that are primarily defined jointly by vegetation and associated physical factors of the environment. For this reason, we can expect what are referred to as “cascading” ecological effects following from vegetation change. That is, there are second-, third-, and fourth-order effects that may be set in motion, some of which will be difficult, if not impossible, to predict with confidence.
One example of this kind of complicated and multilayered ecological interaction was recently demonstrated by a group of Alaskan researchers working on beaver ponds in far Northwestern Alaska (Tape et al. 2022). Using aerial images, they found that (consistent with Indigenous knowledge and local observations) a conspicuous proliferation of beaver ponds has occurred in this huge area during the period 1949 to 2019. In fact, they estimated it’s likely that the construction of 10,000 beaver ponds in tundra-dominated areas has occurred in recent decades synchronously with the onset of considerable warming in the region, resulting in many and various downstream ecological consequences. This process may have a positive feedback element with warming as it is likely that the impounding of so much surface water by these ecosystem engineers is resulting in considerable thawing of permafrost as shallow surface waters absorb heat and transfer it to thawing submerged permafrost, thus releasing additional carbon into the atmosphere as peat and other organic sediments decompose as they thaw (Tape et al. 2018). While this study does not pinpoint the reasons for this apparent explosion in beaver distribution across the landscape, one likely contributing factor is the increasing availability of their preferred woody forage species, which are known to be expanding in response to rapid warming across these tundra regions. So, a warming world is prompting increased shrub growth, which, in turn, may be drawing in beavers whose engineering may be further exacerbating permafrost thaw and thus instigating further changes in a widening cascade of effects.
These ecological effects of humanity’s warming of the global climate represent some of the initial results of a huge experiment from which we are likely only starting to witness the outcomes. The ultimate complexity and reach are likely beyond our current comprehension. For example, the scope and scale of just the few examples of how the vegetation is changing in Alaska that I have described above would have likely been unfathomable even to very experienced and knowledgeable ecologists working in the state just 50 years ago. Indeed, these are unprecedented changes happening at a speed and scale with no analog in our collective history. For a thinking person, a consideration of the changing ecological dynamics occurring in the Far North inevitably begs the question: What further changes will befall Alaska in fifty years that we are currently unable to fathom, that will be wrought by this ongoing colossal and uncontrolled experiment?
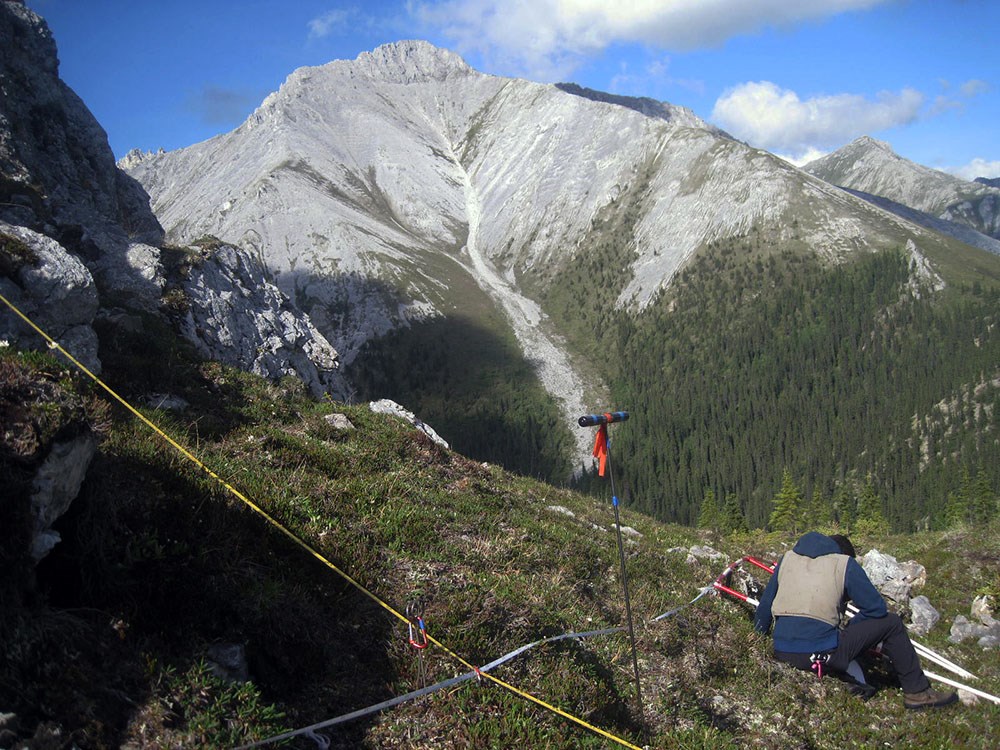
NPS/CARL ROLAND
References
Ballinger, T. K. and J. E. Overland. 2022.
The Alaskan Arctic regime shift since 2017: A harbinger of years to come? Polar Science 32: 100841.
Barrett, R. T., R. D. Hollister, S. F. Oberbauer, and C. E. Tweedie. 2015.
Arctic plant responses to changing abiotic factors in northern Alaska. American Journal of Botany 102(12): 2020-2031.
Bao, T., G. Jia, and X. Xu. 2022.
Warming enhances dominance of vascular plants over cryptogams across northern wetlands. Global Change Biology 28(13): 4097-4109.
Beck, P. S. A. and S. J. Goetz. 2011.
Satellite observations of high northern latitude vegetation productivity changes between 1982 and 2008: Ecological variability and regional differences. Environ. Res. Lett. 6: 045501.
Berg, E. E., J. D. Henry, C. L. Fustier, A. D. De Volder, and S. M. Matsuoka. 2006.
Spruce beetle outbreaks in spruce forests on the western Kenai Peninsula, Alaska, and Luanne National Park and Reserve, Yukon Territory: relationships with summer temperature and regional differences in disturbance regimes. For. Ecol. Manage. 227: 219-232.
Berg, E. E., K. McDonnell Hillman, R. Dial, and A. DeRuwe. 2009.
Recent woody invasion of wetlands on the Kenai Peninsula Lowlands, south-central Alaska: A major regime shift after 18,000 years of wet Sphagnum–sedge peat recruitment. Can. J. For. Res. 39: 2033-2046.
Blinnikov, M. S., B. V. Gaglioti, D. A. Walker, M. J. Wooller, and G. D. Zazula. 2011.
Pleistocene graminoid-dominated ecosystems in the Arctic. Quaternary Science Reviews 30: 2906-2929.
Box, J., W. T. Colgan, T. R. Christensen, N. M. Schmidt, M. Lund, F. W. Parmentier, R. Brown, U. S. Bhatt, E. S. Euskirchen, V. E. Romanovsky, J. E. Walsh, J. E. Overland, M. Wang, R. W. Corell, W. N. Meier, B. Wouters, S. Mernild, J. Mard, J. Pawlak, and M. S. Olsen. 2019.
Key indicators of Arctic climate change: 1971–2017. Environ. Res. Lett. 14: 045010.
Brodie, J. F., C. A. Roland, S. E. Stehn, and E. Smirnova. 2019.
Variability in the expansion of trees and shrubs in boreal Alaska. Ecology 100: e02660.
Cahoon, S. M. P., P. F. Sullivan, A. H. Brownlee, R. R. Pattison, H. E. Andersen, K. Legner, and T. N. Hollingsworth. 2018.
Contrasting drivers and trends of coniferous and deciduous tree growth in interior Alaska. Ecology 99: 1284-1295.
Chen, X., Y. Yang, and J. Du. 2022.
Distribution and attribution of earlier start of the growing season over the Northern Hemisphere from 2001-2018. Remote Sens. 14: 2964.
Comeau, V. M. and L. D. Daniels. 2022.
Multiple divergent patterns in yellow-cedar growth driven by anthropogenic climate change. Climatic Change 170: 22.
Cornelissen, J. H. C., T. V. Callaghan, J. M. Alatalo, A. Michelsen, E. Graglia, A. E. Hartly, D. S. Hik, S. E. Hobbie, M. C. Press, C. H. Robinson, G. H. R. Henry, G. R. Shaver, G. K. Phoenix, D. Gwynn Jones, S. Jonasson, F. S. Chapin III, U. Molau, C. Neill, J. A. Lee, J. M. Melillo, B. Sveinbornsson, and R. Aerts. 2001.
Global change and arctic ecosystems: Is lichen decline a function of increases in vascular plant biomass? Journal of Ecology 89(6): 984-994.
Dial, R. J., T. S. Smeltz, P. F. Sullivan, C. L. Rinas, K. Timm, J. E. Geck, S. C. Tobin, T. S. Golden, and E. C. Berg. 2015.
Shrub-line but not treeline advance matches climate velocity in montane ecosystems of south-central Alaska. Global Change Biology 22(5): 1841-1856.
Dial, R. J., C. T. Maher, R. E. Hewitt, and P. F. Sullivan. 2022.
Sufficient conditions for rapid range expansion of a boreal conifer. Nature 608: 546-551.
Epstein, H. E., M. K. Raynolds, D. A. Walker, U. S. Bhatt, C. J. Tucker, and J. E. Pinzon. 2012.
Dynamics of aboveground phytomass of the circumpolar arctic tundra over the past three decades. Environ. Res. Lett. 7: 015506.
Edwards, M. E., P. M. Anderson, L. B. Brubaker, T. A. Ager, A. A. Andreev, N. H. Bigelow, L. C. Cwynar, W. R. Eisner, S. P. Harrison, F. S. Hu, D. Jolly, A. V. Lozhkin, G. M. MacDonald, C. J. Mock, J. C. Ritchie, A. V. Sher, R. W. Spear, J. W. Williams, and G. Yu. 2000.
Pollen-based biomes for Beringia 18,000, 6000 and 0 14C yr bp. Journal of Biogeography 27: 521-554.
Frost, G. F., C. A. Roland, and J. H. Schmidt. 2023.
Dynamic disequilibrium: Recent widespread increases in vegetation cover on subarctic floodplains of Interior Alaska. Ecosphere 14(1): e4344.
Fryday, A. M. and K. L. Dillman. 2022.
A preliminary investigation of the lichen biota associated with recently deglaciated terrain in southeastern Alaska. Botany 100: 313-328.
Hennon, P. E., D. V. D’Amore, P. G. Schaberg, D. T. Wittwer, and C. S. Shanley. 2012.
Shifting climate, altered niche, and a dynamic conservation strategy for yellow-cedar in the North Pacific Coastal Rainforest. Bioscience 62: 147-158.
Hopkins, D. M. 1982.
Aspects of the paleogeography of the Bering Land Bridge during the late Pleistocene. pp. 3-28 In D. M. N. Hopkins, et al. (eds) Paleoecology of Beringia. Academic Press, New York, NY.
Hu, F. S., P. E. Higuera, P. Duffy, M. L. Chipman, A. V. Rocha, A. M. Young, R. Kelly, and M. C. Dietze. 2015.
Arctic tundra fires: Natural variability and responses to climate change Front. Ecol. Environ. 13: 369-377.
Jia, G. J., H. E. Epstein, and D. A. Walker. 2003.
Greening of Arctic Alaska, 1981-2001. Geophysical Research Letters 30: 2067.
Jia, G. J., H. E. Epstein, and D. A. Walker. 2006.
Spatial heterogeneity of tundra vegetation response to recent temperature changes. Global Change Biology 12: 42-55.
Johnstone, J. F. and F. S. Chapin III. 2006a.
Effects of soil burn severity on post-fire tree recruitment in boreal forest. Ecosystems 9: 14-31.
Johnstone, J. F. and F. S. Chapin III. 2006b.
Fire interval effects on successional trajectory in boreal forests of Northwest Canada. Ecosystems 9: 268-277.
Johnstone, J. F., F. S. Chapin III, T. N. Hollingsworth, M. C. Mack, V. Romanovsky, and M. Turetsky. 2010.
Fire, climate change, and forest resilience in interior Alaska. Canadian Journal of Forest Research 40: 1302-1312.
Jorgenson, M.T., C. H. Racine, J. C. Walters, and T. E. Osterkamp. 2001.
Permafrost degradation and ecological changes associated with a warming climate in central Alaska. Clim. Chang. 48: 551-579.
Jorgenson, J. C., M. T. Jorgenson, M. L. Boldenow, and K. M. Orndahl. 2018.
Landscape change detected over a half century in the Arctic National Wildlife Refuge using high-resolution aerial imagery. Remote Sens. 10(8): 1305.
Kasischke, E. S., D. L. Verbyla, T. S. Rupp, A. D. McGuire, K. A. Murphy, R. Jandt, J. L. Barnes, E. E. Hoy, P. A. Duffy, M. Calef, and M. R. Turetsky. 2010.
Alaska’s changing fire regime—implications for the vulnerability of its Boreal forests. Canadian Journal of Forest Research 40: 1313-1324.
Klaar, M. J., C. Kidd, E. Malone, R. Bartlett, G. Pinay, F. S. Chapin, and A. Milner. 2015.
Vegetation succession in deglaciated landscapes: Implications for sediment and landscape stability. Earth Surface Processes and Landforms 40(8): 1088-1100.
Klein, E., E. E. Berg, and R. Dial. 2005.
Wetland drying and succession across the Kenai Peninsula Lowlands, south-central Alaska. Can. J. For. Res. 35: 1931.1941.
Macander, M. J., P. R. Nelson, T. W. Nawrocki, G. V. Frost, K. M. Orndahl, E. .C Palm, A. F. Well, and S. J. Goetz. 2022.
Time-series maps reveal widespread change in plant functional type cover across Arctic and boreal Alaska and Yukon. Environmental Research Letters 17: 054042.
Matthews, J. J. V. 1982.
East Beringia during late Wisconsin time: A review of the biotic evidence. pp. 307-326 In D. M. N. Hopkins, et al. (eds) Paleoecology of Beringia. Academic Press, New York, NY.
Miller, A., T. Wilson, R. Sherriff, and J. Walton, J. 2017.
Warming drives a front of white spruce establishment near western treeline, Alaska. Global Change Biology 23: 5509-5522.
Nicklen, E. F., C. A. Roland, R. A. Ruess, J. H. Schmidt, and A. H. Lloyd. 2016.
Local site conditions drive climate-growth responses of Picea mariana and Picea glauca in interior Alaska. Ecosphere 7: 1-17.
Nicklen, E. F., C. A. Roland, A. Z. Csank, M. Wilmking, R. W. Ruess, and L. A. Muldoon. 2019.
Stand basal area and solar radiation amplify white spruce climate sensitivity in interior Alaska: Evidence from carbon isotopes and tree rings. Global Change Biology 25(3): 911-926.
Nicklen, E. F., C. A. Roland, R. W. Ruess, T. Scharnweber, and M. Wilmking. 2021.
Divergent responses to permafrost and precipitation reveal mechanisms for the spatial variation of two sympatric spruce. Ecosphere 12(7): e03622.
Panda, S., S. Marchenko, and V. Romanovsky. 2014.
High-resolution permafrost modeling in Denali National Park and Preserve. Natural Resource Technical Report NPS/CAKN/NRTR–2014/858. National Park Service, Fort Collins, Colorado, USA.
Parent, M. B. and D. Verbyla. 2010.
The Browning of Alaska’s Boreal Forest. Remote Sensing, 2 (12): 2729-2747.
Pastick, N. J., M. T. Jorgenson, S. J. Goetz, B. M. Jones, B. K. Wylie, B. J. Minsley, H. Genet, J. F. Knight, D. K. Swanson, and J. C. Jorgenson. 2018.
Spatiotemporal remote sensing of ecosystem change and causation across Alaska. Global Change Biology 25(3): 1171-1189.
Potter, C. and O. Alexander. 2020.
Changes in vegetation phenology and productivity in Alaska over the past two decades. Remote Sens.12 (10): 1546.
Raiho, A. M., H. R. Scharf, C. A. Roland, D. K. Swanson, S. E. Stehn, and M. B. Hooten. 2022.
Searching for refuge: A framework for identifying site factors conferring resistance to climate-driven vegetation change. Diversity and Distributions 28: 1-17.
Roach, J. K., B. Griffith, and D. Verbyla. 2013.
Landscape influences on climate-related lake shrinkage at high latitudes. Global Change Biology 19(7): 2276-84.
Roland, C. A., J. H. Schmidt, and J. F. Johnstone. 2014.
Climate sensitivity of reproduction in a mast-seeding boreal conifer across its distributional range from lowland to treeline forests. Oecologia 174: 665-677.
Roland, C. A. and J. H. Schmidt. 2015.
A diverse alpine species pool drives a “reversed” plant species richness-elevation relationship in interior Alaska. Journal of Biogeography 42: 738-750.
Roland, C. A., S. E. Stehn, J. H. Schmidt, and B. Houseman. 2016.
Proliferating poplars: the leading edge of landscape change in an Alaskan subalpine chronosequence. Ecosphere 7(7): e01398.
Roland, C. A., S. E. Stehn, and J. H. Schmidt. 2017.
Species richness of multiple functional groups peaks in alpine tundra in subarctic Alaska. Ecosphere 8: e01848.
Roland, C. A., G. Sadoti, E. F. Nicklen, S. A. McAfee, and S. E. Stehn. 2019.
A structural equation model linking past and present plant diversity in Alaska: a framework for evaluating future change. Ecosphere 10: e02832.
Roland, C. A., J. H. Schmidt, S. E. Stehn, C. Hampton-Miller, and E. F. Nicklen. 2021.
Borealization and its discontents: Drivers of regional variation in plant diversity across scales in interior Alaska. Ecosphere 12(5): e03485.
Rupp, D. L. and A. S. Larsen. 2022.
Surface water area in a changing climate: Differential responses of Alaska’s subarctic lakes. PLOS Clim 1(6): e0000036.
Shenoy, A., J. F. Johnstone, E. S. Kasischke, and K. Kielland. 2011.
Persistent effects of fire severity on early successional forests in interior Alaska. Forest Ecology and Management 261: 381-390.
Sturm, M., C. Racine, and K. Tape. 2001.
Increasing shrub abundance in the Arctic. Nature 411: 546-547.
Sullivan, P. F., R. R. Pattison, A. H. Brownlee, S. M. P. Cahoon, and T. N. Hollingsworth. 2017.
Limited evidence of declining growth among moisture-limited black and white spruce in interior Alaska. Scientific Reports 7: 15344.
Swanson, D. K. 2015.
Environmental limits of tall shrubs in Alaska’s Arctic national parks. PLoS One 10: e0138387.
Swanson, D. K. 2019.
Thermokarst and precipitation drive changes in the area of lakes and ponds in the National Parks of northwestern Alaska, 1984-2018. Arctic, Antarctic, and Alpine Research 51(1): 265-279.
Tape, K. D., D. D. Gustine, R. W. Ruess, L. G. Adams, and J. A. Clark. 2016.
Range expansion of moose in Arctic Alaska linked to warming and increased shrub habitat. PLoS One 11: e0152636.
Tape, K. D., B. M. Jones, C. D. Arp, I. Nitze, and G. Grosse. 2018.
Tundra be dammed: Beaver colonization of the Arctic. Glob. Change Biol. 24: 4478-88.
Tape, K. D., J. A. Clark, B. M. Jones, S. Kantner, B. V. Gaglioti, G. Grosse, and I. Nitze. 2022.
Expanding beaver pond distribution in Arctic Alaska, 1949 to 2019. Sci Rep 12(1): 7123.
Terskaia, A., R. J. Dial, and P. F. Sullivan. 2020.
Pathways of tundra encroachment by trees and tall shrubs in the western Brooks Range of Alaska. Ecography 43: 769-78.
Verbyla, D. 2008.
The greening and browning of Alaska based on 1982-2003 satellite data. Global Ecology and Biogeography 17: 547-555.
Wagner, D. and P. Doak. 2013.
Long-term impact of a leaf miner outbreak on the performance of quaking aspen. Canadian Journal of Forest Research 43(6): 563-569.
Walker, X. and J. F. Johnstone. 2014.
Widespread negative correlations between black spruce growth and temperature across topographic moisture gradients in the boreal forest. Environmental Research Letters 9: 064016.
Yoshikawa, K. and L. D. Hinzman. 2003.
Shrinking thermokarst ponds and groundwater dynamics in discontinuous permafrost near Council, Alaska. Permafrost and Periglacial Processes 14(2): 151-60.
Zheng, J., G. Jia and X. Xu. 2022.
Earlier snowmelt predominates advanced spring vegetation green-up in Alaska. Agricultural and Forest Meteorology 315: 108828.
Last updated: September 6, 2023