Part of a series of articles titled Alaska Park Science Volume 20 Issue 1 - Parks as Proving Grounds.
Article
Uncrewed Aerial Systems as a Tool for Natural Resource Applications
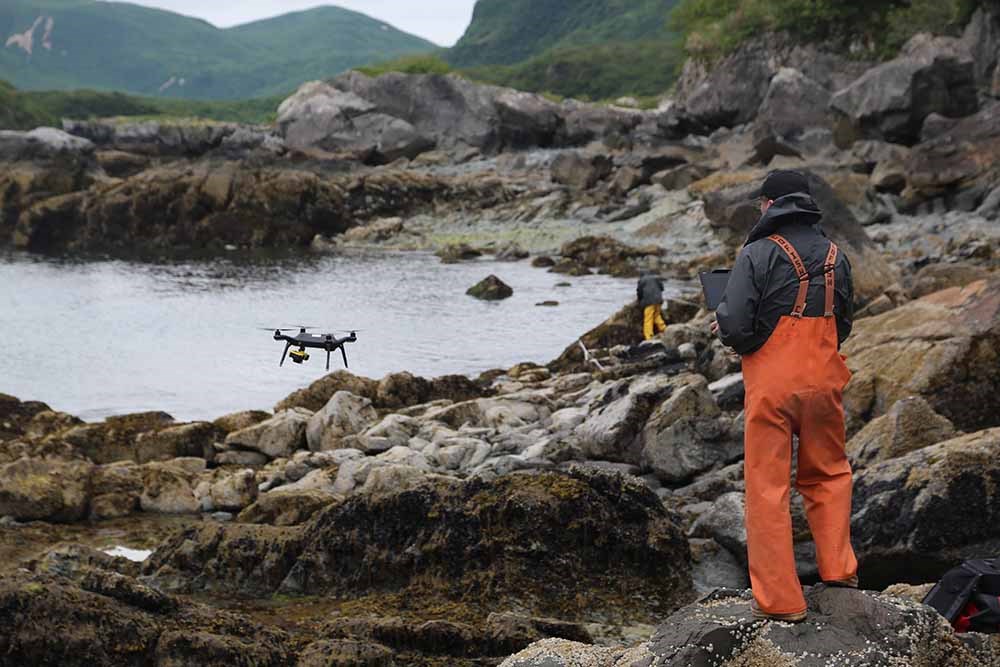
NPS PHOTO
Uncrewed Aerial Systems (UAS) have emerged as valuable tools for natural resource management, science, and geospatial applications. UAS are unoccupied aircraft that are controlled by a combination of a radio-linked interface and an onboard autopilot. UAS can be used in lieu of crewed aircraft to collect natural resource data, increase safety, reduce costs, and provide an aviation resource where crewed aircraft may not be appropriate, available, or able to fly. UAS missions can be accomplished through a variety of ways, including using fleet operations, contractors, and through partnerships with universities or federal and state agencies.
In June 2014, the National Park Service (NPS) Director issued Policy Memorandum 14-05, which outlined the use of UAS for administrative or research purposes. Since 2016, parks and NPS programs in Alaska have used UAS to inform science, natural resource monitoring, and provide park managers with information for decision making. The advantages of UAS technologies are especially promising in the NPS mission areas of inventory and monitoring (I&M), natural and cultural resource management, law enforcement, and search and rescue. By developing UAS capabilities in these areas, the NPS can inform decisions to better understand and protect park resources and values.
Benefits of UAS as a Tool
Platforms for collecting remotely sensed data include satellite, occupied aircraft, and UAS (Table 1). Factors to optimize platform usage depend upon the question of interest, geographic scale, and desired data product and resolution. Traditionally, occupied aircraft have been used to conduct surveys to collect relatively high-resolution data over large geographic areas. However, surveys that are conducted using occupied aircraft can pose significant safety risks for scientists and pilots, may be costly, and may have limited availability in many regions of Alaska. Depending on the scale of the research question and study area, UAS may be more cost-effective, particularly for projects that are limited in geographic scope. UAS may also provide the ability to assess aspects of animal behavior that are not feasible with occupied aircraft and potentially reduce disturbance to wildlife during surveys. In addition, UAS have the ability to fly at lower altitudes beneath the clouds (e.g., Sweeney et al. 2015), can be pre-programmed to conduct systematic surveys, and, in most cases, rely upon battery power as opposed to fossil fuels (DOI OAS 2015, Johnston 2019).
Platform | Multi-rotor UAS | Fixed Wing UAS | Crewed Aircraft | Satellite |
---|---|---|---|---|
Altitude | Surface to 1,200’ AGL | Surface to 12,000’ AGL |
100’ AGL to 15,000’ MSL |
> 200 miles AGL |
Area | 1-50 acres (single flight/day) |
1-640 acres | 1-1,000 sq. miles | > 1,000 sq. miles |
Typical Resolution (Ground Sample Distance) |
0.5”- 3” | 1”- 3” | 3”- 40” | > 6” |
Speed | slowest | slower | faster | fastest |
Examples of Applications | vertical 3D modeling, structural/habitat evaluations |
mapping | wildlife surveys, Lidar |
vegetation index, sea surface temperature and ocean color |
UAS Platforms and Sensors
The airborne system of uncrewed aircraft is composed of two primary components: a platform and a sensor. Platforms include multi-rotor, fixed-wing, or transitional aircraft that vertically take-off and land (VTOL). Multi-rotor UAS have the benefit of hovering; fixed-wing have the benefit of using lift generated by the airfoil as opposed to thrust (power), thus providing longer flight endurance; VTOL aircraft can launch and land in smaller areas and have the ability to hover over an area.
UAS can carry a variety of inexpensive commercial-off-the-shelf (COTS) payloads without requiring modification. Payloads can include, but are not limited to, mapping cameras, real-time high-definition (HD) video cameras, thermal-infrared sensors, multispectral cameras, Lidar sensors, and a variety of other data collection sensors (e.g., aerosol monitors; Table 2). Data resolution is a function of sensor lens, data capture rate, and flight altitude. Data streams can include video, digital images, and thermal images. Processed products can include orthomosaics, digital surface models, and time-series data.
Examples of Sensors | Optical | Thermal | Multi-spectral | Other Data Collection Sensors |
---|---|---|---|---|
Types | visual (red, green, blue) color-infrared (red, green, near-infrared) |
radiometric (longwave thermal infrared) | red-edge (red, green, blue, red-edge, near-infrared) | aerosol, temperature, particulate, UV, Lidar |
Resource Applications | wildlife, habitat | hydrology, geology, wildlife | botany, archaeology, habitat | volcanology, hydrology, air quality, wildlife, astronomy |
Products | digital image, orthomosaiced photos, live-stream video | orthomosaiced photos, live-stream video | orthomosaiced photos | time series, physical and biological samples |
Examples of Natural Resource Applications
Since 2016, UAS have been used as a tool in Alaska parks for a variety of physical and biological science related projects. The NPS UAS program has also supported all-hazard assignments, inter-agency projects, and continues to develop best practices for future UAS integration into parks to help managers determine safe, efficient, and effective ways to integrate UAS operations with park missions.
In Denali National Park and Preserve, multi-rotor UAS platforms have been used to map streams affected by mining activities for restoration and trail project planning, to generate elevation models for monitoring volumetric changes of mass wasting sites, and to create 3D models of historic districts to assess land instability due to permafrost melt, along with thermal imaging to identify ground temperatures. Denali National Park and Preserve has also used UAS to map permafrost and fire ecology sites with multi-spectral and thermal sensors to assess plant growth in burned areas, identify spatial patterns in permafrost thaw dynamics, and map elevation. UAS have also been used in partnership with the University of Alaska Fairbanks to map paleontological specimens in Denali National Park and Preserve and to conduct glacier mapping in Kenai Fjords National Park.
The NPS Inventory & Monitoring Program has conducted UAS surveys of the intertidal zone in Katmai and Lake Clark national parks and preserves in collaboration with Gulf Watch Alaska and the US Geological Survey-Alaska Science Center. The resulting data are being evaluated to assess intertidal species distribution and abundance and create multi-spectral orthomosaics for land cover and topographic change analysis along the coastline. A pilot project in Denali National Park and Preserve collected real-time video streams of nesting Canada jays to quantify chick health.
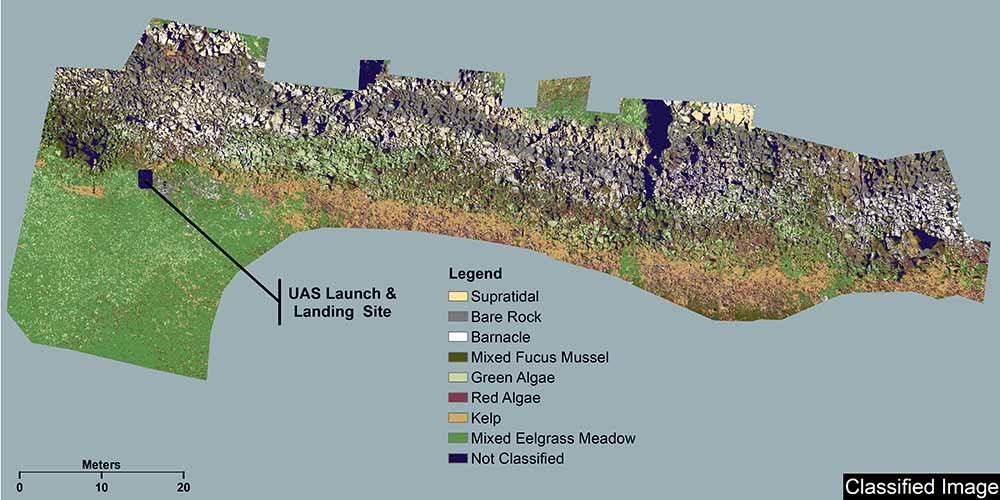
Future Applications
Several UAS projects are in the planning stages that will support natural resource applications in NPS units. For example, UAS technologies are expected to provide valuable information on the current condition and long-term trends of vegetation and other natural resources to determine how well management practices are sustaining park ecosystems. Through a partnership agreement with the US Forest Service, NPS fleet operations within the Alaska I&M Program will provide high-resolution baseline imagery for vegetation map accuracy assessments of forested areas in Alaska.
Population assessment surveys for terrestrial and marine wildlife species present unique challenges for transitioning to small UAS platforms, given the large geographic areas and remote regions in which they occur (e.g., Schmidt et al. 2017, Womble et al. 2020). Nonetheless, innovation in UAS platforms with longer battery endurance will have the ability to transform the field for wildlife population assessment over large geographic areas (Christie et al. 2016, Johnston 2019). Plans to map thermal refugia with infrared sensors and stream turbidity with multi-spectral sensors for hydrologic surveys, as well as detect fish presence with optical sensors, are planned for Denali National Park and Preserve. Trial surveys conducted by the US Fish and Wildlife Service and NPS for nest surveys of birds may hold promise for future NPS projects (Magness et al. 2019).
Transitioning from crewed aircraft to UAS platforms will substantially increase safety for biologists and pilots as wildlife surveys are typically low, slow, and often over water or ice, which presents substantial risks. There are plans for a forthcoming project to evaluate UAS as a platform for population assessment of harbor seals, sea otters, and their habitats with the goal of improving the safety and efficiency of future data collection efforts. This project will take advantage of recently developed statistical designs combined with aerial photographic methods that were developed in occupied aircraft (Williams et al. 2017, Womble et al. 2020). Additionally, aerial surveys for Dall’s sheep require flights in narrow box canyons in turbulent conditions and in close proximity to terrain. A UAS equipped with thermal and optical sensors may serve as an alternative platform and would reduce human exposure to aviation hazards.
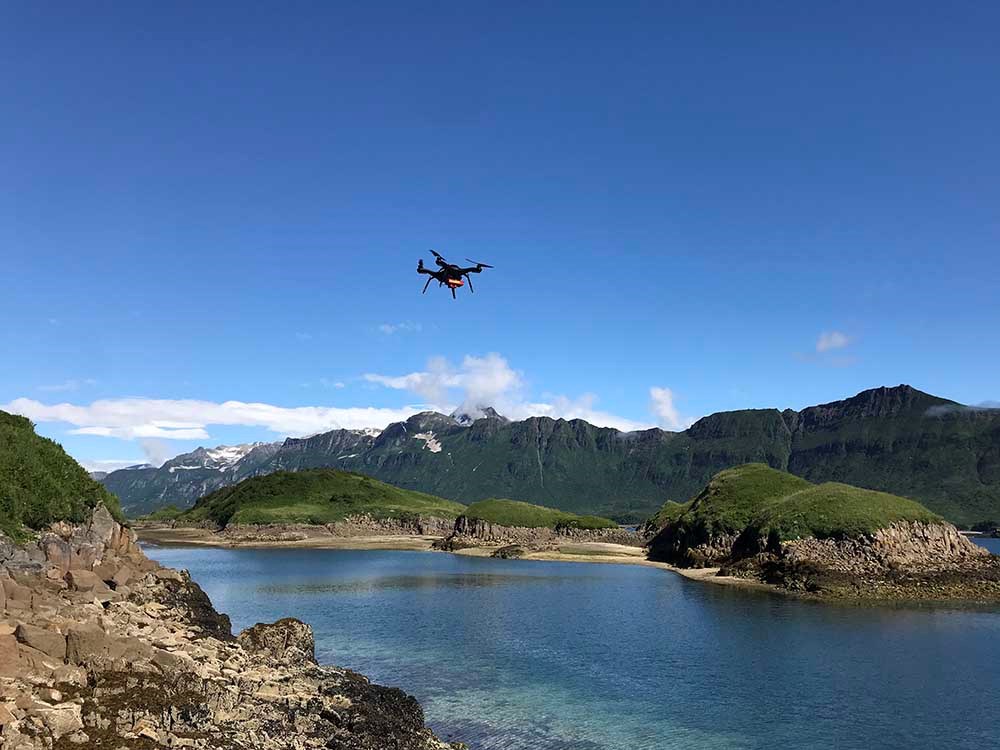
Challenges and Future Directions of UASs
Advances in UAS platform and sensor technology, regulatory and policy development, and a balanced integration of the tool provides future opportunities for Alaska parks. While there are many benefits of using UAS, the adoption of new technology also comes with challenges. Battery technology and endurance can be limiting; however, as innovation continues, battery capacity for longer flights should increase while also decreasing costs. For example, Department of the Interior call-when-needed contracted UAS are available with 16-hour flight times, while engineers are currently designing a quad-biplane for the National Oceanic and Atmospheric Administration (NOAA) with long-endurance (multi-day), heavy-payload, and agile flight response for atmospheric profiling and meteorological data collection.
UAS have exposed mechanical parts, making them more susceptible to rain and snow, cold temperatures, and wind. Smaller UAS are also limited in the weight they can carry, which typically means smaller sensor size and limited sensor resolution. UAS that provide weatherized and ruggedized bodies, as well as the ability to maintain radio signals at greater distances, are in the development and approval phases.
Similar to other natural resource projects, workflows that include identifying the question of interest and the appropriate tool, project planning, data collection, statistical analysis, data management, and publication are essential for success. Like satellite and crewed aircraft surveys, UAS surveys can generate large amounts of data and imagery and the post-processing workload can be time-consuming, technical, and costly. Properly accounting for the post-processing workload is essential and will require efficient workflows, data management, and technical expertise.
Continued research and development related to semi-automated or automated methods, such as machine learning, for automated image processing and detection of target species (e.g., Seymour et al. 2017) will be essential for improving the efficiency of post-processing. For example, NOAA Fisheries is currently leading an initiative with industry and academic partners to create end-to-end open-source software for automated analysis of optical data streams collected from vessels, occupied aircraft, and UAS for use in fisheries and marine mammal stock assessments (Angliss et al. 2020).
Federal regulations for UAS operations have evolved with the technology and use of UAS, and safe integration of UAS in the national airspace now allows for more opportunities to fly beyond line-of-sight. There are concerns related to potential impacts on wildlife, soundscapes, and visitor experience, as well as potential privacy or sensitivity incursions. For example, using UAS for monitoring and research of protected species, such as marine mammals, requires federal research permits from managing agencies. In addition, there are plans by the Department of the Interior (DOI) Office of Aviation Services (OAS) to evaluate “Blue” UAS, which would allow for enhanced cybersecurity for future DOI missions.
Use of UAS for natural resource monitoring will continue to benefit from collaboration with other agency, academic, and industry partners that have the ability to develop, test, and extend UAS capabilities and post-processing techniques for natural resource monitoring and research. The application of UAS in parks has helped define best practices, processes, and procedures for operations in Alaska national parks, as well as establishing interagency relationships with other DOI bureaus, and developing additional partnerships with local, state, federal and academic entities. By expanding existing aviation capabilities and integrating cost-efficient UAS as a survey platform, the NPS has access to a versatile tool for monitoring the status of natural resources in Alaska.
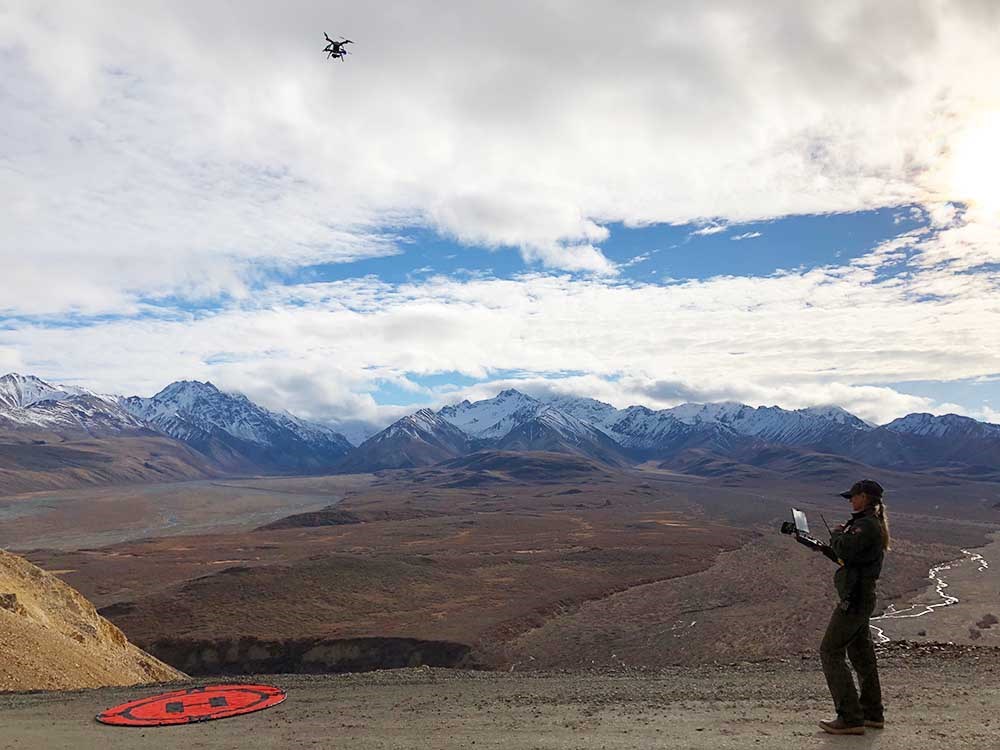
References
Angliss, R., K. Sweeney, E. Moreland, B. Hou, E. Richmond, C. Khan, B. Sanderson, S. Robinson, M. Lynn, and A. Martinez. 2020.
Report of the Image Processing Workshop. U.S. Dep. Commer., NOAA Tech. Memo. NMFS-AFSC-408, 77.
Christie, K. S., S. L. Gilbert, C. L. Brown, M. Hatfield, and L. Hanson. 2016.
Unmanned aircraft systems in wildlife research: Current and future applications of a transformative technology. Frontiers in Ecology and the Environment 14: 241-251.
Department of the Interior (DOI) Office of Aviation Services (OAS). 2015.
Department of the Interior Unmanned Aircraft Systems (UAS) Integration Strategy (2015-2020). Version 1.0. July 10, 2015.
Johnston, D. W. 2019.
Unoccupied aircraft systems in marine science and conservation. Annual Review in Marine Science 11: 439-63.
Magness, D. R., T. Eskelin, M. Laker, and H. M. Renner. 2019.
Evaluation of small unmanned aerial systems as a census tool for Aleutian Tern Onychoprion aleuticus colonies. Marine Ornithology 47: 11–16
Schmidt, J. H., T. L. Wilson, W. L. Thompson, and J. H. Reynolds. 2017.
Improving inference for aerial surveys for bears: The importance of underlying assumptions and the cost of unnecessary complexity. Ecology and Evolution 7:4812-4821.
Seymour, A. C., J. Dale, P. N. Hammill, P. N. Halpin, and D. W. Johnston. 2017.
Automated detection and enumeration of marine wildlife using unoccupied aircraft systems (UAS) and thermal imagery. Scientific Reports 7:45127.
Sweeney, K. L., V. T. Helker, W. L. Perryman, D. J. LeRoi, L. W. Fritz, T. S. Gelatt, and R. P. Angliss. 2015.
Flying beneath the clouds at the edge of the world: Using a hexacopter to supplement abundance surveys of Steller sea lions (Eumetopias jubatus) in Alaska. Journal of Unmanned Vehicle Systems 4(1):70–81.
Womble, J. N., J. M. Ver Hoef, E. A. Mathews, and S. M. Gende. 2020.
Calibrating and adjusting counts of harbor seals in a tidewater glacier fjord to estimate abundance and trends 1992-2017. Ecosphere 11(4): e03111.
Williams, P. J., M. B. Hooten, J. N. Womble, and M. R. Bower. 2017.
Estimating occupancy and abundance using aerial images with imperfect detection. Methods in Ecology and Evolution 8: 1679-1689.
Last updated: October 8, 2024