Part of a series of articles titled Alaska Park Science Volume 20 Issue 1 - Parks as Proving Grounds.
Article
Clues from Glacier Debris: Dating and Mapping Glacial Deposits Since the Last Ice Age in the Western Alaska Range
Jason P. Briner, University at Buffalo
Nicolas E. Young, Columbia University
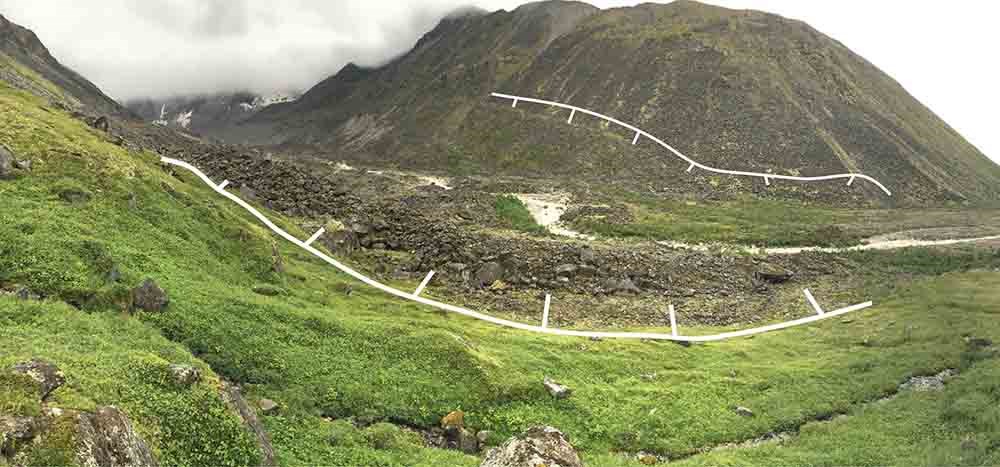
University at Buffalo/Jason P. Briner
During the cold times of the last ice age—roughly 26,000-19,000 years ago (Clark et al. 2009)—glaciers in Alaska and elsewhere accumulated snow, growing to tremendous size, spilling out of mountain ranges and into adjacent lowlands. Ice-age glaciers worldwide, although now gone or reduced in size, left behind signs of their former selves including moraines.
Glaciers act like bulldozers. Moraines are the piles of glacial debris (fine sediments like sand and mud, and large sediments like boulders) that were collected, transported, and deposited by glaciers. Moraines are features easily identified from the ground, on topographic maps, and from aerial images. Sometimes narrow, sometimes broad and lumpy, moraines are ridges of glacial debris draped over the landscape. For glacial geologists, moraines are an exciting archive of past glacier change, full of possibilities. Moraines are the footprint of past glacier positions and, if the age of the moraine is known, they can record the timing and rate of glacier change. In turn, carefully reconstructed glacier histories are used as archives of past climate change since glacier growth and decay are so closely coupled to climatic factors such as temperature and precipitation.
Here, we describe one cutting-edge technique for dating moraines, the challenges associated with using the technique, and how we are applying the method to a promising site in Alaska: the Revelation Mountains. Lessons learned from studying glacial deposits in the Revelation Mountains are valuable for understanding the glacial history of nearby parks and throughout Alaska more generally. Together with the iconic landscapes preserved in frequently visited parks like Denali, our research results can provide park visitors with important geologic context for currently retreating glaciers.
Evidence of past glacier advances throughout Alaska is abundant and has been noted for decades. In 1964, Thor Karlstrom published one of the first state-wide maps of surficial geologic deposits—including moraines—in Alaska. The map was a culmination of decades of careful and dedicated work by over 25 Alaska state geologists (Karlstrom 1964). Yet some of the earliest recorded observations and links between glaciers and the deposits they leave on the landscape date as far back as 150 years ago in Alaska (Blake 1867, Meehan 1884). Since that time, generations of glacial geologists have improved our understanding of the glacial record in Alaska through collaborative projects, workshops, seminars, and hundreds of scientific reports, papers, and maps. Maps of past glacier size across Alaska (e.g., Figure 1) are kept up to date (e.g., Kaufman et al. 2011) and made available for widespread use.
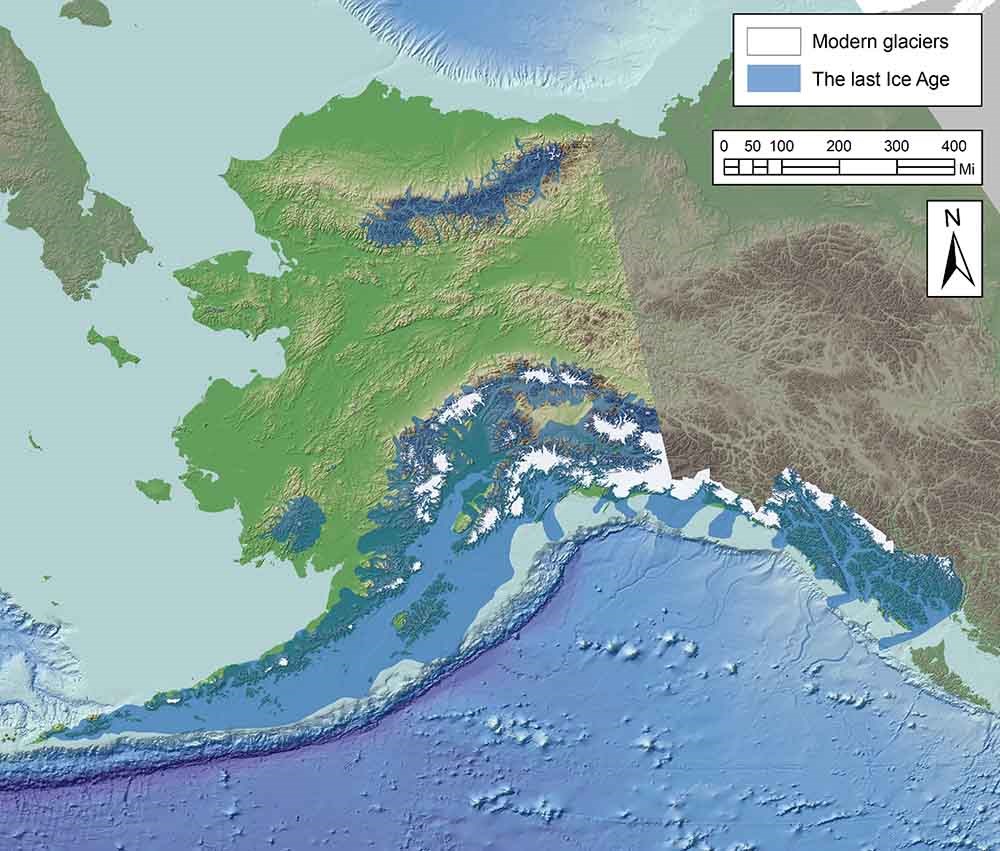
Alaska PaleoGlacier Atlas
Past Glaciers and Climate
Evidence for multiple glacier advances occurring over the last ~2.5 million years exist all over the state of Alaska (e.g., Kaufman et al. 2011). Some of the best-preserved and, therefore, most easily observable deposits were formed during and following the last ice age (spanning in age from approximately 20,000 years ago to less than a few hundred years old). During the last ice age, temperatures in Alaska may have been 2-4 degrees (Celsius) colder than today (Viau et al. 2008, Kurek et al. 2009), and glaciers covered roughly 42% of Alaska (Kaufman 2011; Figure 1). Compare that to the roughly 3.5% of Alaska that is covered by glaciers today (Kienholz et al. 2015; Figure 1). Between the last ice age and present, from 19,000 to 11,000 years ago, the global climate warmed and glaciers in Alaska (and worldwide) underwent a period of substantial retreat. This episode of glacier retreat is referred to as the last deglaciation and is a key period in Earth’s history. The last deglaciation provides glacial geologists with a natural experiment to see how glaciers behave when global climate warms significantly. Determining how glaciers responded to climate warming in the past provides essential insight into how glaciers will respond to climate warming today and in the future.
The key to reconstructing glacier histories is two-fold: (1) there needs to be meticulous mapping of glacial deposits to know where glaciers have been, and (2) there needs to be precise dating of those mapped glacial features to know when glaciers formed the deposits. Opportunities for continued glacial geologic mapping improvements arise as high-quality satellite images and geographic maps are frequently published. Examples include the Arctic Digital Elevation Model provided by the Polar Geospatial Center, which we used to make some of the maps in this paper. However, dating glacial deposits in the state, and worldwide, has only recently been seriously attempted (in the last 50 years or so). Furthermore, dating techniques for glacial deposits need to be exceptionally precise to make meaningful comparisons between past glaciers and other climate archives (such as ice core records from Antarctica and Greenland, ocean sediment records, and lake sediment records, among other archives), and it has only been in the last few decades that advancements to new dating techniques have made those comparisons possible.
Glacial geologists now use a technique known as cosmogenic isotope exposure dating to date moraines deposited during the last deglaciation and we have applied this technique in the Revelation Mountains of the western Alaska Range. This dating technique has been applied with varying degrees of success to other sites in Alaska—including Denali National Park and Preserve—(see Kaufman et al. 2011). Yet after two decades of research across the state, paired with continued improvements to field sampling and lab techniques, we believe that the Revelation Mountains site will provide the best chance of reconstructing a precise and complete post-ice age history of glaciers anywhere in the Alaska Range. The ultimate goal of this research is to use the information gained from studying past glaciers in the Alaska Range to improve our understanding of how and why glaciers in Alaska are responding to current climate change.
Cosmogenic Exposure Dating
Our planet is constantly bombarded with high-energy particles that originate from outside of our solar system, known colloquially as cosmic rays. These charged particles enter Earth’s atmosphere with enough velocity that they strike gas atoms in our atmosphere and burst them apart, like a cue ball breaking racked balls at the start of a pool game. There is so much energy from cosmic rays entering our atmosphere that a giant chain reaction of atoms bursting apart and colliding with other atoms makes its way down to shower the Earth’s surface. At this point, there is still enough remaining energy that particles in the atmosphere penetrate Earth’s crust and burst apart some atoms that make up the minerals in rocks. The leftover pieces of atoms become new isotopes (a term used to distinguish atomic elements with a varying number of neutrons within their nucleus), referred to as cosmogenic isotopes. These cosmogenic isotopes accumulate over time in the surfaces of rocks exposed at Earth’s surface at a relatively steady rate. The longer rock surfaces are exposed, the greater the accumulation of isotopes.
For cosmogenic isotopes to be a useful dating tool, there needs to be some sort of geologic event that exposes fresh rock surfaces from deep below Earth’s surface so that cosmogenic isotopes may begin accumulating on that surface. In other words, there needs to be a “clock starter.” Conveniently for glacial geologists, glaciers grind off previously exposed parts of Earth surface and expose new, fresh rock surfaces that do not contain any cosmogenic isotopes. Glaciers produce beautiful landscapes by carving broad valleys and fjords in mountain ranges, like so many of the iconic landscapes found in Alaska. To form moraines, glaciers collect and transport large boulders and sediments that are both plucked from below the ice and that fall from the steep, carved valley walls onto the glacier. In many cases, these sediments and boulders were not previously exposed to the surface prior to being collected by the glacier. Thus, once the glacier forms a moraine or retreats out of a mountain valley, fresh sediments and bedrock are exposed and the clock starts. In these new surfaces, cosmogenic isotopes begin accumulating and the isotopes on the rock surfaces build. After a long period of time, glacial geologists can collect surface samples from bedrock or from boulders sitting atop moraines and measure the amount of cosmogenic isotopes in those surfaces. From that information, we can calculate the time at which the glacier left those moraine boulders and bedrock surfaces behind by applying known rates of cosmogenic isotope production.
Challenges
There are a certain set of requirements when using cosmogenic isotopes to date moraines. In an ideal situation, a glacier would (1) build a moraine using freshly scraped boulders and other sediments, (2) leave that moraine exposed at the surface once it retreated, and (3) that moraine would then remain perfectly intact for millennia until a glacial geologist collects a sample (Figure 2A). However, glaciers do not always adequately grind down rock surfaces. In some regions on Earth (although not commonly in Alaska), glaciers move very slowly and fail to erode away all of the previously exposed rock surfaces. In this case, there might be leftover cosmogenic isotopes in rock surfaces. Extra cosmogenic isotopes in these rock surfaces would mean that ages appear to be older-than-expected (i.e., inheritance; Figure 2B).
Landscapes on Earth rarely remain perfectly preserved and, in fact, moraines slowly degrade through time. During that process, boulders sitting on top of moraines sometimes tumble over or rise from inside the moraine as mud washes away around them in a process known as boulder exhumation. These processes alter or delay the start of the cosmogenic clock and result in an incorrect age of moraine formation. The ages derived from these boulders appear to be younger than expected (Figure 2C).
The Alaskan landscape is exceptionally dynamic. Active faulting that causes earthquakes and land-slides, the freeze-thaw cycle of the active layer in permafrost, and even volcanism all lead to enhanced erosion and degradation of relatively fragile land-forms like moraines. Thus, moraine degradation is a serious issue for glacial geologists wishing to use cosmogenic isotope exposure dating in Alaska. For this reason, although there have been many studies using cosmogenic isotopes in Alaska, results from moraine dating studies in Alaska vary.
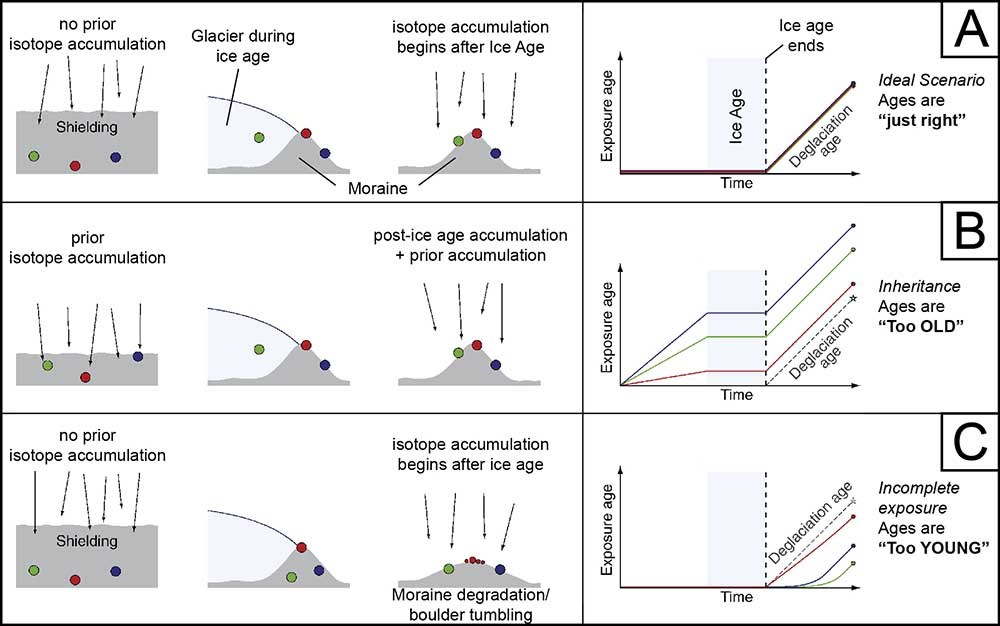
The glacier is sufficiently erosive, but after deposition, the moraine degrades, resulting in ages that are too young. [Figure modified from Heyman et al. 2011.]
Not all moraines are created equally. Typically, moraines contain a mixture of both large- and fine-grained sediments (e.g., Figure 3A). However, the composition of a moraine can range from almost completely boulder-sized sediments (i.e., clast-supported moraine; Figure 3B) to almost completely clay or mud-sized sediments (i.e., matrix-supported moraine; Figure 3C). While the rate of moraine degradation is dependent on environmental factors, the rate is also dependent on moraine composition. In other words, a moraine is more likely to degrade if it is mostly composed of fine-grained sediments instead of being mostly composed of large boulders. This has to do with the fact that fine sediments, like clays, trap ice (Figure 3C) and water. If the ice trapped inside a moraine were to melt, the moraine would not hold its shape but would slump or settle out (like the moraine depicted in Figure 3C). In contrast, moraines mostly built of large boulders do not trap ice very well and are less affected by slumping due to ice melt-out. That said, it is extremely valuable to find moraines on landscapes that appear to be more clast-supported (Figure 3A and 3B) than matrix-supported (Figure 3C), for it is these types of moraines that provide the best chance to sample boulders that have been stable throughout time. Ages from clast-supported moraines are more likely to represent the true date of moraine deposition.
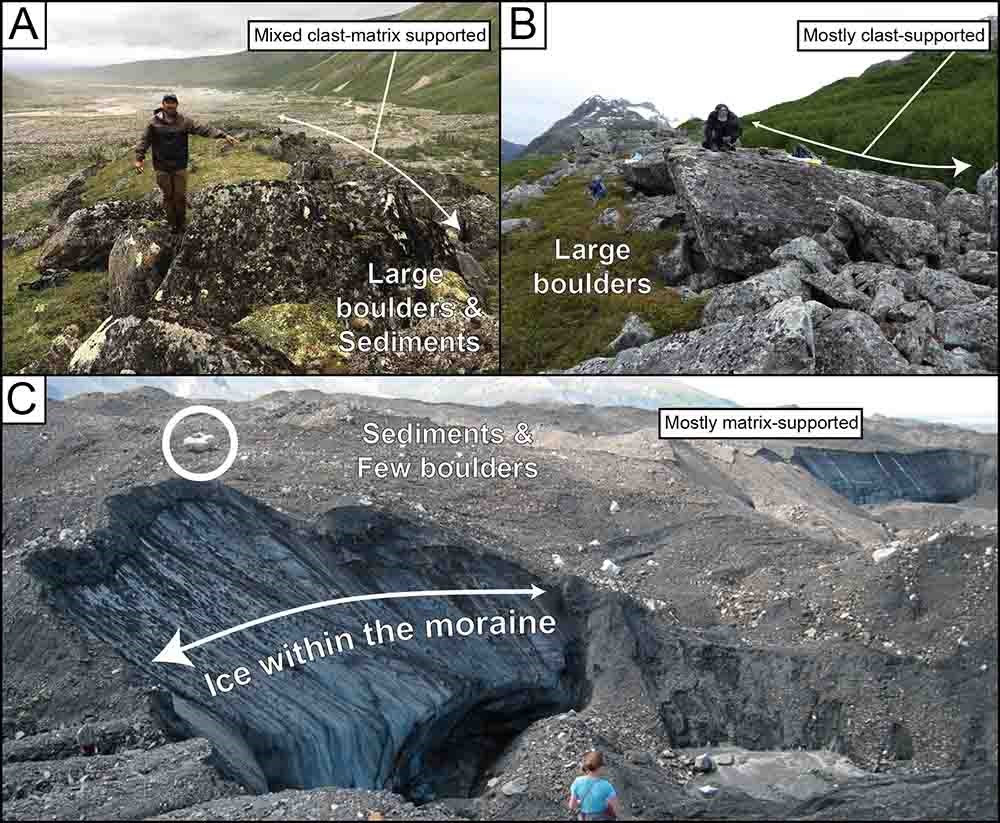
Note also the presence of glacier ice trapped within the moraine that will likely eventually melt out and cause the moraine to degrade even further. This ice is often referred to as “dead ice” and can result in erroneous moraine ages.
For the past few decades, since near the time when cosmogenic isotope exposure dating was first applied to moraines in California (Phillips et al. 1990), glacial geologists have been attempting to use the method on moraines in Alaska (Kaufman et al. 2011). There have been a few successful attempts, but there have also been failures. Both have been critical to further our understanding. It has been only through these past attempts that glacial geologists have learned the importance of meticulously selecting moraines for dating before collecting samples. For example, moraines deposited in Denali National Park and Preserve by the Muldrow Glacier have significantly degraded because they are mainly matrix-supported, with only a few large boulders sprinkled throughout (Figure 3C). In addition, these moraines are located near the Denali Fault and may have degraded over time in response to the steady occurrence of powerful earthquakes. For these reasons, previous work has shown that cosmogenic isotope exposure ages from Denali National Park and Preserve likely do not represent the time when the glacier built the moraine, but rather multiple phases of moraine stabilization after the glacier had already significantly retreated (Dortch et al. 2010). While the popular landmark that attracts visitors worldwide is a stunning visual of how glaciers shape landscapes, glacial geologists have found that other sites in Alaska are more suitable for cosmogenic exposure dating.
The Revelation Mountains
Despite the previously mentioned challenges, some sites in Alaska do exhibit properties that more accurately record the timing of moraine formation. Glaciers existed in the Revelation Mountains during and following the last ice age, and the moraines these glaciers formed are preserved on the landscape. In the early 2000s, Dr. Jason Briner and his colleagues Dr. Darrell Kaufman, Dr. Al Werner, and others visited multiple sites across Alaska, including a site known as the Swift River Valley in the Revelation Mountains. They were searching for the ideal site to generate a precise glacier chronology for Alaska (Briner et al. 2005). This reconnaissance work produced some promising, yet incomplete, results. They found that unlike the moraines in Denali and elsewhere in Alaska, the moraines in the Revelation Mountains were less degraded and contained many large, stable boulders with moraines that were not predominantly matrix-supported.
Beginning in 2016, following more than a decade of continued research in Alaska, and improvements to both sampling and lab techniques by the global community of glacial geologists, our team revisited the Swift River Valley. The goal was to generate a reliable chronology of the oldest moraines from the last ice age to both demonstrate a successful application of cosmogenic exposure dating in Alaska and compare glacier change in Alaska to past climate. In the example shown in Figure 4, we found one moraine that was likely deposited sometime around 17,800 years ago based on four reliable exposure ages (note the two outliers that are significantly older than the rest; we suspect those are samples affected by inheritance). The rest of the moraine ages from that site may be found in Tulenko and others (2018). Since we were able to produce a well-constrained dataset from a collection of stable moraine boulders, we were able to discuss how Alaska’s glacier history related to climate change. We found that even though global temperature and atmospheric carbon dioxide (CO2) concentrations remained relatively low toward the end of the last ice age, glaciers in Alaska began retreating prior to some other glaciers around the world. We suggested this warming was due to steadily increasing solar radiation in the Arctic. However, the dataset we produced is incomplete; we still do not know what happened to the glaciers in the Revelation Mountains through the last deglaciation. Was this relatively early retreat at the end of the last ice age sustained?
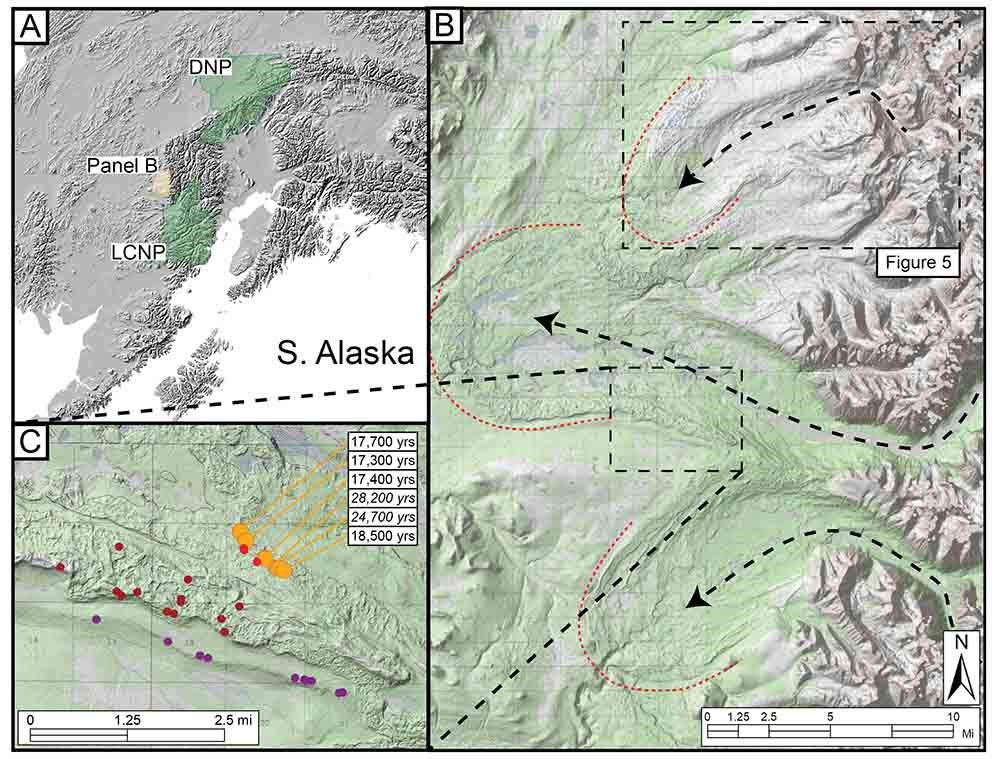
(C) Zoomed image of lateral moraines lining the Swift River Valley: All samples collected in 2016 denoted by colored dots and an example of boulder ages on one moraine sampled in 2016 (four reliable ages and two ages suspected of showing inheritance).
In the summer of 2019, we visited the Revelation Mountains once again. This time we had a second goal: to investigate the detailed retreat of a glacier following the last ice age. Our specific study glacier in the north Swift River Valley deposited multiple discrete moraines since the last ice age, and to date them, we collected surface samples from 79 boulders (Figure 5). Based on moraine mapping and the chronology generated from our 2018 paper, we hypothesize that these moraines were deposited sometime between the last ice age and today, with many deposited during the last deglaciation interval. As with the site we visited in 2016, we hope we will be able to precisely date these moraines. This time however, we hope to use the chronology to characterize the rate of retreat of this glacier through the last deglaciation. This will allow us to make a direct comparison between our glacier chronology and other climate records to determine exactly why the glacier—and glaciers across Alaska—retreated after the last ice age.
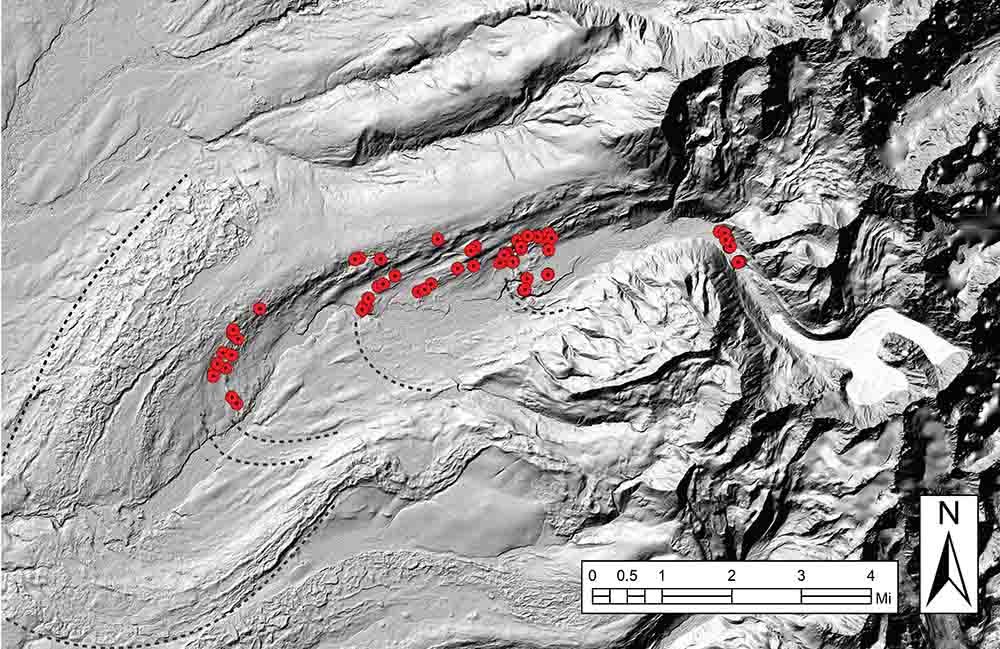
[Hillshade map created from data freely available from the Polar Geospatial Center’s ArcticDEM product.]
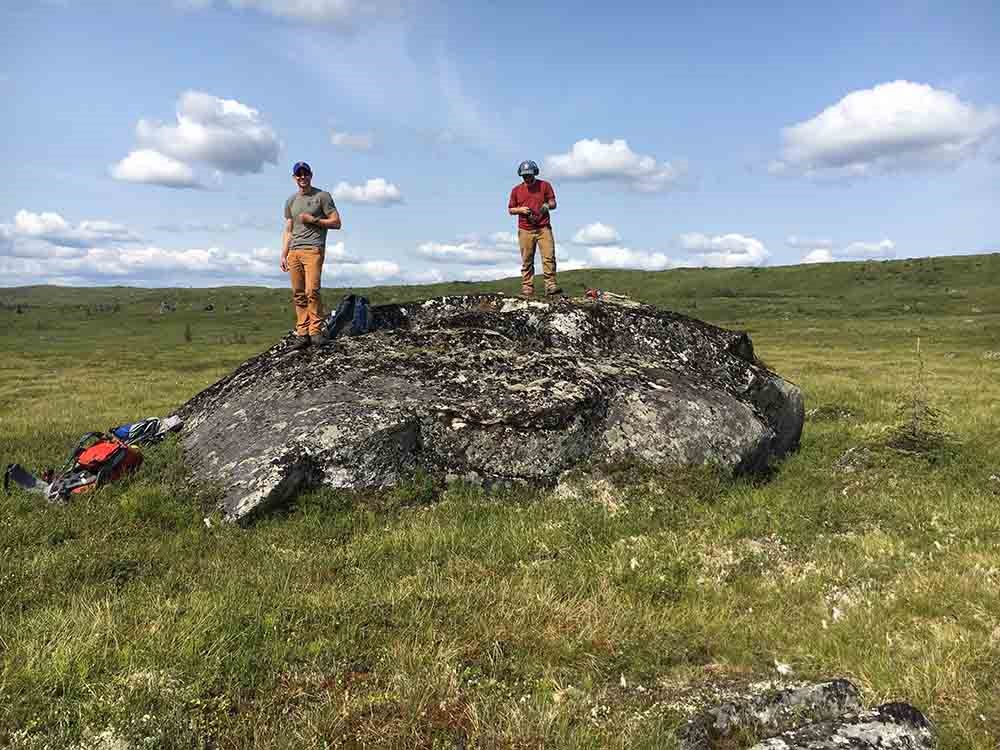
University at Buffalo/Jason P. Briner
Summary and Future Project Directions
Although our work is ongoing, we hope to soon contribute data to help answer several big questions in the climate science community:
- Is there one single mechanism of climate change (such as greenhouse gases) that controls how glaciers behave worldwide, or are Arctic glaciers different?
- The last deglaciation was one of the most recent times in the geologic record that the climate warmed quickly. How fast did glaciers in Alaska melt away during this interval?
- Do these past changes provide some useful context and comparison to how quickly glaciers are currently retreating, and will continue to retreat, as the climate is once again warming rapidly?
There are precise records of glacier retreat during the last deglaciation for many sites across the globe, but not yet in Alaska. With this project, we aim to generate a retreat chronology with precision comparable to other chronologies elsewhere to determine if alpine glaciers in the Arctic behaved similarly to glaciers in other regions and what climatic factors caused these similarities or differences. We hypothesize that lessons learned from studying interactions between glaciers and climate of the past will provide valuable context for current and future warming and glacier retreat. Visitors to parks across Alaska are able to see firsthand how quickly glaciers are retreating. It is our hope that providing some geologic context will demonstrate why current glacier retreat is so alarming.
References
Blake, W. P. 1867.
The glaciers of Alaska, Russia, America. American Journal of Science (130): 96-101.
Briner, J. P., D. S. Kaufman, W. F. Manley, R. C. Finkel, and M. W. Caffee. 2005.
Cosmogenic exposure dating of late Pleistocene moraine stabilization in Alaska. Geological Society of America Bulletin 117(7-8): 1108-1120.
Clark, P. U., A. S. Dyke, J. D. Shakun, A. E. Carlson, J. Clark, B. Wohlfarth, . . . A. M. McCabe. 2009.
The last glacial maximum. Science 325(5941): 710-714.
Dortch, J. M., L. A. Owen, M. W. Caffee, and P. Brease. 2010.
Late Quaternary glaciation and equilibrium line altitude variations of the McKinley River region, central Alaska Range. Boreas 39(2): 233-246.
Heyman, J., A. P. Stroeven, J. M. Harbor, and M. W. Caffee. 2011.
Too young or too old: Evaluating cosmogenic exposure dating based on an analysis of compiled boulder exposure ages. Earth and Planetary Science Letters 302(1): 71-80.
Karlstrom, T. N. V. 1964.
Surficial geology of Alaska (357). Available at: http://pubs.er.usgs.gov/publication/i357 (accessed February 1, 2021)
Kaufman, D. S., N. E. Young, J. P. Briner, and W. F. Manley. 2011.
Alaska Paleo-Glacier Atlas (Version 2). In J. Ehlers, P. L. Gibbard, & P. Hughes (Eds.), Quaternary Glaciations - Extent and Chronology (Vol. 15). Amsterdam, Netherlands: Developments in Quaternary Science.
Kienholz, C., S. Herreid, J. L. Rich, A. A. Arendt, R. Hock, and E. W. Burgess. 2015.
Derivation and analysis of a complete modern-date glacier inventory for Alaska and northwest Canada. Journal of Glaciology 61(227): 403-420.
Kurek, J., L. C. Cwynar, T. A. Ager, M. B. Abbott, and M. E. Edwards. 2009.
Late Quaternary paleoclimate of western Alaska inferred from fossil chironomids and its relation to vegetation histories. Quaternary Science Reviews 28(9): 799-811.
Meehan, T. 1884.
Notes on glaciers in Alaska. Philadelphia Academy of Natural Sciences Proceedings 1883.
Phillips, F. M., M. G. Zreda, S. S. Smith, D. Elmore, P. W. Kubik, and P. Sharma. 1990.
Cosmogenic chlorine-36 chronology for glacial deposits at Bloody Canyon, eastern Sierra Nevada. Science 248(4962): 1529-1532.
Tulenko, J. P., J. P. Briner, N. E. Young, and J. M. Schaefer. 2018.
Beryllium-10 chronology of early and late Wisconsinan moraines in the Revelation Mountains, Alaska: Insights into the forcing of Wisconsinan glaciation in Beringia. Quaternary Science Reviews 197: 129-141.
Viau, A., K. Gajewski, M. Sawada, and J. Bunbury. 2008.
Low-and high-frequency climate variability in eastern Beringia during the past 25,000 years. Canadian Journal of Earth Sciences 45(11): 1435-1453.
Young, N. E., J. P. Briner, J. Schaefer, S. Zimmerman, and R. C. Finkel. 2019.
Early Younger Dryas glacier culmination in southern Alaska: Implications for North Atlantic climate change during the last deglaciation. Geology 47(6): 550-554.
Last updated: May 18, 2021