Part of a series of articles titled Alaska Park Science Volume 20 Issue 1 - Parks as Proving Grounds.
Article
Using Aquatic Invertebrates to Measure the Health of Stream Ecosystems: New Bioassessment Tools for Alaska’s Parklands
Trip Armstrong, Bureau of Land Management
Charles P. Hawkins, Utah State University
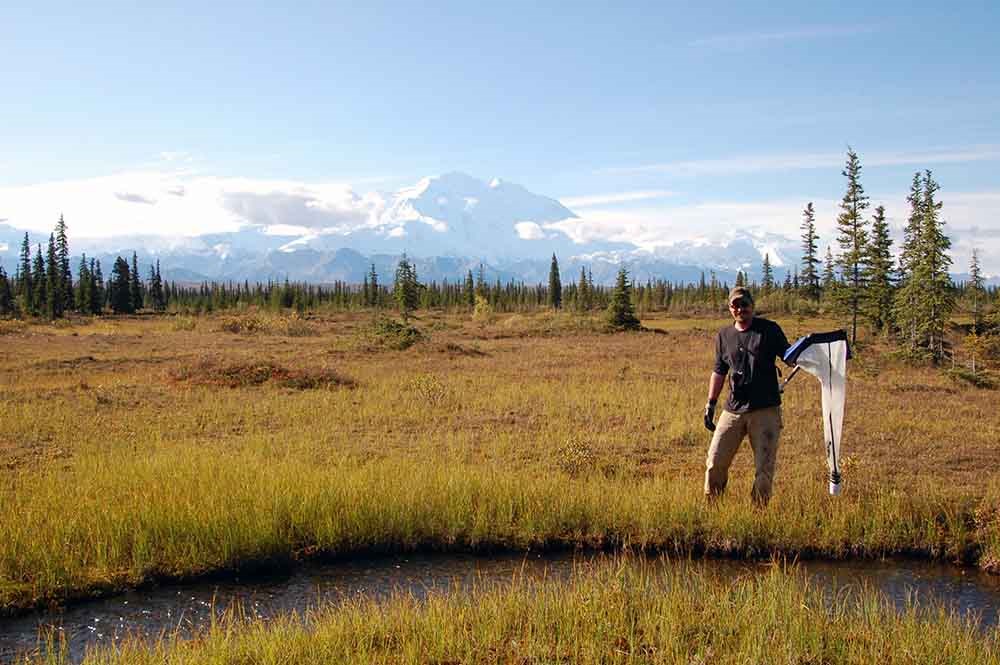
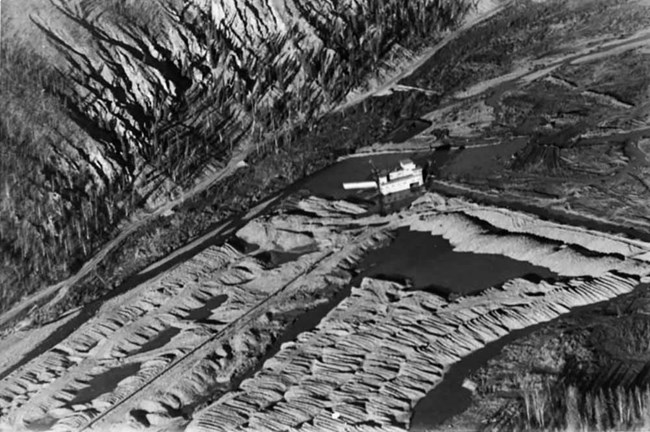
Although the popular image of Alaska and its national parks is one of vast tracts of unspoiled wilderness, in fact, human disturbance has had significant impacts on stream ecosystems in many areas, particularly in interior Alaska. Over a century of mining, primarily for gold, has been the main source of this disturbance. Mining took place in all three interior Alaska parks (Denali National Park and Preserve, Wrangell-St. Elias National Park and Preserve, and Yukon-Charley Rivers National Preserve) as well as in much of the surrounding area. Although mining in the parks largely ended in the 1980s, some activity continues to this day, and mining continues in many other areas. In some cases, hydraulic dredge mining has led to drastic alteration of entire valleys (Figures 1 and 2), whereas other impacts to mined streams have been smaller and more localized. Mining activity may lead to a variety of detrimental changes to stream ecosystems, including habitat simplification, removal of riparian vegetation, channelization, altered hydrology, excessive sedimentation, acidification, and increased levels of toxic compounds such as heavy metals (Bernhardt and Palmer 2011, Brim Box and Mossa 1999). Any or all of these changes may negatively affect the biological communities that live in streams, and lead to impairment of ecosystem function. Biological responses to human disturbances like mining may include changes in biodiversity, food web structure, productivity and nutrient cycling (Wagener and LaPierrere 1985, Van Nieuwenhuyse and LaPerriere 1986, Wedemeyer 1987, Niyogi et al. 2002, Maret et al. 2003, Daniel et al. 2004, Milner and Piorkowski 2004). Other types of human disturbance such as roads, logging, urbanization, and agriculture can impact the biodiversity and function of stream ecosystems in similar ways. The authors and others have been working on developing methods to allow us to quantify the effects of these disturbances on the ecological integrity of streams throughout interior Alaska, including in national parks.
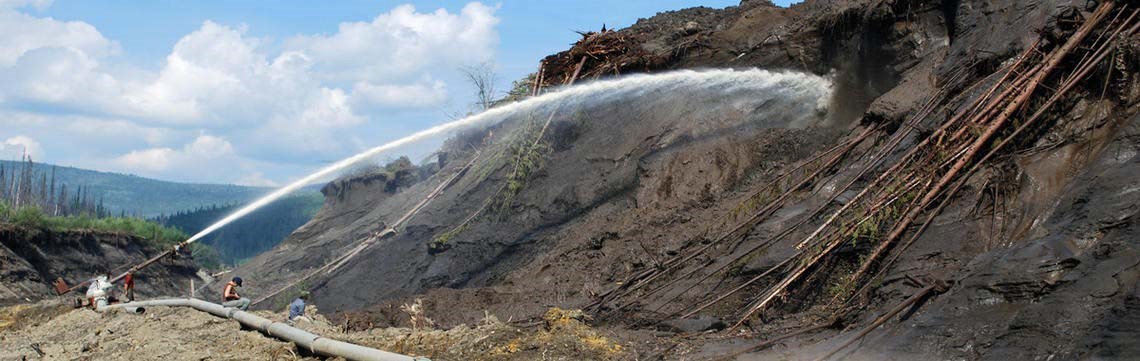
U.S. Geological Survey
There are several reasons why it is important to be able to quantify these effects on the integrity of stream ecosystems. In parks, the National Park Service is mandated to protect, and improve if necessary, park resources, which includes streams and the organisms that depend on them (National Park Service 2006). As part of this mandate, it is important that national park managers be able to understand the effects of both past human activity and current management actions on the integrity of park ecosystems, including streams and rivers. In addition, the core objective of the Clean Water Act of 1972 is to “restore and maintain the chemical, physical, and biological integrity of the nation’s waters” (USEPA 1972). The Clean Water Act requires that states report on the physical, chemical and biological condition of their waterbodies, determine whether they meet the designated use criteria that the state has established, and develop plans to improve the condition of any that are deemed to be impaired. In order to do so, states must develop defensible methods for determining whether waterbodies meet the criteria they have established. Finally, the ability to quantify ecological integrity will be critical for both prioritizing disturbed sites for restoration and for monitoring the degree to which those restoration efforts succeed in the future.
Historically, water quality assessment focused on the chemical aspects of water quality; for example, whether concentrations of nutrients or toxic compounds exceeded levels thought to be safe for aquatic life or human consumption. However, starting in the 1980s water quality practitioners began to move away from strictly chemical measures, and to attempt to consider a more holistic approach that also included measures of biological and physical integrity, as originally spelled out in the Clean Water Act. Methods that use various measures of the status of the biological community as indicators of the health of stream ecosystems have been the predominant focus of these more holistic ways to assess water quality (although similar methods are used to assess lake and wetland ecosystems, this article is focused on stream applications). The fundamental idea behind this approach is that the biological community reflects overall ecological integrity—that is, when the biological components of an ecosystem are healthy, then the physical and chemical components are also in good condition. These methods are collectively referred to as biological assessment, or bioassessment, and are in widespread use by water quality agencies around the world (Wright et al. 2000, Bailey et al. 2004, Carter and Resh 2013).
There are a number of reasons why assessing the status of the organisms that inhabit a stream or river (collectively referred to as the biota) is a powerful approach to determining the condition of a stream ecosystem. The primary advantage is that the biota is always present in the ecosystem. That means that it integrates conditions over time, and can respond to intermittent effects (for example, pulses of toxic compounds released during high flows) that may be missed by typical periodic sampling efforts. Similarly, the biota can respond to the chronic effects of low levels of stress, to stressors that we can’t easily measure, and to the aggregate effects of multiple stressors. Finally, the biota integrates conditions over space, in that it can respond to conditions or stressors upstream of the sampled site, as well as in the riparian and upland zones surrounding the site. We can use this approach to quantitatively assess the ecological condition of a stream site, allowing us to determine whether human activity has in fact had a negative impact on that ecosystem, and to potentially identify the particular stressors responsible.
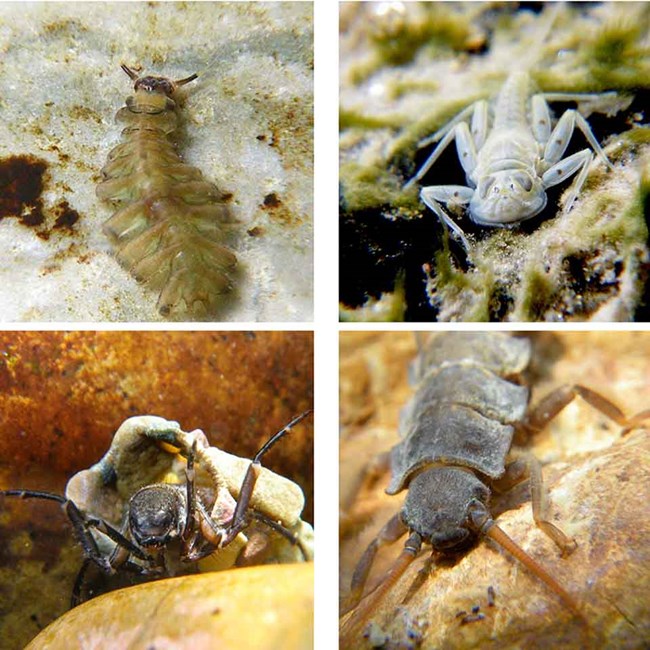
U.S. Geological Survey/Joe Giersch
Assessment approaches that use biological indicators to measure stream ecosystem condition have been in use for over a century (Kolkwitz and Marsson 1908, Metcalfe 1989), but in recent years have become much more widely applied and grown increasingly sophisticated. In principle, a bioassessment could be based on measuring the condition of any species assemblage that is present in a stream, including aquatic macroinvertebrates, fish, amphibians, algae, or aquatic macrophytes, or multiple assemblages in combination. However, the vast majority of these methods in fact rely on assessing aquatic macroinvertebrates (Figure 3). There are several reasons why macroinvertebrates are the preferred indicators of stream ecosystem health. First, they are ubiquitous, being found in every stream and river, no matter how small or extreme, meaning that macroinvertebrate-based assessments can be applied universally. They are also typically abundant and diverse in streams, making them relatively straightforward and cheap to collect and analyze. In Alaska, as many as 70 unique macroinvertebrate taxa (aquatic macroinvertebrates can often only be identified to the genus or family level; so we refer to taxa rather than “species”) may be present at a single stream site, and densities may be as high as 20,000 individuals per square meter. Due to this diversity, the macroinvertebrate assemblage as a whole is sensitive to a wide variety of potential stressors. Because macroinvertebrates typically live no more than a year or two, they tend to respond to the current state of the ecosystem. And finally, macroinvertebrates are relatively sessile; that is, they don’t tend to move long distances during their lifetimes, meaning that they are responding to local conditions (whereas salmon, for example, may be responding to changing conditions in the ocean rather than at a site we are interested in assessing).
Although a wide variety of methods exist for using macroinvertebrates to assess stream ecological integrity, there are two main approaches, both of which use the reference condition approach (Bailey et al. 2004, Stoddard et al. 2006), in which the status of the macroinvertebrate community observed at the streams being evaluated for the effects of human disturbance is compared to the status observed at unimpaired stream sites (sites with no or minimal human activity), which are referred to as reference sites (Stoddard et al. 2006, Herlihy et al. 2008, Hawkins et al. 2010). The conditions observed at these reference sites are used to set expectations for sites to be assessed. Although in many parts of the world identifying relatively pristine streams to be used as reference sites can be challenging, in Alaska it is relatively straightforward. Because macroinvertebrate assemblages naturally vary considerably among streams in response to local and regional environmental drivers, the reference sites must be properly classified so that the biological potential at each assessed site can be accurately determined by comparison to the appropriate subset of reference sites.
The first of these common bioassessment methods uses data about the environmental setting (for example, elevation, mean annual precipitation, basin area) and the invertebrate taxa observed at a large number of reference sites in a given area to develop predictions about the distribution of native invertebrates in response to environmental gradients (Wright et al. 2000, Clarke et al. 2003, Carlisle and Hawkins 2008). These predictions can then be used in combination with data on the environmental setting that describe a site we want to assess to predict the native invertebrate community that would be expected to be present at that site in the absence of human disturbance (i.e., if that site were, in fact, of reference site quality). Divergence away from those expected conditions, expressed as a percentage loss of predicted native taxa, indicates a loss of biological integrity and suggests that the site may be impaired by human disturbance. This divergence can be quantified using a simple index, O/E, which is the ratio of the number of expected native taxa actually observed at the site (O) to the number expected based on the predictions (E). O/E is a direct measure of how “complete” the native macroinvertebrate community is at that site, relative to what would be found at a similar unimpaired reference site. This method has several advantages. It is conceptually straightforward, in that an O/E value of 0.5 at an assessed site indicates that 50% of the native taxa have been lost. Similarly, an O/E value near 1.0 indicates that the native invertebrate community is largely intact. It is also site-specific, in that rather than making general predictions, for example at a regional level, about the native taxa to be expected, it makes specific predictions for each site based on that site’s environmental setting. And it is standardized, in that an O/E index value of 0.5 means the same thing everywhere, even among streams that may vary widely in the number of expected taxa. This allows for comparisons of conditions among sites that differ ecologically.
In the second common approach, what’s generally known as an Index of Biological Integrity (IBI), or in more recent years a Multi-Metric Index (MMI) is developed (Simon and Lyon 1995, Royer et al. 2001, Stoddard et al. 2008, Vander Laan and Hawkins 2014). Essentially, an IBI is constructed by examining various metrics that describe ecologically important aspects of the macroinvertebrate assemblage, and that are predicted to change in response to ecosystem degradation, for example overall taxa richness, the percentage of sensitive mayfly, stonefly and caddisfly taxa, the percentage of predatory taxa, and the percentage of pollution-tolerant taxa. These metrics are screened for their ability to discriminate between reference sites and a set of selected disturbed sites, based on the metric values calculated for each site. In this method, then, we are applying our knowledge of stream ecology and how we expect stream communities to respond to disturbance, to select the metrics. The metrics whose values best distinguish between reference sites and disturbed sites are then rescaled and combined into a single IBI, which can be used to assess ecological condition. In general, reference sites will have high IBI scores, whereas sites where ecological integrity has been impacted by disturbance will have low scores. The IBI approach is currently the most widely applied bioassessment methodology around the world.
Once developed, either index can be used to quantitatively assess ecological conditions at one or more sites that have been potentially impacted by human disturbance. Generally, assessed sites with index scores (either O/E or IBI) statistically close to the range of index scores at reference sites are judged to be equivalent to reference and hence not impaired by disturbance (or, in other words, to have high ecological integrity), while sites with index scores statistically different from reference sites are deemed to be impaired; that is, to have lower ecological integrity. Typically, the range of index scores is divided into classes that reflect the degree to which ecological integrity has been impaired, ranging from no impairment to severe impairment. The U.S. Environmental Protection Agency (EPA) uses both of these methods in its national assessments of the ecological condition of streams (Paulsen et al. 2008), although Alaska has not yet been included in those assessments.
The National Park Service is currently collaborating with the Bureau of Land Management (BLM) and Utah State University to apply these index-based bioassessment methods to streams throughout eastern Alaska. Although there has been limited development of both IBI (Rinella et al. 2005, Rinella and Bogan 2007, Rinella and Bogan 2010) and O/E (Simmons and Ostermiller 2012) indices in Alaska in the past, they have been relatively limited in geographic scope and examined relatively small numbers of sites. Accordingly, our project will be the most comprehensive and large-scale bioassessment effort to date in Alaska. As noted above, O/E indices and IBIs use different approaches to determining ecological condition, and can perform differently depending on various factors; accordingly we are developing and applying both methods, which will provide two complementary assessments and thus increase the robustness of our conclusions about the ecological condition of assessed streams.
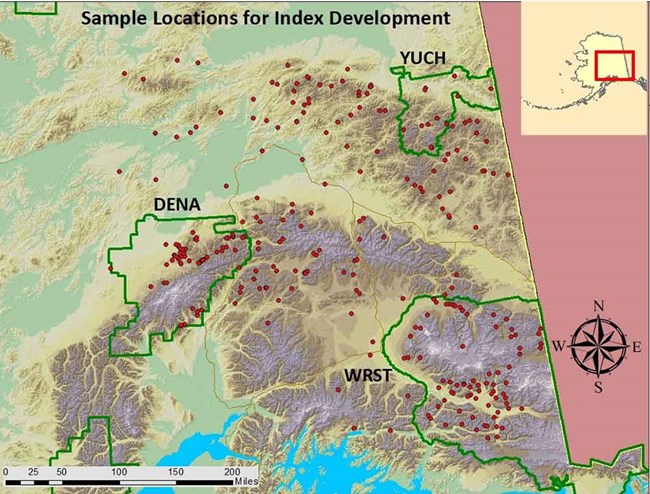
We are using macroinvertebrate and environmental data collected from 299 streams across a large swath of eastern Alaska (Figure 4) to develop the two indices. These data were collected by the Central Alaska Network for the NPS Inventory and Monitoring Program, by the Alaska Department of Environmental Conservation for an EPA-funded pilot study that was part of the EPA’s National Rivers and Streams Assessment, by the BLM Assessment, Inventory and Monitoring Program for the BLM National Aquatic Monitoring Framework, and by the U.S. Geological Survey as part of two NPS-funded water quality studies. In addition, data were collected in 2018 at a small number of sites in the Kantishna Hills of Denali National Park and Preserve in collaboration with the BLM to provide additional data on historically mined streams in that area. Data collection methods were similar across all agencies and are similar to the field protocols for the EPA National Rivers and Streams Assessment (USEPA 2017). The majority of these streams are classified as reference sites, meaning they are largely unaffected by any human activity, while a subset have been classified as potentially disturbed sites due to varying degrees of human impacts. Most of the disturbed streams have been affected by historic mining activity. Applying our indices to these disturbed sites will provide a quantitative assessment of the degree to which the disturbances have altered the ecological integrity of these streams. This in turn can help facilitate the prioritization of streams for restoration, by providing a defensible ranking system for comparing disturbed sites across interior Alaska using a common standard. Furthermore, these indices will be useful in determining the degree to which stream restoration efforts succeed in improving ecological integrity. Although this project is being developed with the primary goal of examining the effects of historical and current mining disturbance on streams, these indices will also allow agency managers and the state of Alaska to quantify the effects of other disturbances, for example roads and urbanization, on the integrity of stream ecosystems in Alaska.
References
Bailey, R. C., R. H. Norris, and T. B. Reynoldson. 2004.
Bioassessment of Freshwater Ecosystems using the Reference Condition Approach. Kluwer Academic Publishers, New York.
Bernhardt, E. S. and M. A. Palmer. 2011.
The environmental costs of mountaintop mining valley fill operations for aquatic ecosystems of the Central Appalachians. Annals of the New York Academy of Science 1223: 36-57.
Brim Box, J. and J. Mossa. 1999.
Sediment, land use, and freshwater mussels: Prospects and problems. Journal of the North American Benthological Society 18: 99-117.
Carter, J. L. and V. H. Resh. 2013.
Analytical approaches used in stream benthic macroinvertebrate biomonitoring programs of State agencies in the United States. U.S. Geological Survey Open-File Report 2013-1129. U. S. Geological Survey, Washington, D.C.
Clarke, R. T., J. F. Wright, and M. T. Furse. 2003.
RIVPACS models for predicting the expected macroinvertebrate fauna and assessing the ecological quality of rivers. Ecological Modeling 160: 219-233.
Daniel, W. M., D. M. Infante, R. M. Hughes, Y-P. Tsang, P. C. Esselman, D. Wieferich, K. Herreman, A. R. Cooper, L. Wang, and W. M. Taylor. 2004.
Characterizing coal and mineral mines as a regional source of stress to stream fish assemblages. Ecological Indicators 50: 50-61.
Hawkins, C. P., J. R. Olson, and R. A. Hill. 2010.
The reference condition: Predicting baselines for ecological and water-quality assessments. Journal of the North American Benthological Society 29: 312-358.
Herlihy, A. T., S. G. Paulsen, J. Van Sickle, J. L. Stoddard, C. P. Hawkins, and L. L. Yuan. 2008.
Striving for consistency in a national assessment: The challenges of applying a reference condition approach at a continental scale. Journal of the North American Benthological Society 27: 860-877.
Kolkwitz, R. and M. Marsson. 1908.
Okologie der pflanzlichen saprobien. Berichte der Deutschen botanischen Gesellschaft 26a: 505-519. (Translated 1967). Ecology of plant saprobia, pp. 47-52 in Kemp, L. E., W. M. Ingram, and K. M. Mackenthum, editors. Biology of water pollution. Federal Water Pollution Control Administration, Washington, DC.
Maret, T. R., D. J. Cain, D. E. MacCoy, and T. M. Short. 2003.
Response of benthic invertebrate assemblages to metal exposure and bioaccumulation associated with hard-rock mining in northwestern streams, USA. Journal of the North American Benthological Society 22: 598–620.
Metcalfe, J. L. 1989.
Biological water quality assessment of running waters based on macroinvertebrate communities: History and present status in Europe. Environmental Pollution 60(1-2): 101-139.
National Park Service (NPS). 2006.
National Park Service Management Policies. U.S. Government Printing Office. ISBN 0-16-076874-8.
Niyogi, D. K., D. M. McKnight, and W. M. Lewis, Jr. 2002.
Effects of mine drainage on breakdown of aspen litter in mountain streams. Water, Air and Soil Pollution: Focus 2: 329-341.
Paulsen, S. G., A. Mayio, D. V. Peck, J. L. Stoddard, E. Tarquinio, S. M. Holdsworth, J. Van Sickle, L. L. Yuan, C. P. Hawkins, A. T. Herlihy, P. R. Kaufman, M. T. Barbour, D. P. Larsen, and A. R. Olsen. 2008.
Condition of stream ecosystems in the US: An overview of the first national assessment. Freshwater Science 27: 812-821.
Rinella, D. J., D. L. Bogan, K. Kishaba, and B. Jessup. 2005.
Development of a Macroinvertebrate Biological Assessment Index for Alexander Archipelago Streams – Final Report. Environment and Natural Resources Institute, University of Alaska Anchorage.
Rinella, D. J. and D. L. Bogan. 2007.
Development of Macroinvertebrate and Diatom Biological Assessment Indices for Cook Inlet Basin Streams – Final Report. Environment and Natural Resources Institute, University of Alaska Anchorage.
Rinella, D. J. and D. L. Bogan. 2010.
Testing Alaska’s macroinvertebrate- and diatom-based stream condition indices in select urbanized streams. Environment and Natural Resources Institute and Alaska Natural Heritage Program, University of Alaska Anchorage.
Royer, T. V., C. T. Robinson, and G. W. Minshall 2001.
Development of macroinvertebrate-based index for bioassessment of Idaho rivers. Environmental Management 27: 627-636.
Simmons, T. and J. D. Ostermiller. 2012.
Monitoring water quality in Alaskan National Parks: Development of RIVPACS empirical models for assessing ecological condition and detecting change in a heterogeneous landscape. 8th National Monitoring Conference, Portland Oregon, April 30-May 4, 2012.
Simon, T. P. and J. Lyons. 1995.
Application of the index of biotic integrity to evaluate water resource integrity in freshwater ecosystems. Pages 245-262 in W. S. Davis, and T. P. Simon, editors. Biological Assessment and Criteria: Tools for Water Resource Planning and Decision Making. Lewis Publisher, Boca Raton, FL.
Stoddard, J. L., D. P. Larsen, C. P. Hawkins, R. K. Johnson, and R. H. Norris. 2006.
Setting expectations for the ecological condition of streams: The concept of the reference condition. Ecological Applications 16: 1267-1276.
Stoddard, J. L., A. T. Herlihy, D. V. Peck, R. M. Hughes, T. R. Whittier, and E. Tarquinio. 2008.
A process for creating multimetric indices for large-scale aquatic surveys. Freshwater Science 27: 878-891.
U.S. Environmental Protection Agency (USEPA). 1972.
The Challenge of the Environment: A Primer on EPA’s Statutory Authority. Environmental Protection Agency, Washington, DC. Available at: https://archive.epa.gov/epa/aboutepa/epa-history-water-challenge-environment-primer-epas-statutory-authority.html (accessed February 17, 2021)
U.S. Environmental Protection Agency (USEPA). 2017.
National Rivers and Streams Assessment 2018/19: Field Operations Manual – Wadeable. EPA-841-B-17-003a. U.S. Environmental Protection Agency, Office of Water Washington, DC.
Vander Laan, J. J. and C. P. Hawkins. 2014.
Enhancing the performance and interpretation of freshwater biological indices: an application in arid zone streams. Ecological Indicators 36: 470-482.
Van Nieuwenhuyse, E. E. and J. D. LaPerriere. 1986.
Effects of placer gold mining on primary production in subarctic streams of Alaska. Journal of the American Water Resources Association 22: 91-99.
Wagener, S. M. and J. D. LaPerriere. 1985.
Effects of placer mining on the invertebrate communities of interior Alaska streams. Freshwater Invertebrate Biology 4(4): 208-214.
Wedemeyer, K. 1987.
Effects of Antimony Mining on Stream Invertebrates and Primary Producers in Denali National Park, Alaska. Master’s thesis, University of Alaska, Fairbanks.
Wright, J. F., D. W. Sutcliffe, and M. T. Furse, editors. 2000.
Assessing the Biological Quality of Fresh Waters: RIVPACS and other Techniques. FBA, The Ferry House, Far Sawrey, Cumbria, England.
Last updated: July 6, 2021