The lower Cantwell Formation is a late Cretaceous fluvial deposit that contains dinosaur footprints and plant fossils.
Introduction
The Cantwell Formation crops out in the foothills and northern mountains of the Alaska Range in southcentral Alaska. In Denali National Park and Preserve (Denali Park) the formation can be readily recognized from the park road by its precipitous cliffs and dramatic bluish black, yellow and reddish colors.
Exposures in the Sable Moun-tain area in Denali Park consist of numerous successions of conglomerates, tabular and lenticular sandstones, organic-rich siltstones and shales. Plant fossils and recently discovered dinosaur footprints, bird footprints, and diverse invertebrate traces indicate a flourishing terrestrial ecosys-tem in a high-latitude environment (Fiorillo et al. 2007), as the Cantwell basin was probably located at a paleolatitude > 65 ºN at the time of deposition (Spicer et al. 1987).
Analyzed in lithological context, the plant fossils of the lower Cantwell Formation provide new information about the landscape, vegetation, climate and depositional processes for this Late Cretaceous basin.
Geologic Background
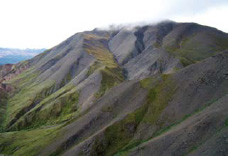
C.S. Tomsich
Beginning about 90 million years ago, Alaska grew in size as small segments of northward-displaced lithospheric crust (terranes) collided with the former south-ern Alaska plate margin (Plafker et al. 1994). The terres-trial Cantwell Formation was deposited during the final accretionary phase of the composite Wrangellia terrane to the North American continent (Ridgway et al. 1997). Now located within the Denali Fault system, the Cantwell Formation forms a 22 mile (35 km) wide, about 78 mi (125 km) long, east-west trending belt along the north-ernmost edge of the Alaska Range.
The formation comprises a 2.5 mi (4 km) thick fluvial sedimentary succession of late Campanian to Maastrichtian age (75 to 70 million years in age) (Ridgway et al. 1997) and an upper vol-canic unit of Paleocene and Eocene age (60 to 55.5 million years in age) (Cole et al. 1999). Both the upper and lower Cantwell Formation were folded and faulted during subsequent tectonic deformation and then uplifted during the rise of the Alaska Range. At Sable Mountain, the older sedimentary rocks are well exposed (Figure 1).
Fluvial Lithofacies and Facies Associations
A floodplain constitutes a low-gradient land surface that is drained by a major river and its tributaries. It is not a static environment. Here streams erode, transport and redistribute sediments as they change their course. In between streams and rivers, marshes, lakes and ponds develop in low-lying areas where drainage conditions are poor. Fine sediment accumulates slowly during ephemeral floods as the basin subsides. Levees form where coarser sediment builds up during overbank floods or where channels incise into older sediments.
As a result, differ-ent depositional environments such as channels, levees, crevasse splays, lakes, ponds and floodplain develop next to each other and succeed one another; the changing dy-namics produce a variety of lithologies (facies) and facies assemblages in an alluvial setting. Habitat preference, fre-quency of recurring floods and soil quality determine the types of vegetation that will grow in these different fluvial environments. Sedimentary and fossil records thus harbor complementary paleoenvironmental information.
Sedimentary Facies of the lower Cantwell Formation
Facies 1: Massive conglomerate
This facies contains clasts up to 6 inches (15 cm) in diameter with a predominantly matrix-supported texture. Deposits are often trough-shaped, winnow out laterally and are rarely more than 13 ft (4 m) thick. Moderately sorted clasts are subangular to wellrounded and occur in coarse to very coarse, subangular to subrounded sand. Typically conglomerates contain lenses of sand or thin layers of mud, dividing the deposits into multiple stories. Bedding is cross-stratified or massive. Tree trunk im-pressions are common. Facies 1 is interpreted as gravelly channel fill.
Facies 2: Pebbly sandstone
Facies 2 consists of tabular or lens-shaped coarse- to medium-grained, pebbly sandstones less than 20 ft (6 m) thick that grade laterally into finer-grained, organic-rich facies. Angular to well-rounded pebbles ranging in size from 0.1 to 2 in (0.3 to 5 cm) commonly rest on low-angle foresets. Deposits are massive or trough cross-stratified and grade upward into medium sandstone. Facies 2 is in-terpreted as levee, small channel or crevasse splay channel fill.
Facies 3: Coarse- to medium-sandstone
Tabular and lenticular coarse- to medium-grained sandstone bodies embedded in finer-grained deposits are typically less than 3.3 ft (1 m) thick. Deposits are massive, planar, or trough cross-bedded and may have granules on foresets. Sand grains are predominantly subangular and moderately sorted. Facies 3 is interpreted as levee, small shallow channel fill and overbank sheetflood deposits.
Facies 4: Fine sandstone
Facies 4 is a tabular or lenticular sandstone embed-ded in finer-grained deposits consisting of subangular to subrounded grains. Bedding is massive, rippled or trough cross-stratified with rare mud on foresets. This sandstone is typically burrowed and moderately rooted. It frequent-ly contains twigs and cones of Metasequoia, Equisetum rhizomes and stems, leaf impressions and wood. Facies 4 is interpreted as small channel fill, crevasse splay, and sheet-flood deposits.
Facies 5: Interbedded fine to very fine sandstone and silt-stone
This facies consists of laterally extensive decimeter- to centimeter-scale tabular beds of interbedded fine-to very fine-grained sandstone and siltstone that often grade into siltstone. Sandstone is massive or rippled. Deposits contain invertebrate trace fossils and abundant plant macrofossils. Roots and concretions indicate weak soil development. Facies 5 is interpreted as crevasse splay, sheetflood, levee and overbank deposits.
Facies 6: Interbedded fine to very fine sandstone and mudstone
Facies 6 is a centimeter to millimeter-thick fine- to very fine-grained, tabular sandstone interbedded or in-terlaminated with mudstone. It may be rippled, trough cross-bedded or laminated and contain small traces made by beetles, gastropods, ostracodes and annelids. Plant macrofossils are abundant; and roots and concretions are evidence for soil development. This facies is interpreted as distal crevasse splay, sheetflood, levee and overbank deposits.
Facies 7: Siltstone
Facies 7 is a dark grey to black siltstone, centimeters to tens of meters thick. It is organic-rich and may be interlam-inated with mudstone. This facies contains conifer needles, Equisetum rhizomes, carbonaceous roots and other plant fragments. Iron concretions or nodules are very common. Beds typically show irregular relief at the top that was likely caused by dinoturbation. This facies is interpreted as distal crevasse splay, floodplain and lake margin deposits.
Facies 8: Mudstones and shales
This very fine-grained facies consists of dark grey to black organic-rich mudstone and shale that is usually 0.4 in to 3.3 ft (1 cm to 1 m) thick, rich in clay, and locally calcareous. Mudcracks may be seen at the surface or beds may be irregular due to erosion and bioturbation. Facies 8 typically occurs in coarser-grained deposits in the form of rip-up clasts. Fossils consist of Equisetum segments, small, frequently coalified, plant fragments, carbonaceous root-lets, and bivalve and gastropod shells. This facies is inter-preted as floodplain, shallow lake or pond deposits.
Facies 9: Unorganized deposits
Facies 9 contains clasts of all sizes, pieces of wood, and mud rip-up clasts in a sandstone or organic-rich mudstone matrix. Deposits are often poorly consolidated, infrequent and occasionally contain in situ tree stump impressions. We interpret Facies 9 as debris flows.
Alluvial Deposition
The facies and facies associations in the lower Cantwell Formation indicate that the rocks at Sable Mountain represent alluvial sheetfloods, braided chan-nels, crevasse channels and splays, overbank and levee deposits, small shallow lakes or ponds and floodplain sediments. Lithologies reflect sedimentary processes typical of low-lying fluvial environments. The sedimentary environment has been characterized as an alluvial fan system drained transversally by an axial sandy braided river (Ridgway et al. 1997).
The many tabular sand-stones and floodplain fines at Sable Mountain indicate that deposition occurred close to the center of a fluvial valley either at the distal end of an alluvial fan lobe where ephemeral floods would have been common (Leeder 1999) or in the floodplain of a trunk river and its tributaries. Poorly organized colluvial deposits (Facies 9) in the Sable Mountain area and elsewhere in the basin (Ridgway at el. 1997) suggest that the tabular sheetflood sandstones may be periodic, distal allu-vial fan stream flow deposits.
However, trough-shaped conglomerates and coarse sandstones in extensive floodplain fines signify that alluvial fan sediments in-terfinger with the floodplain deposits of the main river system that drained the ancient valley.
The different lithologies and geometries of the sedi-ment created a variety of local habitats with different drainage conditions. For example, the numerous suc-cessions of shallow, fine-grained sandstones and silt-stones suggest that overbank floods were frequent but that deposition was quickly abandoned as waters receded.
Abundant roots in the interbedded sandstones suggest that these facies allowed for a rapid re-vegeta-tion of the disturbed areas. The lithology of the deposits becomes finer-grained with increasing distance from active streams. Marshy lowlands and standing water bodies developed in poorly drained areas. Minor paleosol development in floodplain fines signals predominantly wet conditions.
As fluctuating water tables generally affect soil formation and consequently plant species distribution, a riparian vegetation would have been required to readjust with each change to the depositional setting (Dwire et al. 2006).
The Fossil Flora of the Lower Cantwell Formation
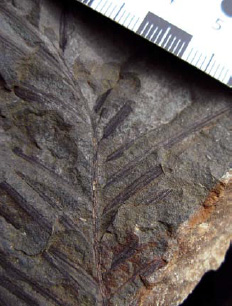
C.S. Tomsich
The lower Cantwell Formation yields abundant plant impressions including the stems and rhizomes of horse-tails (Equisetum); fronds of schizaceous and gleichena-ceous ferns and of Cladophlebis, an extinct fern; leaves, cones and seeds of the deciduous conifers Metasequoia (Figure 2) and Glyptostrobus; fragments of ginkgophytes, cycadophytes, diverse dicotyledonous angiosperm leaves (Figures 3-4), and the monocot Sparganium (Thypha) and other unidentified blade-like leaves. Also present are log impressions (Figure 2B) and lithified wood.
Sparse and poorly preserved fossil pollen grains and spores extracted from organic-rich mudstones yielded the angiosperm pollen taxa Alnipollenites and Aquillapollenites, the coniferous pollen taxa Taxodiaceaepollenites, few cycad or ginkgo pollen grains, and abundant horsetail and fern spores.
Angiosperm leaf fossils have simple forms with palmately, pinnately or palmately-pinnately venation, but fragments of large palmately-lobed leaves also occur. Leaf sizes range from 0.6 in (1.5cm) to an estimated 20 in (50 cm), based on vein spacing.
The majority of the taxa belong to the families Platanaceae, Nymphaeaceae, Menispermaceae and Hamamelidaceae. Of the platanoid types forms such as Pseudoprotophyllum are rare while leaves of betulaceous morphology (cf. Corylites and Alnites) are especially abundant. Other taxa such as Viburniphyll and the trochodendroid, zizyphoid and the vine-like menispermoid groups often occur in association with the platanoids and remains of taxodiaceous conifers. In all, 21 angiospermous morphotypes were identified from the study area on the basis of leaf organization.
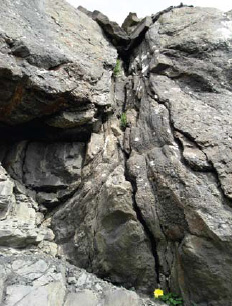
C.S. Tomsich
The Depositional Habitat Environments
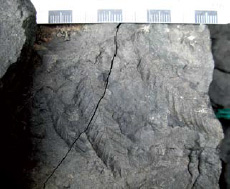
C.S. Tomsich
Plant fossils recovered from fluvatile facies approximate the composition of an ancient local flora (Spicer and Par-rish 1990). At Sable Mountain the sandier deposits apparently favored leaf preservation due to better drainage and rapid accumulation. Fossil assemblages are more diverse in levee, crevasse splay, and overbank deposits than in flood-plain and lake facies, and plant community associations are interpreted to correspond to three sub-environments: stream margin, elevated floodplain and low-lying flood-plain.
Angiosperm leaf taxa appear individually or as part of a mixed forest litter. Platanoid trees or shrubs grew pre-dominantly along streams (Facies 2 and 3). A more complex assemblage containing Metasequoia leaves and cones and diverse hamamelid angiosperm leaves are found primar-ily in sandy overbank deposits and embankments (Facies 3-6). The finer-grained and more organic-rich floodplain facies (Facies 5-7) contain elements of the conifer Glypto-strobus, the angiospermous leaves Alnites, Menispermites, and Sparganium, rare cycad leaves, Equisetum and a variety of ferns.
The different fossil assemblages signify that soil and drainage conditions varied within the floodplain according to facies distribution and frequency of river sand and fertile mud deposition. In better-drained and more built-up areas diverse angiosperm shrubs and small-diameter trees may have formed an open canopy forest together with Metasequoia. In contrast the dominant lowland vegetation in the floodplain and along the lake and pond margins consisted of a low-diversity flora, which included platanoids, nymphaelalean vine-like plants, a variety of ferns and hydrophilic gymnosperms. Cycads, Equisetum and herbaceous plants covered the marshes.
Geographic Species Distributions and Paleoclimate
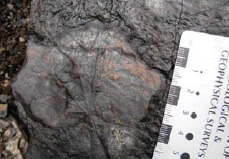
C.S. Tomsich
The Cantwell Flora contains elements that are present in other Arctic floras. Taxodiaceous conifers such as Metasequoia and Glytostrobus and species of the dicot leaf genera Trochodendroides, Menispermites, Zizyphoides, Pseudo-protophyllum, Corylites, the monocot Sparganium and the ferns Gleichenia and Cladophlebis were widespread in the Arctic during the late Cretaceous (Hollick 1930, Spicer et al.
1987, Budantsev 1992, Herman and Spicer 1995). Yet, even though as these genera are well represented in the Cantwell flora, it is still unclear how they relate at the species level given that very few species could be identified.
Fossil leaf character correlation with modern leaf physiognomies and climate variables has proven effective in computing ancient climate parameters (Wolfe and Spicer 1999). Using Climate Leaf Analysis Multivariate Program (CLAMP) (Wolfe and Spicer 1999), our first esti-mate for a mean annual temperature (MAT) is 51.1ºF (10.6 ºC), a warmest monthly mean (WMMT) is 69.1ºF (20.6 ºC) and a coldest monthly mean (CMMT) is 34.7ºF (1.5 ºC). In comparison, Spicer and Parrish (1990) calculated a MAT of 35.6 to 43ºF (2 to 6 ºC); and Parrish et al. (1987) es-timated the WMMT at 50 to 53.6ºF (10 to 12 ºC) and the CMMT at 35.6 to 39.2ºF (2 to 4 ºC) for the Maastrichtian flora of the Prince Creek Formation which crops out on Alaska’s North Slope.
These results indicate that the Earth was much warmer at the time and that winters were mild. Variables computed for the Cantwell basin, however, show greater disparity between summer and winter temperatures. While the CMMT calculated for the two floras is very similar, average summer temperatures are significantly higher for the Cantwell data. This disparity could be a symptom of terrain and latitudinal differences. The North Slope at the time was a fluviodeltaic setting facing the Arctic Ocean. It was located at approximately 85 ºN, and angiosperm diversity was report-edly low (Spicer and Parrish 1990).
The Cantwell basin, in contrast, was located further inland, 10 degrees or more to the south, opening toward an epicontinental sea to the east, the Cretaceous Western Interior Seaway (Ridgway et al. 1997). Results suggest a strong pole-ward summer temperature gradient possibly due to southward increases in insolation. Taxa and calculated temperatures indicate that the Cantwell flora like other late Cretaceous Arctic floras can be categorized as a polar coniferous and broad-leaved deciduous forest flora (see Wolfe 1987).
Conclusion
The late Cretaceous lower Cantwell Formation at Sable Mountain represents braided channel, small channel, crevasse splay, levee, overbank, floodplain, and lacustrine sediments and occasional debris flows typical for floodplain and/or distal alluvial fan deposition.
Plant fossils analyzed in the context of lithofacies show that coniferous and angiospermous plants formed distinct floral community associations in a variety of depo-sitional environments. Vertical facies successions and accompanying changes to the floral communities reflect altered hydraulic conditions during floodplain evolution. Taxodiaceous conifers and dicotyledonous angiosperms achieved significant diversity; taxa at the family and genus levels correspond to those described from other Arctic floras.
Accordingly, the Cantwell flora is classified as a polar broad-leaved deciduous mixed forest flora. CLAMP analysis implies that climate in this high-latitude region was temperate, with mild winters and consider-ably warmer summers than those predicted for Alaska’s North Slope during the Late Cretaceous. In this way, the paleofloristic data amend our understanding of vegetation distributions and past climate variability in ancient greater Beringia.
Acknowledgments
We gratefully acknowledge the National Park Service, the Denali Educational Center, the Murie Science and Learning Center, UA Museum of the North, the Geological Society of America, the Alaska Geological Society, and British Petroleum for sponsoring this project, and Phil Brease, Denali NPS geologist, and Dr. Lucy Tyrrell, Denali NPS Research Administrator, for logistical support. We also wish to extend our thanks to Dr. Dave Sunderlin for helpful comments and assistance.
Part of a series of articles titled Alaska Park Science: Volume 8, Issue 2: Park Science in the Arctic.
Last updated: February 5, 2015