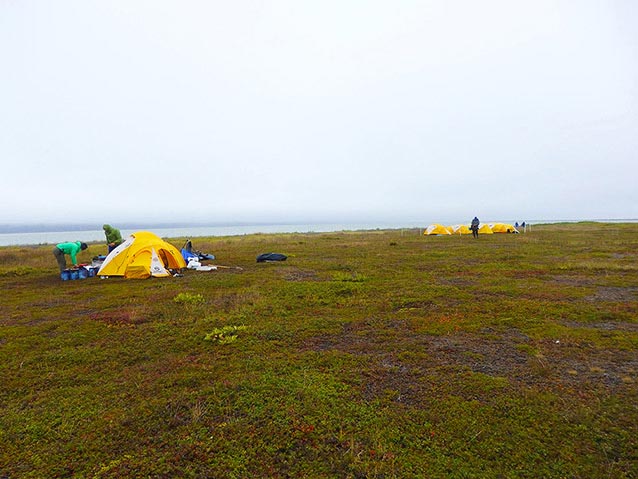
Photo courtesy of Brooke Luokkala
The National Park Service (NPS) stewards roughly 1,000 miles (1,600 kilometers) of coastal landforms in Northwest Alaska (Figure 1). Bering Land Bridge National Preserve and Cape Krusenstern National Monument exhibit a wide variety of coastal landforms including barrier lagoons, tundra bluffs, accreting spits, and beach ridge complexes; all home to vulnerable fauna, flora, and avian communities; internationally significant archaeological, historic, and ethnographic resources; and unique paleoecological and fossil records. Coastal landform erosion and its impact on cultural resources in Northwest Alaska has been a focal study area since the 1980s (Jordan 1988; Mason 1995; Jordan and Mason 1999; Figure 2). Recent geomorphological studies, however, show that landscape erosion have intensified since 1980s, erasing the evidence of human adaptation to the coast at unprecedented rates (ACIA 2004; Manley 2007). To address this significant issue an interdisciplinary team of researchers comprised of archaeologists and geologists led by NPS and its research partners are updating perceptions about how these dynamic earth processes are impacting vulnerable cultural and paleontological resources on the coast (Figure 3).
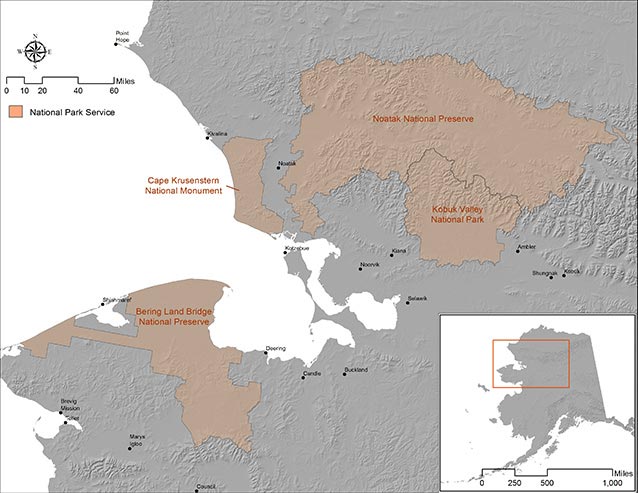
Ice Age History
Archetypal Ice Age fossils such as the woolly mammoth (Mammuthus primigenius), steppe bison (Bison priscus), and wild horse (Equus sp.; Hopkins 1982; Guthrie 2003, 2006; Höfle 2000; Hardes 2014) roamed Beringia until the end of the Ice Age when climatic amelioration ended a 75,000-year glacial period. This general warming trend (termed “Holocene”) began roughly 12,000 years ago, sparking dramatic shifts in ancient Beringian biomes (Bigelow 2003; Bird 2009; Calkin 1998; Jacoby 1999; Mann 2002), as melting ice sheets and glaciers steadily raised global sea levels to current levels by the mid-Holocene (7,000 to 5,000 years ago) inundating the Bering Land Bridge (Manley 2002; NOAA 2008). Fossils and sediments preserving a record of ancient flora and fauna during the terminal end of our last great Ice Age are being destroyed by powerful Earth processes along coastal peat bluffs.
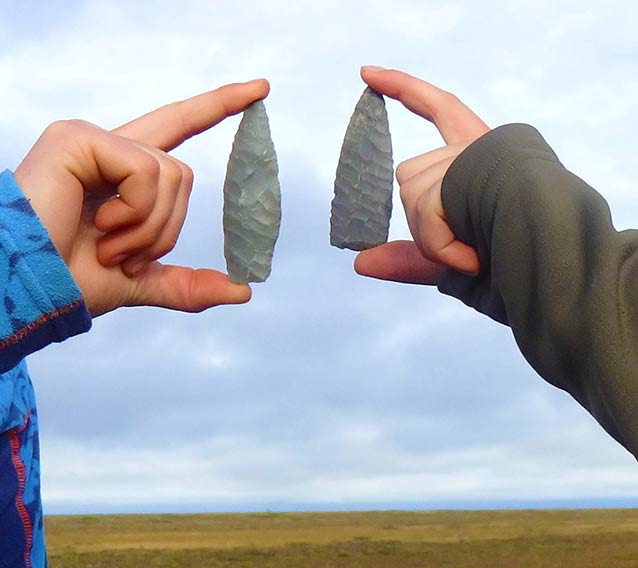
Photo courtesy of Brooke Luokkala
Cultural History and Climate Change
Cultural resources (i.e., archaeological, historic and ethnographic evidence) are the physical expression of past and present human land uses; a testament of how people adapt to their dynamic surroundings. As global temperatures and sea levels fluctuated throughout the Holocene, altering coastal landforms and biota (Bigelow 2003; Bird 2009; Calkin 1998; Jacoby 1999; Mann 2002), humans adapted unique lifeways in order to take full advantage of the world around them. This fundamental interaction between humans and their surroundings is called human-behavioral ecology, and is the theoretical perspective archaeologists use to study the enigmatic behaviors of our prehistoric forbearers (Cronk 1991).
Archaeological evidence can be used to demonstrate humankind’s connection with the region’s coast for almost 12 millennia starting with Paleoarctic (11,000 to 6,000 years ago) and Northern Archaic (6,000 to 3,000 years ago) foraging groups (Giddings and Anderson 1986; Anderson 1988; Schaaf 1988). These earlier groups may have taken advantage of opportunities along the coast, or simply passed through. It was, however, not until the late Holocene (a period spanning the last 5,000 years) that humans would develop lifeways and cultural identities centered on marine resources in the region (Giddings and Anderson 1986; Figure 4).
Tradition | Cultural Sequence | Approximate Age Range (Max) | Approximate Age Range (Min) |
---|---|---|---|
n/a | Paleoarctic | 11000 | 6000 |
n/a | Northern Archaic | 6000 | 3000 |
Arctic Small Tool | Denbigh | 4500 | 2750 |
Arctic Small Tool | Choris | 2750 | 2250 |
Arctic Small Tool | Norton | 2250 | 1350 |
Arctic Small Tool | Ipiutak | 1750 | 1150 |
Northern Maritime | Birnirk | 1350 | 750 |
Northern Maritime | Western Thule | 1000 | 550 |
Northern Maritime | Arctic Woodland | 750 | 250 |
Northern Maritime | Kotzebue Period | 550 | 250 |
Ethnohistoric | Historic & Contemporary Inupiat Lifeways | 165 | 0 |
Figure 4: Coastal cultural sequence model.
Neoglaciation marks the start of the late Holocene, coinciding with the formation of the beach ridges and barrier island landforms in the region. This dramatic shift from warming, ca. 9,000 to 5,000 years ago to cooler global temperatures, and subsequent changes in biomes and resources likely compelled humans to focus more intensively on coastal environments and resources. These coastal landforms provided ideal conditions for human foraging groups focused on marine resources to support larger populations and influenced sociocultural complexity (Giddings and Anderson 1986; Schaaf 1988; Jordan and Mason 1999; Freeburg and Anderson 2012). Barrier islands and beach ridges are incredibly vulnerable to the ravages of climate change in the region, and are being erased at unprecedented rates (ACIA 2004; Manley 2007)—destroying an important record of human land use throughout the late Holocene.
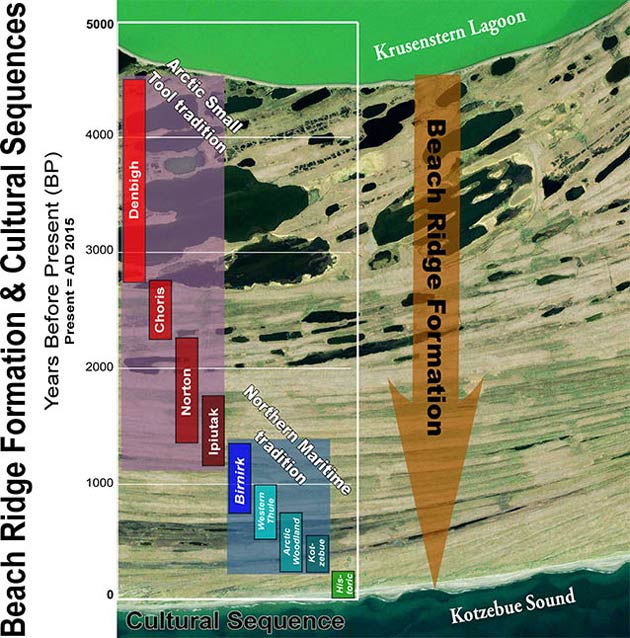
There is no better example of the interaction between human foraging groups and the environment than at the Cape Krusenstern and Cape Espenberg (Bering Land Bridge) beach ridge complexes, where horizontal beach ridge building occurred in synchronicity with human occupation (Gidding and Anderson 1986; Darwent 2013). Louis Giddings, pioneer archaeologist, developed a coarse grained model of cultural change based on the sequential formation of beach ridges, using horizontal stratigraphy as a way to reconstruct regional culture histories or “beach-ridge archaeology” (Giddings 1967; Giddings and Anderson 1986).
Perhaps the earliest evidence of a coast-adapted lifeway derive from minute traces of small seasonal camps and artifact scatters deposited 4,500 to 2,750 years ago (Denbigh Flint complex); this evidence found within paleosols (ancient soil) along the oldest beach ridges, marked the beginning of the Arctic Small Tool tradition (ASTt; Giddings and Anderson 1986; Tremayne 2014). The presence of semi-subterranean sod houses as well as pottery and complex tool assemblages are indicative of more sedentary lifeways focused on the coast during the Choris cultural period (2,750 to 2,250 BP) and subsequent Norton cultural period (2,250 to 1,350 BP; Giddings and Anderson 1986). Human occupation of the coast during the ASTt reached its zenith during the Ipiutak culture (1,750 to 1,150 BP), marked by evidence of increased settlement, technological innovation, and growing sociopolitical complexity (Larsen and Rainey 1948; Anderson 1962; Giddings and Anderson 1986; Schaaf 1988; Bowers 2006; Figure 5).
There is overlap between ASTt and Northern Maritime tradition (NMt) between 1,350 and 1,150 years ago (Giddings and Anderson 1986; Schaaf 1988), sparking a cultural transition which was heavily influenced by the Medieval Warm Period in Alaska (1,200 to 800 years ago; Hu 2001). Iñupiat people living in Northwest Alaska today are directly descended from the NMt, which dominates the archaeological record on the region’s coast. It was during NMt people permanently occupied and adapted to the coast, experienced a florescence in population, increased sociopolitical complexity, managed extensive trade networks and expansive territories, and developed large settlements and intricate tool assemblages. Material deposits and features derived from the Thule (950 to 550 years ago), Kotzebue cultures (550 to 250 years ago), and contemporary Iñupiat people within the last 1,000 years represent the most intensified human use of the coast (Giddings and Anderson 1986; Schaaf 1988; Anderson 2013). Arctic Woodland culture likely represents an expansion of Thule and Kotzebue cultures into interior watersheds between 750 to 250 years ago (Giddings 1952; Giddings and Anderson 1986). Climate amelioration sparked a 300-year global cooling trend called the Little Ice Age beginning 500 years ago, which once again influenced a regional shift in settlement and subsistence patterns as human foraging groups moved away from sea mammal-based lifeways to those centered around fishing, as evinced by advanced fishing tools and modified land use patterns (Giddings and Anderson 1986).
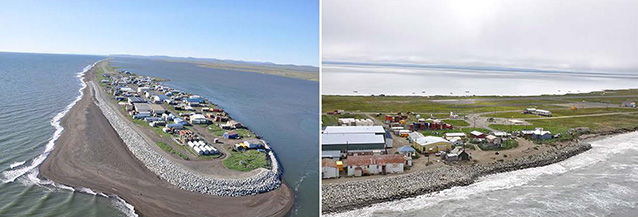
Photo courtesy of NPS Shorezone Mapping Project
The historic period in the region began circa 1950s and ended 1965 (165 to 50 BP), representing a period of significant cultural and technological change for the region’s indigenous people. While the Iñupiat had very limited interface with outsiders prior to this time (often termed “contact era”), the region was eventually exposed to the Euro-American fur trade industry, exploration, and subsequent distribution of firearms and other technological advances. A well-documented caribou famine in the 1890s served as the impetus for introducing reindeer herding in Northwest Alaska. By the early Twentieth Century, church missions established the current village centers in the region, contributing to a more sedentary lifeway (Ray 1975; Burch 1998, 2006).
Contemporary Iñupiat societies and their ancestors have relied on land and resources in the region since time immemorial, developing unique ways in which to thrive and adapt to arctic coastal environments (Ray 1975; Burch 1998, 2006). These distinctive lifeways are imperiled in places like the Native Villages of Kivalina (Figure 6) and Shishmaref (Figure 7) where the effects of climate change are exacting a direct and unprecedented impact on food security and cultural identity (Druckenmiller 2011; Willis 2004; Sackur 2013).
Processes of Coastal Change in the Arctic
Coastal erosion occurs through a number of processes including storm driven waves along the bluffs and low-lying barrier islands, tidal inlet migration, and sediment redistribution due to large overwash events. Storm events appear to be key drivers, greatly influencing rates of coastal erosion (Figure 8).
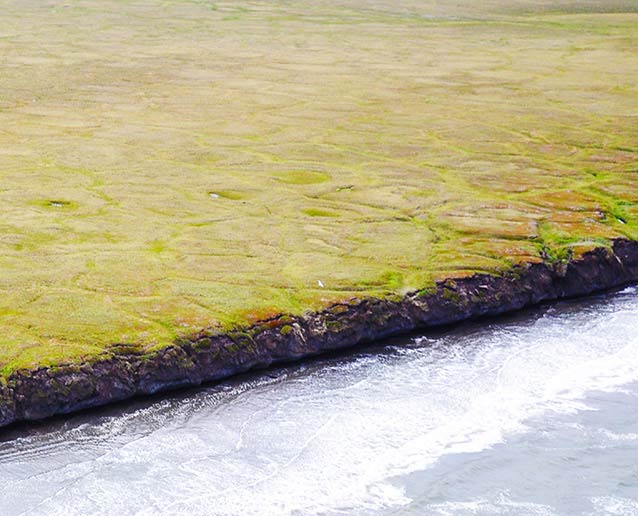
Photo courtesy of Gregory Luna Golya
Unlike coastal systems in the lower latitudes, Bering Land Bridge and Cape Krusenstern coastlines are closely tied to the dynamics of sea ice. Sea ice exerts crucial control over coastal processes, and climate change is now altering the sea ice regime in the Bering and Chukchi Seas quickly and radically. Arctic sea ice extent and thickness has been declining more than 10 percent per decade since satellite observations began in 1981 (Stroeve 2012). Over the last 40 years, the duration of landfast (or shorefast) sea ice along the Beaufort Sea coast has declined by approximately one week per decade (Mahoney 2014). A summer ice-free Arctic ocean is expected by 2030 (Overland and Wang 2013).
Another unique feature of the Bering Land Bridge and Cape Krusenstern shorelines is the presence of permafrost-rich bluffs, which when thawed are susceptible to erosion during storm events. Current observations show widespread permafrost degradation adjacent to the shoreline and the development of thermoerosion gullies draining onto the beach. In some cases, the process causes lagoons to breach (Figure 9).
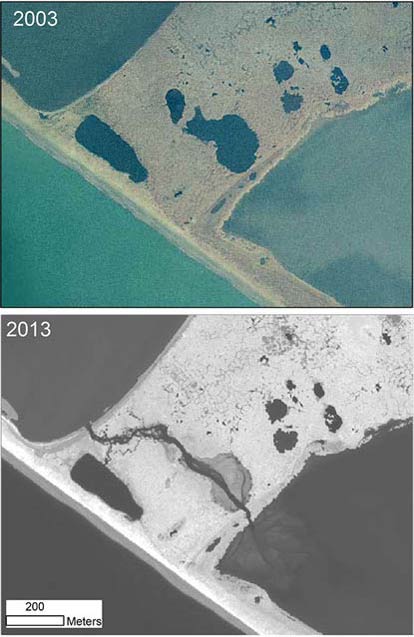
Coastal Permafrost Dynamics
Cape Krusenstern exhibits a number of typical thermokarst features such as ice-wedge polygons and shallow lakes situated among a series of ancient beach ridges. Permafrost thawing occurs as average temperatures in May rise above freezing, reaching its maximum active thawing depth by the end of summer. This process reverses in early fall as average temperatures drop below 0°C (32°F) and the ground once again freezes. Depth of the active thawing layer is driven by variability in seasonal climate and impacted by both unseasonably long summers and higher-than-average temperatures. The rate of active thawing influences stability of the underlying permafrost layer. Permafrost changes can therefore be expected with a warming regional climate, particularly with warmer-than-average summers and longer seasonal timeframes when the average temperature remains above freezing (Figure 10).
Permafrost contains substantial frozen organic material and thawing can lead to decomposition of this material and the associated release of greenhouse gasses, such as methane. For this reason, permafrost loss is considered a global environmental threat. Locally, permafrost changes threaten infrastructure, ecology, and cultural resources by altering the dynamics of the shallow subsurface. This can result in accelerated erosion, vertical transport of artifacts, and general distortion of features within the archaeological record, thereby destroying or complicating contextual relationships. Archaeological sites in low-lying coastal areas of Bering Land Bridge and Cape Krusenstern are further exposed to salt water inundation in the wake of permafrost failure (Figure 11).
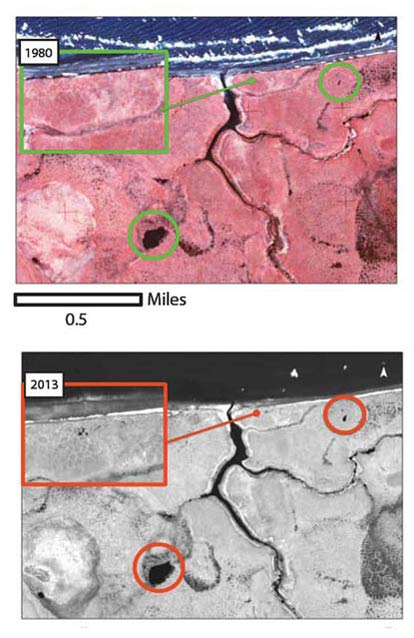
A 2011 geophysical investigation of the Old Whaling archaeological site (Cape Krusenstern) revealed a late-summer permafrost table deeper than had been noted in previous archaeological investigations in the 1950s and even as recently as the early 2000s (Wolff and Urban 2013). The thickness of the active layer in a 100-meter stretch between two excavations was determined with ground-penetrating radar (GPR) in 2011, and then re-collected at the same location in 2013 and 2015. With GPR, the frozen layer is distinguished as a subsurface interface of abruptly increasing velocity in comparison to the lower velocity active layer. The velocity change occurs because frozen ground has different electrical properties than thawed ground. This results in a reflection from the frozen layer. Velocity estimates of the active layer using hyperbolic curves generated by frost cracks in the subsurface are then used to convert the two-way travel time of the radar signal into a depth representing the permafrost table.
Geochronology of Coastal Change
Changes in coastal geomorphology occur at many different time scales. Glacial-interglacial cycles operate over tens of thousands of years and cause fluctuations in sea level influenced by the accumulation and release of water for ice sheet growth and decay. As a result of fluctuating sea level, the shoreline moves either further inland or offshore. The modern day shorelines of Bering Land Bridge and Cape Krusenstern have evolved over the last 10,000 (during the Holocene) years as sea level rose from its ice age low and has fluctuated around its current level (Carter and Woodroffe 1997). Permafrost bluff sediment and ice accumulated over tens of thousands of years due to the continuous deposit of silt by wind and snow processes during the late Pleistocene.
Impacts of Coastal Change
Over the last two years we have conducted a combination of field and remote sensing-based investigations looking into whether the coastlines of Bering Land Bridge and Cape Krusenstern have begun to change more rapidly due to loss of sea ice and warming air and ocean temperatures. Building on previous work by Manley (2010), measuring rates of change over two time periods (1950-1980, and 1980-2003), current data extends these observations to 2014. Our measurements show an increase in rates of coastal erosion along the Bering Land Bridge and Cape Krusenstern coastlines since 2003. Between 1980 and 2003, rates of coastal change along the Bering Land Bridge shoreline averaged .03 feet/year (0.01 meter/year) while Cape Krusenstern was found to be on average gaining land at 0.23 feet/year (0.07 meters/year) Bering Land Bridge’s coastline is now eroding at an average rate of 2.82 feet/year (0.86 meters/year) and Cape Krusenstern’s 2.26 feet/year (0.69 meters/year). This increase in erosion rates also coincides with a significant decrease in the duration and extent of sea ice in the Arctic.
Shrinking sea ice increases wave action, which provides more energy for coastal erosion (Overeem 2011) and barrier island inundation and sediment redistribution during storm events. The effects of changing sea ice condition are made even greater by the presence of extensive sections of ice-rich, permafrost bluffs. This makes the western Arctic coastline particularly vulnerable to the increasing temperatures of both the ocean and air as icy sediments thaw more rapidly.
Bluff erosion at Kitluk River ethnohistoric Iñupiat settlement (Bering Land Bridge) (Figure 12) has been the focus of past shoreline attrition studies (Jordan 1988). Fossils are commonly found exposed on the beach to the east of the settlement, eroding from bus-sized blocks of peat that had been preserved in Pleistocene ice and sediments. In 2015 a NPS survey team revisited the historic site to assess its continued vulnerability to coastal processes. Bounded by the Chukchi Sea (to the north) and storm surge channel along Kitluk River (to the west), the bluffs are continually battered by storms and the erosion impact is amplified by active thermokarsting. Landform erosion was measured using orthorectified historic aerial photogrammetric techniques (Manley 2010) to reconstruct bluff edges from 1950, 1980, and 2003 in addition to GPS data collected in 2015 by NPS archaeological survey crew. Eleven control points were arrayed along the 2015 bluff edge, spaced apart at 10-meter intervals to measure minimum, maximum, and average erosion from 1950 to 2015. Since 1950 there has been an average bluff loss of 53.5 feet (16.3 meters), ranging between 3.8 feet (1.1 meters) and 109.4 feet (33.3 meters). This is indicative of moderate-to-heavy annual erosion rates between 0.1 feet/year (0.02 meters/year) and 1.7 feet/year (0.51 meters/year). The components formerly contributing to the significance of Kitluk River site have been lost to the ravages of an unrelenting Chukchi Sea (Figure 12).
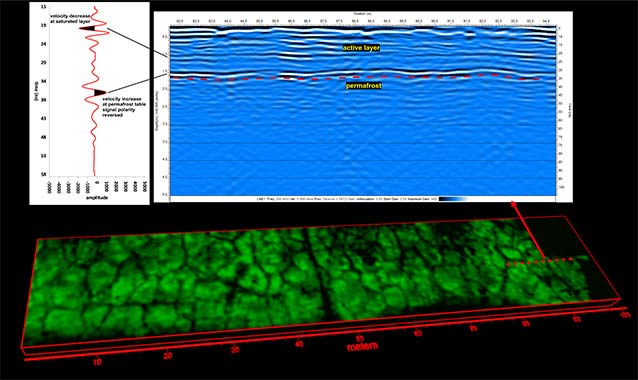
Perhaps the most vulnerable coastal landform in Northwest Alaska is the internationally significant Cape Krusenstern beach ridge complex. The storm battered fore-ridges have shown the most severe attrition since 1950 while the back-ridges are extremely susceptible to the impacts of melting permafrost in the form of frost-cracking and changing water tables, which amplify the effect of eolian erosion. As in the Kitluk River example, orthorectified historic aerial photogrammetric techniques (Manly 2010) aided in reconstructing fore-ridge edges in 1950, 1980, and 2003. Fore-ridge reconstruction for 2015 is a projection based on 2010 satellite imagery and average rates of erosion between 1950 and 2003. Thirteen control points were arrayed along the projected 2015 fore-ridge edge, spaced at 10-meter intervals to measure minimum, maximum, and average erosion between 1950 and 2015. Since 1950 there has been an average fore-ridge loss of 351.3 feet (107.1 meters), ranging between 314.7 feet (95.9 meters) and 379.2 feet (115.6 meters). This equates to severe annual erosion rates between 4.8 feet/year (1.5 meters/year) and 5.8 feet/year (1.8 meters/year). This pattern of erosion occurs throughout the Cape Krusenstern beach ridge complex, as cryogenic and eolian processes continue to impact the older ridges at unprecedented rates.
GIS Modeling
A Geographic Information Systems (GIS)-based predictive model was created to assist archaeologists in locating and documenting sites before they are lost to the effects of a changing climate. The predictive model is composed of two components: the first is a deductive model identifying parts of the coast subject to erosion, and the second is an inductive model identifying areas of the coast likely to contain an archaeological site. The two pieces were then added together to highlight the areas of the coast that are both likely to erode and to contain an archaeological site. The raster can then be used to prioritize areas for archaeological study.
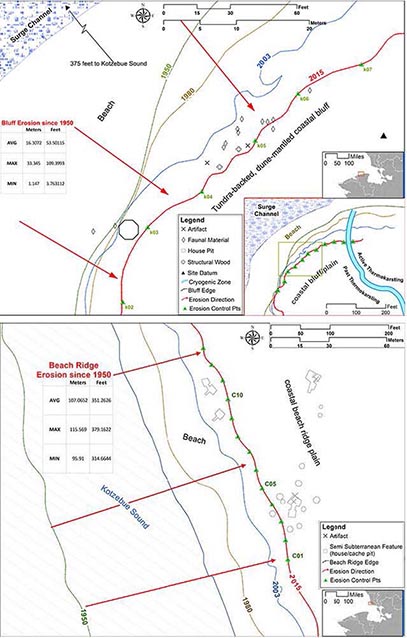
Progress Report
Since 2012, the NPS has administered a vulnerability assessment program to identify our most significant and at-risk cultural and paleontological resources on the western Arctic coast (Anderson 2015). A vulnerability prioritization matrix was developed in cooperation with Portland State University (Anderson 2015) and further refined by the NPS to aid in the prioritization process. The matrix serves as a tool providing resource managers and researchers with consistent measures to assess the vulnerabilities of our affected resources. It does so by quantifying the product of four values including significance/data potential, condition/integrity, impact severity, and immediacy of threat. Significant/data potential is the antecedent value factored to the sum of the other values and based on the resource’s potential to contribute to our understanding of past human adaptations and natural histories. Condition/integrity is determined by the integrity or quality of the significant components of a resource. Impact severity is used to determine how climate change or other impact agents have affected the significant components of a resource. Immediacy of threat is a chronological estimation of when an impact is expected to adversely affect the significant components of a resource.
The GIS model was tested with survey data collected by Portland State University in 2013 (Anderson 2015). The GIS predictive model has proven to be a useful tool for identifying our most significant and at-risk resources on the coast, further aiding in our efforts to understand and triage the impacts of climate change.
The NPS and our research partners have thus far succeeded in assessing vulnerabilities of cultural and paleontological resources along approximately 180 miles (289.7 kilometers) of coastline. The vulnerability matrix has been applied to 192 cultural and paleontological resources, resulting in a prioritized list for the treatment of our most significant and at-risk resources. The first phase of cultural and paleontological resources inventory and prioritization will continue in 2016 and 2017. Treatment and excavation (data recovery) for the next phase is planned subsequent to completion of survey and prioritization efforts, beginning in 2018 (Figure 13).
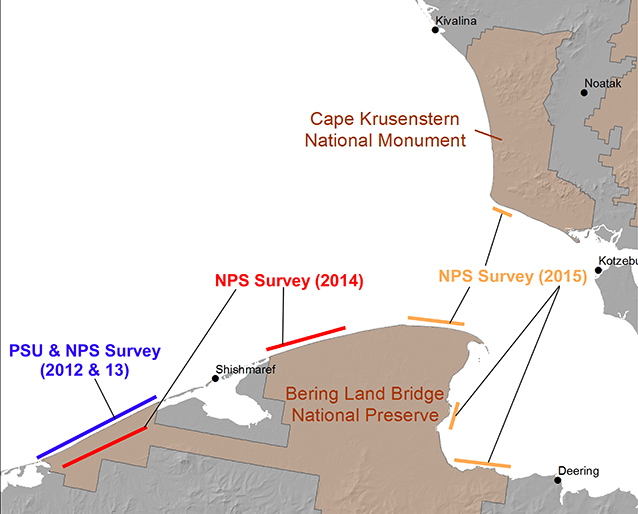
Concluding Remarks
Though Earth processes have continually shaped the region’s coastal landforms and ecosystems since the onset of the Holocene, current data suggests rising global temperatures and sea level are amplifying the effects of coastal landform erosion at unprecedented rates, leaving them more vulnerable than ever. Storm surges previously buffered by shore-fast ice and resilient permafrost are battering the coast at unparalleled intensity and frequency. Diminished annual snow cover, permafrost melt, and changing vegetation are exposing the region’s sand dunes to eolian erosion.
Understanding how permafrost responds to seasonal temperatures and monitoring significant changes at key locations such as Cape Krusenstern could aid in the future management of these resources. Our results at Cape Krusenstern have shown that the permafrost responds predictably to temperature increases and decreases from season to season. New permafrost monitoring stations were established in 2015 at the location of an eroding paleontological site at Imik Lagoon (Cape Krusenstern) and at an eroding archaeological site at Bering Land Bridge, to allow for future monitoring.
The irreversible impact of powerful Earth processes on cultural and paleontological resources is a key concern for NPS and our preservation partners. These fragile resources represent a moment frozen in time, giving us insight as to how our enigmatic predecessors adapted to past episodes of climatic amelioration. As bus-sized blocks of peat spill into the sea at unprecedented rates, paleontologists are rapidly losing evidence with which to study the movement and behaviors of the quintessential fauna roaming Beringia prior to global extinction at the end of our last great Ice Age. The most imminent concerns are those centering around the region’s coastal communities and uniquely adapted societies, as contemporary climate change related issues severely impact home and food security, and threaten independence and cultural identity. The NPS, with help from interdisciplinary research partners and traditional knowledge experts, are studying climate change related processes and how they threaten our most significant and at-risk cultural and paleontological resources. Finally, research resulting from this program is contributing substantially to our understanding of past and present human-environment dynamics in the region; a fascinating tale of survival, adaptation and the enduring human spirit.
References
Anderson, D. 1962.
Cape Krusenstern Ipiutak Economic and Settlement patterns. Providence: Brown University.
Arctic Climate Impact Assessment. 2004.
Impacts of a Warming Arctic: Arctic Climate Impacts Assessment. Cambridge University Press.
Anderson, D. 1988.
Onion Portage: The Archaeology of a Stratified Site from Kobuk River, Northwest Alaska. Anthropological Papers of the University of Alaska 22 (1-2).
Anderson, S. 2013.
Climate Change and Archaeology in Northwest Alaska: Nuluk Study. In partial fulfillment of cooperative agreement H8W07110001. Kotzebue: National
Park Service.
Anderson, S. 2015.
Climate Change and Archaeology in Northwest Alaska: Nuluk Study. Final Report. Portland and Anchorage: Portland State University and National Park Service.
Bigelow, N. 2003.
Climate change and Arctic ecosystems: Vegetation changes north of 55 degrees N between the last glacial maximum, mid-Holocene, and present. Journal of Geophysical Research-Atmospheres 108 (D19).
Bird, B. 2009.
A 2,000-year Varve-based Climate Record from the Central Brooks Range, Ala. Journal of Paleolimnology 41, 25-41.
Bowers, P. 2006.
Update on the Deering Archaeological Program. Anchorage: Northern Land Use Research, Inc.
Burch, E. 1998.
The Cultural and Natural Heritage of Northwest Alaska. Kotzebue, Alaska: NANA Museum of the Arctic.
Burch, E. 2006.
Social Life in Northwest Alaska: The Structure of Inupiaq Eskimo Nations. Fairbanks: University of
Alaska Press.
Calkin, P. 1998.
Glacier Regimes, Periglacial Landforms, and Holocene Climate Change in the Kigluaik Mountains, Seward Peninsula, Alaska, U.S.A. Arctic and Alpine Research 30(2), 154-165.
Carter, R., and D. Woodroffe. 1997.
Coastal Evolution: Late Quaternary Shoreline Morphodynamics. (R. W. Woodroffe, Ed.) University of Ulster.
Cronk, L. 1991.
Human Behavioral Ecology. Annual Review of Anthropology 20: 25-53.
Darwent, J. 2013.
1,000 Years of House Change at Cape Espenberg, Alaska: A Case Study in Horizontal Stratigraphy. American Antiquity 78 (3): 433-455.
Druckenmiller, M. 2011.
Alaska Shorefast Ice: Interfacing Geophysics with Local Sea Ice Knowledge and Use. A Thesis in Partial Fulfillment of Doctor of Philosophy. Fairbanks: University of Alaska.
Freeburg, A., and S. Anderson. 2012.
Human-Environmental Dynamics at Cape Krusenstern: 200 Generations: On the Beath of Their Time. Final Report for Task Agreement J8W07070032. Seattle: University of Washington.
Giddings, L. 1952.
Arctic Woodland Culture of the Kobuk River. Philadelphia, Pennsylvania: University of
Pennsylvania Museum.
Giddings, L. 1967.
Ancient Men of the Arctic. New York: Knopff.
Giddings, L., and D. Anderson. 1986.
Beach Ridge Archaeology of Cape Krusenstern: Eskimo and Pre-Eskimo Settlements Around Kotzebue Sound, Alaska. Washington, DC: National Park Service.
Guthrie, R. 2003.
Rapid body size decline in Alaskan Pleistocene horses before extinction. Nature 426, 169-171.
Guthrie, R. 2006.
New carbon dates link climatic change with human colonization and Pleistocene extinctions. Nature 441, 207-209.
Hardes, J. 2014.
Late Pleistocene Paleontology and Native Heritage in Northwest Alaska. Alaska Park Science 13 (1), 82-87.
Höfle, C. 2000.
The Full-Glacial Environment of the Northern Seward Peninsula, Alaska, Reconstructed from the 21,500-Year-Old Kitluk Paleosol. Quarternary Research 53, 143-453.
Hopkins, D. 1982.
Aspects of the Paleogeography of Beringia During the Late Pleistocene. In J. V. D. M. Hopkins (Ed.), Paleoecology of Beringia (pp. 3-28). New York: Academic Press.
Hu, F. 2001.
Pronounced climatic variations in Alaska during the last two millennia. Proceedings of the National Academy of Sciences 98: p. 105552.
Jacoby, G. 1999.
Laki Eruption of 1783, Tree Rings, and Disaster for Northwest Alaska Inuit. Quaternary Science Reviews 18, 1365-1371.
Jordan, J. and O. Mason. 1999.
A 5000-Year Record of Intertidal Peat Stratigraphy and Sea Level Change from Northwest Alaska. Quaternary International (60): 37-47.
Jordan, J. 1988.
Erosion Characteristics and Retreat Rates along the North Coast of Seward Peninsula. In J. Schaaf (Ed.), The Bering Land Bridge National Preserve: an Archaeological Survey (Vol. I, pp. 322-362). Anchorage: National Park Service.
Larsen, H., and F. Rainey. 1948.
Ipiutak and the Arctic Whale Hunting Culture.
New York: American Museum of Natural History.
Mahoney, A. 2014.
Land-fast ice project/experiments. Retrieved 2015 7-November from Forum for Arctic Modeling & Observational Synthesis: http://web.whoi.edu/famos/land-fast-ice/
Manley, W. 2002.
Postglacial Flooding of the Bering Land Bridge: A Geospatial Animation. Retrieved 2015 29-October from INSTAAR: http://instaar.colorado.edu/QGISL/bering_land_bridge/
Manley, W. 2007.
Coastal Change since 1950 in the Southeast Chukchi Sea, Alaska, Based on GIS and Field Measurements. Arctic Coastal Zones at Risk, Scientifica Workshop on the Impact of Global Climate Change on the Arctic Coastal Zones. Tromso, Norway.
Manley, W. 2010.
Digitized Shorelines for the Coastal Areas of Bering Land Bridge NP (BELA) and Cape Krusenstern NM (CAKR), Northwest Alaska. Arctic Network I&M Program. Fairbanks: National Park Service.
Mann, D. 2002.
Holocene History of the Great Kobuk Sand Dunes, Northwestern Alaska. Quaternary Science Reviews 21(4-6): 709.
Mason, O. 1995.
Late Holocene Storm and Sea-Level History in the Chukchi Sea. Journal of Coastal Research Special Issue No.17: 173-180.
NOAA. 2008.
The Mid-Holocene “Warm Period”. Retrieved 2015 29-October from National Oceanographic and Atomospheric Administration. Paleoclimatology: http://www.ncdc.noaa.gov/paleo/globalwarming/holocene.html
Overeem, J. 2011.
Sea Ice Loss Enhances Wave Action at the Arctic Coast. Geophysical Research Letters 38, 6pp.
Overland, J. and M. Wang. 2013.
When will the Summer Arctic be Nearly Sea Ice Free? Geophysical Research Letters 40: 2097–2101.
Ray, D. 1975.
The Eskimos of Bering Strait, 1650-1898. Seattle: University of Washington Press.
Sackur, S. 2013.
The Alaskan Village Set to Disappear Under Water in a Decade. Retrieved 2015 29-October from BBC News Magazine: http://www.bbc.com/news/magazine-23346370
Schaaf, J. 1988.
Bering Land Bridge National Preserve: An Archaeological Survey. National Park Service, Cultural Resources. Anchorage: National Park Service.
Stroeve, J. 2012.
Trends in Arctic Sea Ice Extent from CMIP5, CMIP3 and Observations. Geophysical Research Letters 39(L16502).
Tremayne, A. 2014.
Investigating the Arctic Small Tool Tradition at Bering Land Bridge National Preserve, Alaska. Anchorage: National Park Service.
Willis, D. 2004.
Sea Engulfing Alaskan Village. Retrieved 2015 29-October from BBC News Magazine: http://news.bbc.co.uk/2/hi/europe/3940399.stm
Wolff, C., and T. Urban. 2013.
Geophysical Analysis at the Old Whaling Site, Cape Krusenstern, Alaska: Reveals the Possible Impact of Permafrost Loss on Archaeological Interpretation. Polar Research 32.
Part of a series of articles titled Alaska Park Science - Volume 15 Issue 1: Coastal Research Science in Alaska's National Parks.
Last updated: November 17, 2016